The emerging role of chemokine receptor CXCR2 in cancer progression
Introduction
Cancer is amongst the leading causes of death worldwide, with the four most frequently occurring cancers being lung, breast, colorectal and prostate (1). Cancer arises through the sequential accumulation of mutations that develop due to damage to the genome (2). The process of carcinogenesis is extremely complex, though our understanding of cancer at the molecular level, including cellular signals, has rapidly progressed over the last two decades. This has led to the identification of novel targets for therapeutic intervention and the development of treatments to improve patient prognosis.
Chemokines and their receptors are increasingly recognised to be involved in the process of tumour development and metastasis. Chemokines are small, secreted peptides that are important in mediating leukocyte migration to sites of inflammation and secondary lymphoid organs, in addition to other physiological and pathological processes (3,4). They are primarily produced by immune cells, but can also be produced by non-immune cells such as vascular endothelial cells for functions such as angiogenesis (5). The CXC chemokine receptor 2 (CXCR2) binds several different chemokines to trigger its function. It is expressed on immune cells including neutrophils, mast cells, monocytes and macrophages (6). However, CXCR2 has also been found on endothelial and epithelial cells, and on numerous types of tumour cells (7,8). This receptor has been implicated in various disease states such as chronic inflammation, sepsis, lung pathology, atherosclerosis and neuroinflammation (8). Inflammation is one of the major hallmarks of cancers and is linked to 15–20% of cancer deaths worldwide (9). CXCR2 has been associated with poor outcomes for different cancers through its effects on migration, invasion and angiogenesis (10-16). The aim of this review is to provide an up to date analysis on the contribution of CXCR2 to the progression of different cancer types, and describe how pharmacological intervention against CXCR2 may be beneficial to patient outcome.
Chemokine superfamily classification
The chemokine superfamily consists of a large variety of ligands and their receptors; however not all ligand-receptor relationships are mutually exclusive. This superfamily is divided into four families related through structure and function—the largest family consists of CC chemokines, based on the four adjacent cysteine residues in their peptide sequence. The main function of CC chemokines is to attract mononuclear cells to sites of chronic inflammation. The second largest family consists of CXC chemokines, which have two cysteine residues and a single amino acid residue between them. Some members of this family, such as CXCL8, attract polymorphonuclear leukocytes to sites of inflammation. The third CX3C family consists of one member Fractalkine (CX3CL1), which has three amino acids separating the initial pair of cysteine residues. It is produced as a long protein with an extended mucin-like stalk fused to its chemokine domain, permitting binding to the surface of certain cells to form a cell-adhesion receptor. This cytokine can be cleaved to form a soluble chemoattractant (17,18). The fourth family also has a single member, lymphotactin (XCL1), which is similar to the members of the CC and CXC families but lacks two of the four characteristic cysteine residues found in most chemokines (19). There is a large amount of both redundancy and specificity within families in the chemokine system (20).
Chemokine receptor structure
Chemokine receptors are members of the seven transmembrane G-protein-coupled receptor (GPCR) family (Figure 1). They are differentially expressed on cells, with highly variable responses to specific chemokines. The receptors are generally composed of 350 amino acids in length, with a short acidic extracellular N-terminus domain that may contain sulfated tyrosine residues and sites for N-linked glycosylation. The intracellular C-terminus domain contains serine and threonine residues that regulate receptor activity through phosphorylation. The transmembrane domains are α-helical and the intracellular and extracellular loops contain hydrophilic amino acids. The first two loops have highly conserved cysteine residues linked by a disulfide bond (3). Each receptor is specific to ligands from only one of the four chemokine families, hence they are also placed into four groups. There are multiple receptor-ligand combinations within these groups resulting in a wide array of biological outcomes (21,22).
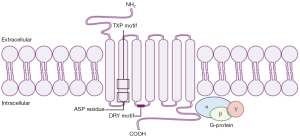
CXC chemokines have a heparin-binding domain in their C-terminus and are involved in regulating the process of angiogenesis (23). Aside from the CXC motif that distinguishes this family of chemokines from others, CXC chemokines have a second structural motif that determines its functional activity. The N-terminus of most CXC chemokines has three amino acid residues (Glu-Leu-Arg: the ELR motif) before the first cysteine amino acid residue in their primary structure (24,25). It has been found that some members that contain this ELR motif (ELR+) such as CXCL8 are not only potent angiogenic factors, but also neutrophil chemoattractants (26). On the other hand, CXC chemokines that lack the ELR motif (ELR−) are potent angiogenic inhibitors, such as CXCL4, CXCL9 and CXCL10 (24,27-29). CXCL12 is an exception to this, as it is an angiogenic ELR− CXC chemokine. ELR+ CXC chemokines bind to CXCR1 and CXCR2, and ELR− CXC chemokines bind to CXCR3–7 (Table 1).
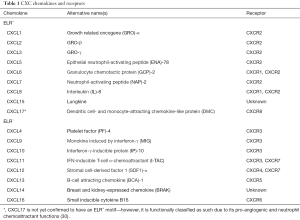
Full table
CXC chemokines and cancer
Aside from the massive contribution of CXC chemokines and their receptors to the immune system, they have also been implicated in the processes of tumour growth, angiogenesis and metastasis (Figure 2). Many tumour cells produce a large variety of chemokines and their receptors (31,32). Chemokines can indirectly function to recruit tumour-associated macrophages that support tumour development. They can also directly function as growth/survival factors, angiogenic factors and chemoattractants for cancer cells through autocrine effects that enhance tumour development and metastasis. ELR+ CXC chemokines in particular act as growth factors for tumour cells. Overexpression of CXCL1 or CXCL8 in melanoma cells has been shown to promote their growth and enhance metastatic capacity in vivo; inhibiting their receptor CXCR2 abolishes these effects (33-35). Overexpression of CXCR4 by breast cancer cells results in an enhanced response to its ligand CXCL12, promoting cell survival in vivo. This has been shown in primary and metastatic breast cancer (36,37). CXCL8 acts a direct autocrine growth factor in a number of different tumours including pancreatic, liver, ovarian, colon and melanoma (38-41). The receptors for CXCL8 are CXCR1 and CXCR2, which share 77% sequence homology, though they have different binding affinities and selectivity for chemokines; CXCR1 is highly selective for CXCL8 and has very low binding affinities to other ligands (42). The study of CXCR2 gene-deficient mice has shown that CXCR2 is activated by ELR+ CXC chemokines including CXCL1–3 and CXCL5–8 to mediate their angiogenic activity, though its most potent ligand is CXCL8 (43,44). Despite the difference in ligand binding affinities, CXCR1 and CXCR2 have both been shown to interact with the epidermal growth factor receptor (EGFR) signalling pathway, critical in regulating growth, survival, proliferation and differentiation in mammalian cells (39,45). CXCR2 in particular has been associated with the migration, invasion and angiogenesis of different cancers (10-16). The following sections describe the involvement of CXCR2 in the progression of four of the most common cancers worldwide, including lung, prostate, breast and colorectal, in addition to recent relevant data on pancreatic and ovarian cancer.
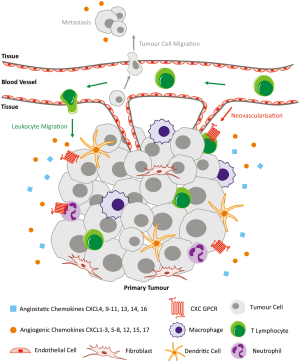
Lung cancer
Lung cancer is the leading cause of cancer deaths, with lung adenocarcinoma representing the most common histologic subtype of non-small cell lung cancer (NSCLC) (1,46). CXCR2 expression has been found to be upregulated in lung cancer in several studies (47-49). In one of these studies, CXCR2 expression was analyzed in tumour cells from over 260 human NSCLC and it was found that poorly differentiated tumours had significantly greater cytoplasmic CXCR2 levels compared to moderately and well-differentiated tumours. CXCR2 expression in lung adenocarcinomas was associated with smoking and poor prognosis, implicating the CXCR2 axis as a potential therapeutic target for in smoking-related lung adenocarcinoma (48). In a different study, inhibiting CXCR1 and CXCR2 using a mutant CXCL8 analogue (G31P) in two lung cancer cell lines showed decreased cell proliferation and migration, and enhanced apoptosis. Similar inhibition in an in vivo orthotopic xenograft mouse model of human lung cancer showed suppressed tumour growth, metastasis and angiogenesis (47).
Airway inflammation has been associated with the progression of lung cancer, particularly in cigarette smokers (50-52). Increased neutrophil infiltration as a result of the immune response is linked to poorer outcome in patients with lung cancer (53). Tazzyman et al. found that neutrophil recruitment into A549 tumours in vitro and in vivo is largely dependent on CXCR2 activation (54). Supporting these findings, a more recent study by Gong et al. showed that CXCR2 inhibition in a K-RAS mutant mouse model of lung cancer suppressed neutrophilic inflammation and significantly reduced tumour progression (55).
In a preclinical mouse model of lung cancer, ELR+ chemokines were found to mediate angiogenic activity via CXCR2 and depletion of CXCR2 inhibited tumour growth and angiogenesis (56). On the other hand, the previously mentioned study by Tazzyman et al. demonstrated no reduction in microvascular density in CXCR2-deficient mice, leading to their conclusion that angiogenesis was CXCR2-independent (54). It is important to note that in the first study, Lewis lung cancer cells were injected into CXCR2-deficient C57BL/6 mice; in the second study A549 lung cancer cells were injected into CXCR2-deficient SCID mice. Therefore the differences seen in the results of these studies may be attributable to the different murine models or cancer cell lines used. It is also possible that perhaps an intact immune system is required for CXCR2-dependent angiogenesis.
In contrast to the findings that CXCR2 is associated with tumourigenesis, a recent study by Ryan et al. identified a functional single nucleotide polymorphism (SNP) in the 3' untranslated region (UTR) of CXCR2 that alters binding of a specific miRNA (miR-516a-3p), affecting CXCR2 mRNA and protein expression (57). Surprisingly, this SNP gives rise to increased CXCR2 expression but is associated with reduced risk of lung cancer. Initial studies by Ryan et al. of primary samples showed the reduction of CXCR2 in lung cancer compared to non-involved lung tissue. They carried out further studies using RNA-seq data from TCGA on lung adenocarcinoma and squamous cell carcinoma, which also showed decreased expression of all three CXCR2 mRNA isoforms. It is known that CXCR2 may mediate p53-dependent senescence in the lung, highlighting the potential tumour-suppressive role of CXCR2 (58). A mutation found in the C-terminus of CXCR2 (G354W), identified in the NCI-H1395 lung cancer cell line, prevents autocrine CXCL8-induced receptor internalization and alleviates senescence (58,59). Reduced CXCR2 expression has been suggested to be another mechanism of avoiding senescence in lung cancer.
Prostate cancer
Prostate cancer is the most frequently diagnosed cancer in males, and the fifth leading cause of cancer-related deaths in men (1). Hypoxia is one of the key features of solid tumours as found in prostate cancer, leading to the selection of cells with a more malignant phenotype (60). Elevated CXCL8, CXCR1 and CXCR2 expression has been detected in human prostate cancer (61). Hypoxia was found to further increase expression of CXCL8 and its receptors in a time-dependent manner, and inhibition of transcription factors hypoxia-inducible factor (HIF-1) and nuclear factor-κB (NF-κB) abrogated these effects (62). In another study, the effects of CXCL8 mutant analogue G31P were investigated on the proliferation, migration and invasion of androgen-independent prostate cancer cells in vitro and on growth and angiogenesis in nude mouse xenografts of prostate cancer. They found that G31P treatment suppressed tumourigenesis both in vitro and in vivo. G31P also inhibited tumour tissue vascularization, which was associated with decreased expression of vascular endothelial growth factor (VEGF) and NF-κB expression in the xenograft tissues (63). In CXCR2-deficient mice, Shen et al. also found smaller tumour size and reduced angiogenesis compared to normal mice (64). These findings are similar to those described in ovarian cancer by Yang et al. whereby CXCR2 inhibition in nude mice xenografts resulted in decreased blood vessel density and increased VEGF expression (10). This combined data, amongst others, provides evidence for a clear association between CXCR2 and angiogenesis, a mechanism that may be manifested across different cancers.
Breast cancer
Breast cancer is the second most common cancer in the world and the most frequent cause of cancer death amongst women (1,65). Polymorphisms in CXCL8 and CXCR2 genes are associated with increased breast cancer risk (66). CXCR2 expression has been shown to be higher in malignant breast cancer tissues compared to benign ductal epithelial samples (67). CXCR2 has further been found to promote breast cancer cell invasion in vitro and enhance tumour metastasis in vivo (68). Bone metastasis is common during breast cancer progression, and CXCR2 has been implicated in this process as well (69). In addition, infiltrating macrophages in the tumour microenvironment were found to have increased expression of CXCR2 ligands, inhibited by activation of the TGF-β signalling pathway. Co-culture studies showed that these macrophages can promote both epithelial and tumour cell migration via CXCR2 activation (70). However, the phenomenon of oncogene-induced senescence through CXCR2 signalling has also been observed in breast cancer cells, as described in pancreatic and lung cancers in this review (71).
Colorectal cancer
Colorectal cancer is one of the most common causes of cancer mortality and is often associated with the Western lifestyle (72). Increased expression of CXC chemokines and their receptors in colorectal cancer cells correlates with poor prognosis (73). A higher constitutive expression of CXCL8, CXCR1 and CXCR2 has been associated with greater metastatic potential of colorectal cancer cells (74,75). A recent study comparing CXCR2 expression in colorectal tumour tissues to benign tumour and normal mucosa tissues found a significantly higher expression in the tumour tissues. CXCR2 expression was also observed to be higher in patients either with lymph node metastasis or in advanced cancer stages (76). This data adds to the accumulating evidence that CXCR2 enhances cancer progression and could worsen patient outcome.
Pancreatic ductal adenocarcinoma (PDAC)
PDAC is a leading cause of cancer deaths worldwide with minimal treatment options available (77). Due to multiple studies implicating CXCR2 in cancer and inflammation, research has been carried out recently on the involvement of CXCR2 and its ligands in pancreatic cancer. CXCR2 is expressed in various PDAC cell lines and has been found to increase their proliferation and survival via autocrine and paracrine effects (38,78-80). Immunohistochemical analysis showed positive staining for CXCL8 (50%), CXCR1 (55%) and CXCR2 (65%) in surgically resected human pancreatic cancer, with 40% being positive for both CXCL8 and its receptors (80). ELR+ CXC chemokines mediate their angiogenic activity through CXCR2 and have been found at increased levels in both pancreatic cancer cell lines and pancreatic secretions of pancreatic cancer patients (81-83). It has been suggested that the role of CXCR2 and its chemokines as angiogenic factors for pancreatic cancer may be dependent on the tumour microenvironment and tumour-stromal interactions (11,84). The expression of ELR+ CXC chemokines increases when pancreatic cancer cells are co-cultured with stromal cells (11). In addition, Ijichi et al. found higher CXCR2 expression in fibroblasts from PDAC stroma, implying that PDAC expression of CXCR2 ligands could be important in the formation and maintenance of the stroma. Using a murine model of aggressive PDAC disease similar to the human disease, they found that PDAC cells secreted higher levels of CXCL1, 2, 5 and 16 compared to preinvasive mouse pancreatic intraepithelial neoplasia cells. These chemokines stimulated stromal fibroblasts to induce connective tissue growth factor (CTGF), a pro-tumourigenic factor, resulting in accelerated tumour growth in vivo. Inhibition of CXCR2 improved overall survival of the mice by suppressing tumour angiogenesis (85). These studies highlight the importance of the CXCL-CXCR2 axis in tumour-stromal interactions in the progression of PDAC. However, there have been other recent studies that have reported targeting of the stroma may be detrimental to the patient by accelerating disease progression (86,87). This emphasizes the caution that must be undertaken when targeting PDAC-associated fibroblasts.
Another function of CXCR2 is regulating the migration of myeloid-derived suppressor cells (MDSCs) (88). Increasing evidence suggests that neutrophils, MDSCs and CXCR2 play a role in pancreatic cancer. Neutrophil infiltration in pancreatic cancer is associated with poorer prognosis, and the accumulation of MDSCs in patients with advanced pancreatic cancer also correlates with disease stage (89,90). Recent studies by Steele et al. showed the upregulation of CXCR2 signalling in human pancreatic cancer, predominantly in neutrophils and MDSCs. Inhibiting CXCR2 reduced metastasis and improved the overall survival of mice with established disease. Interestingly, it is reported that only peptide inhibition, not germline deletion of CXCR2, improved survival (91). This suggests that CXCR2 may have multiple roles in the initiation and progression of pancreatic cancer, a phenomenon that has been previously described (59,84).
Further study into the molecular mechanisms of CXCR2 signalling in pancreatic cancer cells both in vitro and in vivo showed that CXCR2 is coupled to downstream effector phospholipase C-β3 (PLC-β3), mediated by a specific motif at its C-terminus called PSD-95/DlgA/ZO-1 (PDZ). This motif enables interactions with PDZ scaffold protein Na+/H+ exchange regulatory factor 1 (NHERF1) to create a macromolecular signalling complex (92). This interaction is thought to be important for the regulation of intracellular signalling and cell functions in neutrophils (93,94). The disruption of this complex was found to significantly inhibit pancreatic cancer cell proliferation and invasion (92). Recent advances in research on the involvement of CXCR2 in pancreatic cancer demonstrate that CXCR2 provides an important contribution to the progression of PDAC through multiple avenues.
Ovarian cancer
Ovarian cancer is the most lethal gynaecological cancer and the fifth leading cause of cancer-related deaths in women in the United Kingdom (95). CXCR2 overexpression has been found in high-grade serous ovarian carcinomas to be an independent prognostic factor of poor overall survival and early relapse (10). A study by Yang et al. found that ovarian cancer cells frequently express CXCR2, which promotes growth by enhancing angiogenesis, reducing apoptosis and dysregulating the cell cycle. The study then went further to investigate the mechanisms behind CXCR2-mediated ovarian cancer growth. They found that CXCR2 promoted cell cycle progression by affecting a host of cell cycle regulatory proteins including cyclin D1 and its cyclin-dependent kinase (CDK)-6. Silencing CXCR2 expression increased cell apoptosis at least 2-fold, possibly due to increased p53 phosphorylation, increased expression of Puma and Bcl-xS (pro-apoptotic proteins) and reduced expression of Bcl-xL and Bcl-2 (anti-apoptotic proteins). CXCR2 inhibition in xenograft mouse tumour tissues generated from animals with ovarian cancer resulted in decreased overall blood vessel density, increased expression of VEGF and decreased expression of thrombospondin-1 (TSP-1), implicating CXCR2 in ovarian cancer angiogenesis. Yang et al. then identified the involvement of multiple signalling pathways in these processes, including the mitogen-activated protein kinase (MAPK), phosphoinositide 3-kinase (PI3K), signal transducer and activator of transcription 3 (STAT3) and NF-κB pathways (10). Several studies have previously shown that activation of these pathways may be involved in the CXCR2 signalling network in other cell types (96-99).
A recent study by Dong et al. explored in further detail the impact of NF-κB signalling on the contribution of CXCR2 to the progression of ovarian cancer (100). Ovarian cancer consists of a proinflammatory tumour microenvironment that may be in part regulated by tumour necrosis factor (TNF) (101,102). TNF has previously been shown to regulate chemokine networks though the NF-κB signalling pathway in ovarian cancer (103,104). CXCR2-driven ovarian cancer progression was found to upregulate its own ligands including CXCL1 and CXCL2. Both basal and TNF-induced levels of NF-κB activation were found to be higher in CXCR2 expressing cells. These cells had increased proliferation, migration and invasion compared to CXCR2-deficient cells. Knocking down CXCR2 resulted in decreased TNF-induced NF-κB activation (100). This is consistent with previous findings whereby in colorectal cancer cells, a CXCR2 antagonist decreased NF-κB phosphorylation and in ovarian cancer cells, the stimulation of angiogenesis by CXCR2 was suggested to involve NF-κB (10,105). Dong et al. also showed that CXCR2 expressing cells had greater transactivation of EGFR, causing higher Akt activation. Inhibiting PI3K blocked Akt activation and attenuated NF-κB promoter activity (100). Interestingly, several CXCR2 ligands, including CXCL1–3 and CXCL5–8 contain NF-κB binding sites in their promoters (6). Therefore, it has been suggested that CXCR2-mediated NF-κB activation can modulate the chemokine network thereby altering cellular function (100).
Therapeutic benefits of CXCR2 functional intervention
The importance of CXCR2 has been highly emphasized in tumourigenesis. ELR+ CXC chemokines are known to mediate their angiogenic effects through the CXCR2 receptor, and indeed its inhibition has shown several anti-tumourigenic effects as previously described. This provides the opportunity to intervene in key processes in tumour development, including growth, invasion, angiogenesis and metastasis. Interestingly, previous studies have shown that many cellular signals including CXCR2 and HIF-1α are upregulated by volatile anaesthetic exposure to cancer cell lines, although the implications of these findings remain elusive (106-109).
CXCR2 has also been identified to play a role in the chemoresistance of numerous cancers including prostate, breast and colorectal. CXCL8 signalling has been shown to confer metastatic prostate cancer cells with resistance to specific chemotherapeutic agents. Administration of 5-fluorouracil (5-FU) to prostate cancer cells (PC3) caused the increase of CXCL8 secretion and upregulation of CXCR1 and CXCR2 gene expression. Inhibition of CXCL8 signalling in prostate cancer cells using the CXCR2 inhibitor, AZ10397767, was found to increase the cytotoxicity of 5-FU, a key anti-metabolite in solid-tumour chemotherapy (110). In breast cancer, malignant cells surviving initial chemo- and radiation therapy were found to have higher expression of CXCR2 ligands. Knocking down CXCR2 increased toxicity of chemotherapy agents paclitaxel and doxorubicin. It also enhanced paclitaxel antitumour activity in an in vivo breast cancer model. Spontaneous lung metastases in animals with CXCR2 knockdown and treated with paclitaxel were significantly reduced compared to control animals (111). Furthermore, a study investigating the involvement of CXCL8 and CXCR2 in a 5-FU chemoresistant colorectal cell line (HCT116/5-FU) found that both were upregulated in comparison to their chemosensitive parental cell line (HCT116). Inhibiting CXCR2 caused reduced cell proliferation in both cell lines. In response to 5-FU treatment, HCT116 upregulated CXCL8, CXCR1 and CXCR2, but HCT116/5-FU only upregulated CXCL8 and CXCR1. On the other hand, treatment with another chemotherapy agent oxaliplatin resulted in no significant differences in either cell line (112). Ning et al., however, reported that CXCL8 overexpression by CXCL8-transfected clones of HCT116 and an additional cell line CaCo2 conferred significant resistance to the chemotherapeutic drug oxaliplatin; inhibiting CXCL8 overexpression reversed this observed chemoresistance. The transfected cell lines also had a greater expression of CXCR2 compared to their normal counterparts (113). These examples highlight the importance of CXCR2 in chemotherapy resistance and provide evidence that targeting CXCR2 signalling can enhance the antitumour and antimetastatic activity of chemotherapeutic drugs.
Conclusions
The role of CXCR2 has been investigated in different cancer types, and there is a plethora of evidence that shows the profound effect that CXCR2 has on tumour progression. Deeper research into the molecular mechanisms of CXCR2 activity has shown its effects on numerous key signalling pathways, including MAPK, NF-κB, Akt and STAT3. Through these pathways, CXCR2 is able to modulate tumour growth, angiogenesis and metastasis, potentiating tumour development. There is a potential tumour suppressive role for CXCR2 that has also been highlighted, possibly depending on the stage of the tumour. Although CXCR2 could be an excellent molecular target for the treatment of cancer, due to the complexity of carcinogenesis and the tumour microenvironment, it is unlikely that a single CXCR2 inhibitor would be curative. Perhaps the use of multiple therapeutic regimens would be more beneficial in the control of tumour development and metastasis.
Acknowledgments
Funding: This research was supported by the BOC Chair Grant, Royal College of Anaesthetists, London, UK.
Footnote
Provenance and Peer Review: This article was commissioned and reviewed by the Section Editor Qian Chen (Center for Inflammation & Epigenetics, Houston Methodist Hospital Research Institute, Houston, TX, USA).
Conflicts of Interest: Both authors have completed the ICMJE uniform disclosure form (available at http://dx.doi.org/10.21037/tcr.2016.10.06). The authors have no conflicts of interest to declare.
Ethical Statement: The authors are accountable for all aspects of the work in ensuring that questions related to the accuracy or integrity of any part of the work are appropriately investigated and resolved.
Open Access Statement: This is an Open Access article distributed in accordance with the Creative Commons Attribution-NonCommercial-NoDerivs 4.0 International License (CC BY-NC-ND 4.0), which permits the non-commercial replication and distribution of the article with the strict proviso that no changes or edits are made and the original work is properly cited (including links to both the formal publication through the relevant DOI and the license). See: https://creativecommons.org/licenses/by-nc-nd/4.0/.
References
- Ferlay J, Soerjomataram I, Ervik M, et al. GLOBOCAN 2012 v1.0, Cancer Incidence and Mortality Worldwide: IARC CancerBase No. 11. Lyon, France: International Agency for Research on Cancer; 2013. Available online: http://globocan.iarc.fr. Accessed on 6 August 2016.
- Bertram JS. The molecular biology of cancer. Mol Aspects Med 2000;21:167-223. [Crossref] [PubMed]
- Murdoch C, Finn A. Chemokine receptors and their role in inflammation and infectious diseases. Blood 2000;95:3032-43. [PubMed]
- Rossi D, Zlotnik A. The biology of chemokines and their receptors. Annu Rev Immunol 2000;18:217-42. [Crossref] [PubMed]
- Proudfoot AE. Chemokine receptors: multifaceted therapeutic targets. Nat Rev Immunol 2002;2:106-15. [Crossref] [PubMed]
- Olson TS, Ley K. Chemokines and chemokine receptors in leukocyte trafficking. Am J Physiol Regul Integr Comp Physiol 2002;283:R7-28. [Crossref] [PubMed]
- Strieter RM, Burdick MD, Mestas J, et al. Cancer CXC chemokine networks and tumour angiogenesis. Eur J Cancer 2006;42:768-78. [Crossref] [PubMed]
- Veenstra M, Ransohoff RM. Chemokine receptor CXCR2: physiology regulator and neuroinflammation controller? J Neuroimmunol 2012;246:1-9. [Crossref] [PubMed]
- Balkwill F, Mantovani A. Inflammation and cancer: back to Virchow? Lancet 2001;357:539-45. [Crossref] [PubMed]
- Yang G, Rosen DG, Liu G, et al. CXCR2 promotes ovarian cancer growth through dysregulated cell cycle, diminished apoptosis, and enhanced angiogenesis. Clin Cancer Res 2010;16:3875-86. [Crossref] [PubMed]
- Matsuo Y, Ochi N, Sawai H, et al. CXCL8/IL-8 and CXCL12/SDF-1alpha co-operatively promote invasiveness and angiogenesis in pancreatic cancer. Int J Cancer 2009;124:853-61. [Crossref] [PubMed]
- Gabellini C, Trisciuoglio D, Desideri M, et al. Functional activity of CXCL8 receptors, CXCR1 and CXCR2, on human malignant melanoma progression. Eur J Cancer 2009;45:2618-27. [Crossref] [PubMed]
- Singh S, Singh AP, Sharma B, et al. CXCL8 and its cognate receptors in melanoma progression and metastasis. Future Oncol 2010;6:111-6. [Crossref] [PubMed]
- Reiland J, Furcht LT, McCarthy JB. CXC-chemokines stimulate invasion and chemotaxis in prostate carcinoma cells through the CXCR2 receptor. Prostate 1999;41:78-88. [Crossref] [PubMed]
- Liu Z, Yang L, Xu J, et al. Enhanced expression and clinical significance of chemokine receptor CXCR2 in hepatocellular carcinoma. J Surg Res 2011;166:241-6. [Crossref] [PubMed]
- Han L, Jiang B, Wu H, et al. High expression of CXCR2 is associated with tumorigenesis, progression, and prognosis of laryngeal squamous cell carcinoma. Med Oncol 2012;29:2466-72. [Crossref] [PubMed]
- Charo IF, Ransohoff RM. The Many Roles of Chemokines and Chemokine Receptors in Inflammation. N Engl J Med 2006;354:610-21. [Crossref] [PubMed]
- Bazan JF, Bacon KB, Hardiman G, et al. A new class of membrane-bound chemokine with a CX3C motif. Nature 1997;385:640-4. [Crossref] [PubMed]
- Kelner GS, Kennedy J, Bacon KB, et al. Lymphotactin: a cytokine that represents a new class of chemokine. Science 1994;266:1395-9. [Crossref] [PubMed]
- Mantovani A. The chemokine system: redundancy for robust outputs. Immunol Today 1999;20:254-7. [Crossref] [PubMed]
- Zhu Q, Han X, Peng J, et al. The role of CXC chemokines and their receptors in the progression and treatment of tumors. J Mol Histol 2012;43:699-713. [Crossref] [PubMed]
- Vicari AP, Caux C. Chemokines in cancer. Cytokine Growth Factor Rev 2002;13:143-54. [Crossref] [PubMed]
- Belperio JA, Keane MP, Arenberg DA, et al. CXC chemokines in angiogenesis. J Leukoc Biol 2000;68:1-8. [PubMed]
- Strieter RM, Polverini PJ, Kunkel SL, et al. The functional role of the ELR motif in CXC chemokine-mediated angiogenesis. J Biol Chem 1995;270:27348-57. [Crossref] [PubMed]
- Strieter RM, Polverini PJ, Arenberg DA, et al. The role of CXC chemokines as regulators of angiogenesis. Shock 1995;4:155-60. [Crossref] [PubMed]
- Keeley EC, Mehrad B, Strieter RM. Chemokines as Mediators of Neovascularization. Arterioscler Thromb Vasc Biol 2008;28:1928-36. [Crossref] [PubMed]
- Luster AD, Greenberg SM, Leder P. The IP-10 chemokine binds to a specific cell surface heparan sulfate site shared with platelet factor 4 and inhibits endothelial cell proliferation. J Exp Med 1995;182:219-31. [Crossref] [PubMed]
- Sgadari C, Farber JM, Angiolillo AL, et al. Mig, the monokine induced by interferon-gamma, promotes tumor necrosis in vivo. Blood 1997;89:2635-43. [PubMed]
- Angiolillo AL, Sgadari C, Taub DD, et al. Human interferon-inducible protein 10 is a potent inhibitor of angiogenesis in vivo. J Exp Med 1995;182:155-62. [Crossref] [PubMed]
- Maravillas-Montero JL, Burkhardt AM, Hevezi PA, et al. Cutting edge: GPR35/CXCR8 is the receptor of the mucosal chemokine CXCL17. J Immunol 2015;194:29-33. [Crossref] [PubMed]
- Balkwill F. Cancer and the chemokine network. Nat Rev Cancer 2004;4:540-50. [Crossref] [PubMed]
- Homey B, Muller A, Zlotnik A. Chemokines: agents for the immunotherapy of cancer? Nat Rev Immunol 2002;2:175-84. [Crossref] [PubMed]
- Balentien E, Mufson BE, Shattuck RL, et al. Effects of MGSA/GRO alpha on melanocyte transformation. Oncogene 1991;6:1115-24. [PubMed]
- Singh RK, Gutman M, Radinsky R, et al. Expression of interleukin 8 correlates with the metastatic potential of human melanoma cells in nude mice. Cancer Res 1994;54:3242-7. [PubMed]
- Norgauer J, Metzner B, Schraufstatter I. Expression and growth-promoting function of the IL-8 receptor beta in human melanoma cells. J Immunol 1996;156:1132-7. [PubMed]
- Smith MC, Luker KE, Garbow JR, et al. CXCR4 Regulates Growth of Both Primary and Metastatic Breast Cancer. Cancer Res 2004;64:8604-12. [Crossref] [PubMed]
- Luker KE, Luker GD. Functions of CXCL12 and CXCR4 in breast cancer. Cancer Lett 2006;238:30-41. [Crossref] [PubMed]
- Miyamoto M, Shimizu Y, Okada K, et al. Effect of interleukin-8 on production of tumor-associated substances and autocrine growth of human liver and pancreatic cancer cells. Cancer Immunol Immunother 1998;47:47-57. [Crossref] [PubMed]
- Venkatakrishnan G, Salgia R, Groopman JE. Chemokine receptors CXCR-1/2 activate mitogen-activated protein kinase via the epidermal growth factor receptor in ovarian cancer cells. J Biol Chem 2000;275:6868-75. [Crossref] [PubMed]
- Brew R, Erikson JS, West DC, et al. Interleukin-8 as an autocrine growth factor for human colon carcinoma cells in vitro. Cytokine 2000;12:78-85. [Crossref] [PubMed]
- Schadendorf D, Moller A, Algermissen B, et al. IL-8 produced by human malignant melanoma cells in vitro is an essential autocrine growth factor. J Immunol 1993;151:2667-75. [PubMed]
- Lee J, Horuk R, Rice GC, et al. Characterization of two high affinity human interleukin-8 receptors. J Biol Chem 1992;267:16283-7. [PubMed]
- Stadtmann A, Zarbock A. CXCR2: From Bench to Bedside. Front Immunol 2012;3:263. [Crossref] [PubMed]
- Addison CL, Daniel TO, Burdick MD, et al. The CXC Chemokine Receptor 2, CXCR2, Is the Putative Receptor for ELR+ CXC Chemokine-Induced Angiogenic Activity. J Immunol 2000;165:5269-77. [Crossref] [PubMed]
- Oda K, Matsuoka Y, Funahashi A, et al. A comprehensive pathway map of epidermal growth factor receptor signaling. Mol Syst Biol 2005;1:2005.0010.
- Travis WD, Brambilla E, Noguchi M, et al. International association for the study of lung cancer/american thoracic society/european respiratory society international multidisciplinary classification of lung adenocarcinoma. J Thorac Oncol 2011;6:244-85. [Crossref] [PubMed]
- Khan MN, Wang B, Wei J, et al. CXCR1/2 antagonism with CXCL8/Interleukin-8 analogue CXCL8(3-72)K11R/G31P restricts lung cancer growth by inhibiting tumor cell proliferation and suppressing angiogenesis. Oncotarget 2015;6:21315-27. [Crossref] [PubMed]
- Saintigny P, Massarelli E, Lin S, et al. CXCR2 expression in tumor cells is a poor prognostic factor and promotes invasion and metastasis in lung adenocarcinoma. Cancer Res 2013;73:571-82. [Crossref] [PubMed]
- Ohri CM, Shikotra A, Green RH, et al. Chemokine receptor expression in tumour islets and stroma in non-small cell lung cancer. BMC Cancer 2010;10:172. [Crossref] [PubMed]
- Spitz MR, Gorlov IP, Dong Q, et al. Multistage analysis of variants in the inflammation pathway and lung cancer risk in smokers. Cancer Epidemiol Biomarkers Prev 2012;21:1213-21. [Crossref] [PubMed]
- Shacter E, Weitzman SA. Chronic inflammation and cancer. Oncology 2002;16:217-26. [PubMed]
- Celli BR. Chronic obstructive pulmonary disease and lung cancer: common pathogenesis, shared clinical challenges. Proc Am Thorac Soc 2012;9:74-9. [Crossref] [PubMed]
- Bellocq A, Antoine M, Flahault A, et al. Neutrophil alveolitis in bronchioloalveolar carcinoma: induction by tumor-derived interleukin-8 and relation to clinical outcome. Am J Pathol 1998;152:83-92. [PubMed]
- Tazzyman S, Barry ST, Ashton S, et al. Inhibition of neutrophil infiltration into A549 lung tumors in vitro and in vivo using a CXCR2-specific antagonist is associated with reduced tumor growth. Int J Cancer 2011;129:847-58. [Crossref] [PubMed]
- Gong L, Cumpian AM, Caetano MS, et al. Promoting effect of neutrophils on lung tumorigenesis is mediated by CXCR2 and neutrophil elastase. Mol Cancer 2013;12:154. [Crossref] [PubMed]
- Keane MP, Belperio JA, Xue YY, et al. Depletion of CXCR2 inhibits tumor growth and angiogenesis in a murine model of lung cancer. J Immunol 2004;172:2853-60. [Crossref] [PubMed]
- Ryan BM, Robles AI, McClary AC, et al. Identification of a functional SNP in the 3'UTR of CXCR2 that is associated with reduced risk of lung cancer. Cancer Res 2015;75:566-75. [Crossref] [PubMed]
- Acosta JC, Gil J. A role for CXCR2 in senescence, but what about in cancer? Cancer Res 2009;69:2167-70. [Crossref] [PubMed]
- Acosta JC, O'Loghlen A, Banito A, et al. Chemokine signaling via the CXCR2 receptor reinforces senescence. Cell 2008;133:1006-18. [Crossref] [PubMed]
- Movsas B, Chapman JD, Horwitz EM, et al. Hypoxic regions exist in human prostate carcinoma. Urology 1999;53:11-8. [Crossref] [PubMed]
- Murphy C, McGurk M, Pettigrew J, et al. Nonapical and cytoplasmic expression of interleukin-8, CXCR1, and CXCR2 correlates with cell proliferation and microvessel density in prostate cancer. Clin Cancer Res 2005;11:4117-27. [Crossref] [PubMed]
- Maxwell PJ, Gallagher R, Seaton A, et al. HIF-1 and NF-kappaB-mediated upregulation of CXCR1 and CXCR2 expression promotes cell survival in hypoxic prostate cancer cells. Oncogene 2007;26:7333-45. [Crossref] [PubMed]
- Liu X, Peng J, Sun W, et al. G31P, an antagonist against CXC chemokine receptors 1 and 2, inhibits growth of human prostate cancer cells in nude mice. Tohoku J Exp Med 2012;228:147-56. [Crossref] [PubMed]
- Shen H, Schuster R, Lu B, et al. Critical and opposing roles of the chemokine receptors CXCR2 and CXCR3 in prostate tumor growth. Prostate 2006;66:1721-8. [Crossref] [PubMed]
- Kamali-Sarvestani E, Aliparasti MR, Atefi S. Association of interleukin-8 (IL-8 or CXCL8) -251T/A and CXCR2 +1208C/T gene polymorphisms with breast cancer. Neoplasma 2007;54:484-9. [PubMed]
- Snoussi K, Mahfoudh W, Bouaouina N, et al. Combined effects of IL-8 and CXCR2 gene polymorphisms on breast cancer susceptibility and aggressiveness. BMC Cancer 2010;10:283. [Crossref] [PubMed]
- Miller LJ, Kurtzman SH, Wang Y, et al. Expression of interleukin-8 receptors on tumor cells and vascular endothelial cells in human breast cancer tissue. Anticancer Res 1998;18:77-81. [PubMed]
- Nannuru KC, Sharma B, Varney ML, et al. Role of chemokine receptor CXCR2 expression in mammary tumor growth, angiogenesis and metastasis. J Carcinog 2011;10:40. [Crossref] [PubMed]
- Halpern JL, Kilbarger A, Lynch CC. Mesenchymal stem cells promote mammary cancer cell migration in vitro via the CXCR2 receptor. Cancer Lett 2011;308:91-9. [Crossref] [PubMed]
- Bohrer LR, Schwertfeger KL. Macrophages promote fibroblast growth factor receptor-driven tumor cell migration and invasion in a CXCR2-dependent manner. Mol Cancer Res 2012;10:1294-305. [Crossref] [PubMed]
- Ruan JW, Liao YC, Lua I, et al. Human pituitary tumor-transforming gene 1 overexpression reinforces oncogene-induced senescence through CXCR2/p21 signaling in breast cancer cells. Breast Cancer Res 2012;14:R106. [Crossref] [PubMed]
- Herszényi L, Tulassay Z. Epidemiology of gastrointestinal and liver tumors. Eur Rev Med Pharmacol Sci 2010;14:249-58. [PubMed]
- Verbeke H, Struyf S, Laureys G, et al. The expression and role of CXC chemokines in colorectal cancer. Cytokine Growth Factor Rev 2011;22:345-58. [Crossref] [PubMed]
- Li A, Varney ML, Singh RK. Constitutive expression of growth regulated oncogene (gro) in human colon carcinoma cells with different metastatic potential and its role in regulating their metastatic phenotype. Clin Exp Metastasis 2004;21:571-9. [Crossref] [PubMed]
- Li A, Varney ML, Singh RK. Expression of interleukin 8 and its receptors in human colon carcinoma cells with different metastatic potentials. Clin Cancer Res 2001;7:3298-304. [PubMed]
- Wu W, Sun C, Xu D, et al. Expression of CXCR2 and its clinical significance in human colorectal cancer. Int J Clin Exp Med 2015;8:5883-9. [PubMed]
- Siegel RL, Miller KD, Jemal A. Cancer statistics, 2016. CA Cancer J Clin 2016;66:7-30. [Crossref] [PubMed]
- Takamori H, Oades ZG, Hoch OC, et al. Autocrine growth effect of IL-8 and GROalpha on a human pancreatic cancer cell line, Capan-1. Pancreas 2000;21:52-6. [Crossref] [PubMed]
- Hidaka H, Ishiko T, Furuhashi T, et al. Curcumin inhibits interleukin 8 production and enhances interleukin 8 receptor expression on the cell surface:impact on human pancreatic carcinoma cell growth by autocrine regulation. Cancer 2002;95:1206-14. [Crossref] [PubMed]
- Kuwada Y, Sasaki T, Morinaka K, et al. Potential involvement of IL-8 and its receptors in the invasiveness of pancreatic cancer cells. Int J Oncol 2003;22:765-71. [PubMed]
- Wente MN, Keane MP, Burdick MD, et al. Blockade of the chemokine receptor CXCR2 inhibits pancreatic cancer cell-induced angiogenesis. Cancer Lett 2006;241:221-7. [Crossref] [PubMed]
- Matsuo Y, Raimondo M, Woodward TA, et al. CXC-chemokine/CXCR2 biological axis promotes angiogenesis in vitro and in vivo in pancreatic cancer. Int J Cancer 2009;125:1027-37. [Crossref] [PubMed]
- Frick VO, Rubie C, Wagner M, et al. Enhanced ENA-78 and IL-8 expression in patients with malignant pancreatic diseases. Pancreatology 2008;8:488-97. [Crossref] [PubMed]
- Hertzer KM, Donald GW, Hines OJ. CXCR2: a target for pancreatic cancer treatment? Expert Opin Ther Targets 2013;17:667-80. [Crossref] [PubMed]
- Ijichi H, Chytil A, Gorska AE, et al. Inhibiting Cxcr2 disrupts tumor-stromal interactions and improves survival in a mouse model of pancreatic ductal adenocarcinoma. J Clin Invest 2011;121:4106-17. [Crossref] [PubMed]
- Özdemir BC, Pentcheva-Hoang T, Carstens JL, et al. Depletion of carcinoma-associated fibroblasts and fibrosis induces immunosuppression and accelerates pancreas cancer with reduced survival. Cancer Cell 2014;25:719-34. [Crossref] [PubMed]
- Rhim AD, Oberstein PE, Thomas DH, et al. Stromal elements act to restrain, rather than support, pancreatic ductal adenocarcinoma. Cancer Cell 2014;25:735-47. [Crossref] [PubMed]
- Highfill SL, Cui Y, Giles AJ, et al. Disruption of CXCR2-mediated MDSC tumor trafficking enhances anti-PD1 efficacy. Sci Transl Med 2014;6:237ra67 [Crossref] [PubMed]
- Reid MD, Basturk O, Thirabanjasak D, et al. Tumor-infiltrating neutrophils in pancreatic neoplasia. Mod Pathol 2011;24:1612-9. [Crossref] [PubMed]
- Diaz-Montero CM, Salem ML, Nishimura MI, et al. Increased circulating myeloid-derived suppressor cells correlate with clinical cancer stage, metastatic tumor burden, and doxorubicin-cyclophosphamide chemotherapy. Cancer Immunol Immunother 2009;58:49-59. [Crossref] [PubMed]
- Steele CW, Karim S, Leach JG, et al. CXCR2 Inhibition Profoundly Suppresses Metastases and Augments Immunotherapy in Pancreatic Ductal Adenocarcinoma. Cancer Cell 2016;29:832-45. [Crossref] [PubMed]
- Wang S, Wu Y, Hou Y, et al. CXCR2 macromolecular complex in pancreatic cancer: a potential therapeutic target in tumor growth. Transl Oncol 2013;6:216-25. [Crossref] [PubMed]
- Lu G, Wu Y, Jiang Y, et al. Structural insights into neutrophilic migration revealed by the crystal structure of the chemokine receptor CXCR2 in complex with the first PDZ domain of NHERF1. PLoS One 2013;8:e76219 [Crossref] [PubMed]
- Wu Y, Wang S, Farooq SM, et al. A chemokine receptor CXCR2 macromolecular complex regulates neutrophil functions in inflammatory diseases. J Biol Chem 2012;287:5744-55. [Crossref] [PubMed]
- Cancer Research UK. Ovarian Cancer Key Facts: Cancer Research UK. 2014; Available online: http://www.cancerresearchuk.org/cancer-info/cancerstats/keyfacts/ovarian-cancer/. Accessed on 8 August 2016.
- Barbieri F, Bajetto A, Porcile C, et al. CXC receptor and chemokine expression in human meningioma: SDF1/CXCR4 signaling activates ERK1/2 and stimulates meningioma cell proliferation. Ann N Y Acad Sci 2006;1090:332-43. [Crossref] [PubMed]
- Juffermans NP, Dekkers PE, Peppelenbosch MP, et al. Expression of the chemokine receptors CXCR1 and CXCR2 on granulocytes in human endotoxemia and tuberculosis: involvement of the p38 mitogen-activated protein kinase pathway. J Infect Dis 2000;182:888-94. [Crossref] [PubMed]
- Burger M, Hartmann T, Burger JA, et al. KSHV-GPCR and CXCR2 transforming capacity and angiogenic responses are mediated through a JAK2-STAT3-dependent pathway. Oncogene 2005;24:2067-75. [Crossref] [PubMed]
- Sai J, Walker G, Wikswo J, et al. The IL sequence in the LLKIL motif in CXCR2 is required for full ligand-induced activation of Erk, Akt, and chemotaxis in HL60 cells. J Biol Chem 2006;281:35931-41. [Crossref] [PubMed]
- Dong YL, Kabir SM, Lee ES, et al. CXCR2-driven ovarian cancer progression involves upregulation of proinflammatory chemokines by potentiating NF-kappaB activation via EGFR-transactivated Akt signaling. PLoS One 2013;8:e83789 [Crossref] [PubMed]
- Dobrzycka B, Terlikowski SJ, Kowalczuk O, et al. Circulating levels of TNF-alpha and its soluble receptors in the plasma of patients with epithelial ovarian cancer. Eur Cytokine Netw 2009;20:131-4. [PubMed]
- Macciò A, Madeddu C. Inflammation and ovarian cancer. Cytokine 2012;58:133-47. [Crossref] [PubMed]
- Son DS, Kabir SM, Dong YL, et al. Inhibitory effect of tumor suppressor p53 on proinflammatory chemokine expression in ovarian cancer cells by reducing proteasomal degradation of IkappaB. PLoS One 2012;7:e51116 [Crossref] [PubMed]
- Son DS, Parl AK, Rice VM, et al. Keratinocyte chemoattractant (KC)/human growth-regulated oncogene (GRO) chemokines and pro-inflammatory chemokine networks in mouse and human ovarian epithelial cancer cells. Cancer Biol Ther 2007;6:1302-12. [Crossref] [PubMed]
- Ning Y, Labonte MJ, Zhang W, et al. The CXCR2 antagonist, SCH-527123, shows antitumor activity and sensitizes cells to oxaliplatin in preclinical colon cancer models. Mol Cancer Ther 2012;11:1353-64. [Crossref] [PubMed]
- Iwasaki M, Zhao H, Jaffer T, et al. Volatile anaesthetics enhance the metastasis related cellular signalling including CXCR2 of ovarian cancer cells. Oncotarget 2016;7:26042-56. [PubMed]
- Luo X, Zhao H, Hennah L, et al. Impact of isoflurane on malignant capability of ovarian cancer in vitro. Br J Anaesth 2015;114:831-9. [Crossref] [PubMed]
- Huang H, Benzonana LL, Zhao H, et al. Prostate cancer cell malignancy via modulation of HIF-1α pathway with isoflurane and propofol alone and in combination. Br J Cancer 2014;111:1338-49. [Crossref] [PubMed]
- Benzonana LL, Perry NJ, Watts HR, et al. Isoflurane, a commonly used volatile anesthetic, enhances renal cancer growth and malignant potential via the hypoxia-inducible factor cellular signaling pathway in vitro. Anesthesiology 2013;119:593-605. [Crossref] [PubMed]
- Wilson C, Maxwell PJ, Longley DB, et al. Constitutive and treatment-induced CXCL8-signalling selectively modulates the efficacy of anti-metabolite therapeutics in metastatic prostate cancer. PLoS One 2012;7:e36545 [Crossref] [PubMed]
- Sharma B, Nawandar DM, Nannuru KC, et al. Targeting CXCR2 enhances chemotherapeutic response, inhibits mammary tumor growth, angiogenesis, and lung metastasis. Mol Cancer Ther 2013;12:799-808. [Crossref] [PubMed]
- Dabkeviciene D, Jonusiene V, Zitkute V, et al. The role of interleukin-8 (CXCL8) and CXCR2 in acquired chemoresistance of human colorectal carcinoma cells HCT116. Med Oncol 2015;32:258. [Crossref] [PubMed]
- Ning Y, Manegold PC, Hong YK, et al. Interleukin-8 is associated with proliferation, migration, angiogenesis and chemosensitivity in vitro and in vivo in colon cancer cell line models. Int J Cancer 2011;128:2038-49. [Crossref] [PubMed]