Pancreatic cancer immunotherapy: coming of age
Pancreatic cancer remains a deadly disease that kills ~40,000 Americans each year (1). The death rate from pancreatic cancer is steadily increasing and is anticipated to surpass that from prostate and breast cancer in the next two decades (2). The absence of any early detection markers as well as the lack of effective drugs has kept the survival rates from pancreatic cancer at <7% (3). This makes pancreatic cancer the only malignancy with an overall survival (OS) rate in single digit. This disease is therapy resistant and radiotherapy provides only modest benefits (4). Surgery is potentially the only curative treatment, however, the limited number of patients (<20%) that are eligible for surgical resection develop recurrences and succumb to the disease (5).
Pancreatic cancer therapy resistance is multi-factorial and emanates from both the macro and microenvironment of the tumor (6). Disturbances in multiple signaling pathways, particularly that of oncogenic K-ras driven (altered in >95% of pancreatic cancer), give a very complex network of interacting aberrations (7). Such complex interactions are not only hard to parse through; they are also difficult to perturb using drugs for meaningful therapeutic outcome. In terms of their microenvironment, pancreatic tumors are recognized to consist of heterogeneous cellular subtypes and are particularly marked for the presence of a dense desmoplastic stroma that is composed of activated stellate cells, extracellular matrix, immunosuppressive cell populations, such as regulatory T cells (Tregs), and limited protective immune surveillance due to the low number of cytotoxic CD8+ tumor infiltrating lymphocytes (TILs) that is observed in the majority of the patients (8-10). Both interstitial fluid and recently the less mobile, gel-fluid phase has been appreciated to be also involved in the observed hypoperfusion of pancreatic tumors (11). Additionally, there is increasing recognition of the presence of a side population of highly resistant cancer stem like cells with the propensity to undergo epithelial-to-mesenchymal transition (12). The dense desmoplastic stroma hinders proper drug penetration and simultaneously, the immunosuppressive microenvironment suppresses the activation of proper anti-tumor immune surveillance. This makes targeting the desmoplasia as well as conversion of an immune compromised microenvironment to a more immune competent setting an attractive therapeutic strategy against pancreatic cancer. Unfortunately, approaches to inhibit desmoplasia seem to trigger an acute immunogenic response that ends up promoting pancreatic tumorigenesis (13). Alternately, re-modeling of the microenvironment has been considered as a less drastic approach. However, any success in such approaches that reverse the recalcitrant pancreatic tumor to more responsive sub-type is still awaited.
Cancer immunotherapy has gained traction in recent years (14). The concept is particularly appealing for pancreatic cancer where immune compromised status hinders anti-tumor response from drug treatments (15). Presently, there are 66 clinical trials listed on ClinicalTrials.gov website involving pancreatic cancer and immunotherapy (using key words search “Pancreatic cancer”; “immunotherapy”). These studies range from the use of checkpoint inhibitors (also known as immune modulators), vaccines, adoptive cell transfer, monoclonal antibodies, oncolytic viruses, adjuvant immunotherapies, and cytokines.
Among the mediators of immune tolerance, the program death receptor-1 (PD-1) and its ligand, program death ligand 1 (PD-L1) feature as the top molecules (16). They differ in their location as well as cell origin. PD-L1 is usually expressed in parenchymal and myeloid cells, whereas PD-1 is expressed on activated T cells (17,18). Binding of PD-1 to PD-L1 exhausts T cells, a process termed anergy. This hinders proper anti-tumor immune responses, resulting in tumor growth and progression. There are several mechanisms that activate PD-L1. PD-L1 expression was found to be activated in a silica induced inflammation in a lung cancer model (19). Interestingly, K-RAS mutational profile of these tumors demonstrated a change from Q61R to G12D mutations in the inflammatory milieu. These hold relevance to pancreatic cancer which is known to harbor inflammatory microenvironment and are predominantly dependent on K-ras mutation. Disturbed K-ras signaling is also recognized to drive downstream canonical signaling such as PI3K/Akt pathway a well-recognized activator of PD-L1 (20). Interestingly, Nomi and colleagues demonstrated that monoclonal antibodies against PD-L1 or PD-1 caused a marked increase in antitumor efficacy on pancreatic cancer xenograft. PD-L1 blockade promoted CD8(+) T-cell infiltration into the tumor and induced local immune activation (21). In the same study the authors also demonstrated that gemcitabine could synergize with anti-PD-L1 monoclonal antibody resulting in complete elimination of pancreatic cancer tumors grown in mice and such combination lacked toxicity.
Based on the critical role of immune systems in promoting pancreatic tumor therapy resistance, as well as the pre-clinical data presented above, one promising approach is the use of immune checkpoint inhibitors (ICIs). ICIs work by targeting molecules that serve as repressors of anti-tumor immune response. Inhibition of immune inhibitory molecules results in activating stimulatory molecules, these treatments are designed to unleash or enhance pre-existing anti-cancer immune responses. Several checkpoint inhibitors, targeting multiple different checkpoints, are currently in development. There are two distinct approaches (I) targeting PD-1 (Table 1); and (II) targeting PD-L1 (Table 2).
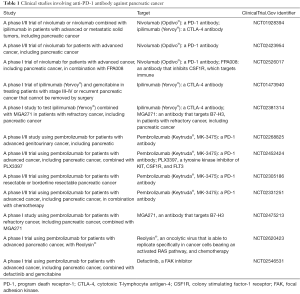
Full table
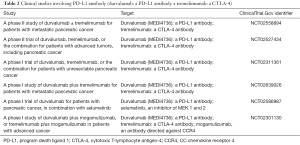
Full table
The ICIs targeting the PD-1/PD-L1 pathway and the cytotoxic T-lymphocyte antigen-4 (CTLA-4) have shown significant improvements in clinical responses in patients with melanoma, renal cell carcinoma, non-small cell lung cancer [reviewed in (22)]. Nevertheless, despite initially promising preclinical findings, administration of these agents as monotherapy in pancreatic ductal adenocarcinoma (PDAC) failed to demonstrate anti-tumor activity. Attempts have been made to understand such lack of activity and researchers have been able to pinpoint certain mechanisms underlying resistance to both PD-1 and PD-L1 therapies. One plausible explanation for the lack of efficacy of these agents in PDAC was attributed to low level of tumor infiltration by effector immune cells and the presence of fibroblast activation protein (FAP)-positive mesenchymal stromal cells that mediate immunosuppression via the CXCR4/CXCL-12 chemokine pathways (23).
Working in this direction, in a recent paper, Diana and colleagues evaluated the prognostic value of PD-1 and PD-L1 together with CD8+ TILs and FOXP3+ Tregs in resectable PDAC samples treated with adjuvant chemotherapy (24). This research group immunohistochemically evaluated whole-mount formalin fixed paraffin embedded (FFPE) tissue sections from 145 pancreatectomies for PD-1, PD-L1, CD8 and FOXP3. The goals of this study were to correlate the expression of these markers with clinicopathological characteristics, and OS, progression-free survival (PFS), local progression-free survival (LPFS) and distant metastases free-survival (DMFS), in the background of stroma density (haematoxylin-eosin) and activity (alpha-smooth muscle actin) and taking into consideration the intratumoral lymphoid aggregates. The median OS range was kept between 2–69 months with median OS was 21 months after a mean follow-up of 20 months. In the multivariate analysis performed on these 145 pancreatectomies, high PD-1+ TILs expression was associated with better OS, LPFS and DMFS (statistical significance P<0.05). Similar findings were observed for CD8+ TILs. Of interest, the expression of FOXP3 and PD-L1 did not demonstrate any prognostic significance. The distribution of TILs in their selected dataset was found to be heterogeneous. Nevertheless, what is intriguing is that in their tumors harboring higher stromal density showed greater infiltration of CD8+ TILs than that in loose density stroma. As anticipated, the authors could not find any correlation with stromal activity. The study also captured lymphoid aggregates in more than 50% of tumors. In these cases the presence of PD-1+ TILs was associated with better OS, LPFS and DMFS, whereas CD8+ TILs only correlated with superior LPFS. PD-1+ and CD8+ TILs (statistical significance P<0.05).
Conclusions
Cancer immunotherapy has been touted as the beginning of the end of cancer (25). At present there are more than 1,450 clinical studies in relation to cancer immunotherapy and more specifically involving ICIs against PD1 and PD-L1. Immunotherapy is particularly appealing concept for pancreatic cancer simply because it is one of the most immune compromised tumor system. As presented in the article by Diana and colleagues, both PD-1 and PD-L1 alongside the CD8+ TILs and FOXP3+ Tregs hold prognostic value for pancreatic cancer. These findings can be considered as guidelines for evaluation of PD-1/PD-L1 in the context of desmoplastic stroma and could help guide future immunotherapies in PDAC. Despite these convincing results, a major caveat associated with this study is that the median OS follow up was kept at 21 months. This may not be the most ideal range given that studies with substantially longer median OS have been reported in certain settings for pancreatic cancer patients. Although briefly described by the authors, the possibility of potential selection bias due to the retrospective nature of the study cannot be ruled out. It will be worthwhile to perform a detailed pathological examination in retrospective cohort to obtain concrete answers on the role of CD8+ and PD-1+ TILs as valid and robust prognostic markers to identify PDAC patients with a more favorable outcome.
Acknowledgments
Funding: Work in the lab of RMM is supported by the SKY Foundation, James H Thie foundation and Perri Foundation.
Footnote
Provenance and Peer Review: This article was commissioned and reviewed by the Section Editor Xiaotian Sun (Department of Internal Medicine Clinic of August First Film Studio, Beijing, China).
Conflicts of Interest: Both authors have completed the ICMJE uniform disclosure form (available at http://dx.doi.org/10.21037/tcr.2016.10.60). The authors have no conflicts of interest to declare.
Ethical Statement: The authors are accountable for all aspects of the work in ensuring that questions related to the accuracy or integrity of any part of the work are appropriately investigated and resolved.
Open Access Statement: This is an Open Access article distributed in accordance with the Creative Commons Attribution-NonCommercial-NoDerivs 4.0 International License (CC BY-NC-ND 4.0), which permits the non-commercial replication and distribution of the article with the strict proviso that no changes or edits are made and the original work is properly cited (including links to both the formal publication through the relevant DOI and the license). See: https://creativecommons.org/licenses/by-nc-nd/4.0/.
References
- Siegel RL, Miller KD, Jemal A. Cancer statistics, 2016. CA Cancer J Clin 2016;66:7-30. [Crossref] [PubMed]
- Rahib L, Smith BD, Aizenberg R, et al. Projecting cancer incidence and deaths to 2030: the unexpected burden of thyroid, liver, and pancreas cancers in the United States. Cancer Res 2014;74:2913-21. [Crossref] [PubMed]
- Mirkin KA, Hollenbeak CS, Wong J. Survival impact of neoadjuvant therapy in resected pancreatic cancer: A Prospective Cohort Study involving 18,332 patients from the National Cancer Data Base. Int J Surg 2016;34:96-102. [Crossref] [PubMed]
- Chand S, O'Hayer K, Blanco FF, et al. The Landscape of Pancreatic Cancer Therapeutic Resistance Mechanisms. Int J Biol Sci 2016;12:273-82. [Crossref] [PubMed]
- Ishida J, Toyama H, Matsumoto I, et al. Second primary pancreatic ductal carcinoma in the remnant pancreas after pancreatectomy for pancreatic ductal carcinoma: High cumulative incidence rates at 5 years after pancreatectomy. Pancreatology 2016;16:615-20. [Crossref] [PubMed]
- Ying H, Dey P, Yao W, et al. Genetics and biology of pancreatic ductal adenocarcinoma. Genes Dev 2016;30:355-85. [Crossref] [PubMed]
- Sahin IH, Iacobuzio-Donahue CA, O'Reilly EM. Molecular signature of pancreatic adenocarcinoma: an insight from genotype to phenotype and challenges for targeted therapy. Expert Opin Ther Targets 2016;20:341-59. [Crossref] [PubMed]
- Apte M, Pirola RC, Wilson JS. Pancreatic stellate cell: physiologic role, role in fibrosis and cancer. Curr Opin Gastroenterol 2015;31:416-23. [Crossref] [PubMed]
- Evans A, Costello E. The role of inflammatory cells in fostering pancreatic cancer cell growth and invasion. Front Physiol 2012;3:270. [PubMed]
- Xu Z, Pothula SP, Wilson JS, et al. Pancreatic cancer and its stroma: a conspiracy theory. World J Gastroenterol 2014;20:11216-29. [Crossref] [PubMed]
- DuFort CC, DelGiorno KE, Hingorani SR. Mounting Pressure in the Microenvironment: Fluids, Solids, and Cells in Pancreatic Ductal Adenocarcinoma. Gastroenterology 2016;150:1545-1557.e2. [Crossref] [PubMed]
- Sancho P, Alcala S, Usachov V, et al. The ever-changing landscape of pancreatic cancer stem cells. Pancreatology 2016;16:489-96. [Crossref] [PubMed]
- Carr RM, Fernandez-Zapico ME. Pancreatic cancer microenvironment, to target or not to target? EMBO Mol Med 2016;8:80-2. [Crossref] [PubMed]
- Neves H, Kwok HF. Recent advances in the field of anti-cancer immunotherapy. BBA Clin 2015;3:280-8. [Crossref] [PubMed]
- Jimenez-Luna C, Prados J, Ortiz R, et al. Current Status of Immunotherapy Treatments for Pancreatic Cancer. J Clin Gastroenterol 2016;50:836-48. [Crossref] [PubMed]
- Gandini S, Massi D, Mandalà M. PD-L1 expression in cancer patients receiving anti PD-1/PD-L1 antibodies: A systematic review and meta-analysis. Crit Rev Oncol Hematol 2016;100:88-98. [Crossref] [PubMed]
- Topalian SL, Hodi FS, Brahmer JR, et al. Safety, activity, and immune correlates of anti-PD-1 antibody in cancer. N Engl J Med 2012;366:2443-54. [Crossref] [PubMed]
- Topalian SL, Taube JM, Anders RA, et al. Mechanism-driven biomarkers to guide immune checkpoint blockade in cancer therapy. Nat Rev Cancer 2016;16:275-87. [Crossref] [PubMed]
- Freire J, Ajona D, de Biurrun G, et al. Silica-induced chronic inflammation promotes lung carcinogenesis in the context of an immunosuppressive microenvironment. Neoplasia 2013;15:913-24. [Crossref] [PubMed]
- Zhang Y, Zhang J, Xu K, et al. PTEN/PI3K/mTOR/B7-H1 signaling pathway regulates cell progression and immuno-resistance in pancreatic cancer. Hepatogastroenterology 2013;60:1766-72. [PubMed]
- Nomi T, Sho M, Akahori T, et al. Clinical significance and therapeutic potential of the programmed death-1 ligand/programmed death-1 pathway in human pancreatic cancer. Clin Cancer Res 2007;13:2151-7. [Crossref] [PubMed]
- Menderes G, Hicks C, Black JD, et al. Immune checkpoint inhibitors in gynecologic cancers with lessons learned from non-gynecologic cancers. Expert Opin Biol Ther 2016;16:989-1004. [Crossref] [PubMed]
- Ene-Obong A, Clear AJ, Watt J, et al. Activated pancreatic stellate cells sequester CD8+ T cells to reduce their infiltration of the juxtatumoral compartment of pancreatic ductal adenocarcinoma. Gastroenterology 2013;145:1121-32. [Crossref] [PubMed]
- Diana A, Wang LM, D'Costa Z, et al. Prognostic value, localization and correlation of PD-1/PD-L1, CD8 and FOXP3 with the desmoplastic stroma in pancreatic ductal adenocarcinoma. Oncotarget 2016; [Epub ahead of print]. [PubMed]
- Farkona S, Diamandis EP, Blasutig IM. Cancer immunotherapy: the beginning of the end of cancer? BMC Med 2016;14:73. [Crossref] [PubMed]