Proton beam therapy for intracranial and skull base tumors
Introduction
Surgical resection is the most fundamental treatment for brain tumors; however, it is sometimes difficult to put wide surgical margins because of damage in the surrounding normal brain tissues such as the cranial nerves, the brainstem, and others. This renders the roles of postoperative radiotherapy (PORT) important or even essential. Particle radiotherapy such as proton beam therapy (PBT) and carbon ion therapy has better dose distribution than photon radiotherapy owing to a narrow energy peak called Bragg peak. Because of this excellent physical property, particle radiotherapy is suitable for treating brain tumors because high doses can be delivered to the target while preserving the surrounding normal tissue. In addition, because it is possible to make irregular target fields, particle radiotherapy can enable us to deliver uniform doses to irregularly shaped tumors.
Proton beams are categorized as low linear energy transfer (LET) radiation with a biological effect of 1.1 times that of photon beams (1). In contrast, carbon ions have a higher biological effectiveness than protons or photons; their effectiveness is almost 2-3 times that of photon beams (2,3), and they may be effective for treating highly radioresistant tumors. In this review, we describe current findings, particularly on PBT for brain tumors, including glioblastoma (GBM), low-grade glioma (LGG), chordoma, and meningioma.
PBT for low-grade glioma
Sequential PORT is recommended in some cases of LGG, depending on the tumor location, extent of residual disease, and proliferative potency. However, the significance of PORT for LGG is still not very clear (4-6). In the EORTC 22845 trial, progression-free survival (PFS) was significantly better in patients who received early PORT at a dose of 54 Gy in 30 fractions compared with that in patients who did not receive radiotherapy (P<0.0001); however, there was no significant difference in the overall survival (OS) (P=0.872) (5). The current recommended dose is 50-55 Gy in conventional fractions (1.8-2 Gy per fraction). Fitzek et al. used PBT in combination with photon beams at doses of 68.2 and 79.7 GyE for glioma of grades 2 and 3, respectively, with dose escalation. However, they were not able to improve the outcome significantly (7). In contrast, Hug et al. reported an improved visual status in 27 pediatric patients with LGG treated with PBT at a median dose of 55.2 Gy (RBE) at 1.8 Gy per fraction (8). With the use of PBT for adult LGG at a median dose of 54 Gy (RBE) at 1.8 Gy per fraction, Hauswald et al. found that tumor progression was noticed in only 1 patient and there was no severe toxicity (9).
The prognosis of patients with LGG is better compared with that of patients with high-grade glioma. The 5- and 10-year OS rates have been reported to be 58% and 32%, respectively, for astrocytoma (10) and 88% and 85%, respectively, for low-grade oligodendroglioma (11). Therefore, the quality of life after treatment is an important consideration for patients with LGG, particularly younger patients. The advantages of PBT in the preservation of the healthy brain and in the reduction of risk for secondary cancer in patients with pediatric brain tumors have been widely suggested (12-19). Further clinical analyses of not only neuro-functional preservation but also secondary cancer prevention are required to establish the efficacy of PBT in younger patients with LGG.
PBT for high-grade glioma
PORT improves OS of patients with high-grade glioma (HGG) (20). Postoperative photon radiotherapy of approximately 60 Gy in conventional fractions with concomitant and adjuvant temozolomide is currently the standard therapy (21). However, the prognosis is still not satisfactory, and it has shown little improvement in patients with GBM over the past decades. The median survival times (MSTs) of patients with HGG have been reported to be 11-18 months, and the 5-year survival rates have been reported to be less than 10% (20-24). Outcomes were not improved even high linear-energy transfer (LET) radiations were applied. MSTs of 13.9 and 9 months were reported using radiation with carbon, helium, and neon (25) and pions, respectively (26). In contrast, dose escalation has yielded favorable results. Fitzek et al. found that accelerated fractionation of 90 Gy (RBE) using conformal protons and photons improved local control and survival in GBM, with MST of the 23 cases being 20 months (27). In their report, tumor recurrence occurred most commonly in areas that received doses of 60-70 Gy (RBE) or less, and only 1 case showed recurrence within areas that received 90 Gy (RBE).
In patients with GBM, Tanaka et al. reported MSTs of 16.2 (12.8-19.6) and 12.4 (10.0-14.8) months and 2-year survival rates of 38.4% (23.5-53.3%) and 11.4% (0.0-25.3%) after treatment with high-dose conformal X-ray radiotherapy of 80-90 Gy and 60 Gy, respectively. The patients who received 60 Gy did not develop radiation necrosis (RN), whereas 9 patients who received higher doses (80-90 Gy) developed RN. In addition, Mizumoto et al. also found a survival benefit of hyperfractionated concomitant boost PBT with nimustine hydrochloride (ACNU) in patients with GBM (28). In this study, radiotherapy was conducted for 20 patients with GBM twice a day as follows: 1.8 Gy (RBE) for the tumor and surrounding edema in the morning and 1.65 Gy (RBE) for the gross tumor on gadolinium-enhanced MRI in the afternoon (6 h later) over 28 days; a total dose of 96.6 Gy (RBE) in 56 fractions was delivered to the tumor. Only 1 of 20 patients had recurrence within the 96.6 Gy (RBE) volume. The MST was 21.6 months and the 1- and 2-year PFS was 45.5% and 15.5%, respectively. RN occurred in 6 cases, and probable leukoencephalopathy was observed in 1 patient. Although dose escalation may be associated with an increased risk of RN, the development of RN might be inevitable in patients with GBM when considering its refractoriness. The findings of Fitzek et al. and Mizumoto et al. indicate that a total dose ≥90 GyE has the potential to control GBM. However, because the accurate evaluation of the area of tumor infiltration was difficult with MRI or CT, recurrence mainly occurred in the marginal area. This fact may indicate that the use of methionine positron emission tomography facilitates the evaluation of exact tumor invasion and improves the outcome.
Carbon ions are also of interest for the treatment of HGG. Mizoe et al. conducted a phase I/II study in 48 patients with HGG (16 with anaplastic astrocytoma and 32 with GBM) using photon and carbon ion therapy with ACNU (29). The radiation dose with photon beams was 50 Gy in 25 fractions, followed by carbon ion therapy. The doses of carbon ions were escalated in 10% incremental steps from 16.8 to 24.8 Gy, and a high dose was associated with good prognosis with MST of 26 months.
Suit et al. suggested 2 directions for future radiotherapy, i.e., the shrinkage of the irradiation field and expansion of a gap between radiosensitivity of tumor and normal tissues (30). In this regard, PBT or carbon ion therapy has considerable potential to achieve the shrinkage of the irradiation field, in particular, by developing intensity-modulated particle therapy (IMPT). A recent study on radiosensitivity showed the feasibility of radiotherapy in combination with not only temozolomide but also other molecular-targeted agents (31,32). Therefore, high-dose particle therapy in combination with recently developed molecular-targeted or radiosensitizing agents should be tried as a promising multimodal treatment for GBM. In addition, boron neutron capture therapy (BNCT) may permit the expansion of the radiosensitivity gap. This approach is based on a nuclear capture reaction that occurs when boron (10B) is irradiated with neutrons of thermal energy to yield high-energy short-range α particles (4He2+) and recoiling lithium (7Li) nuclei. Because 10B is selectively taken up by cancer cells, theoretically, only cancer cells are destroyed by this treatment. The outcomes of patients with GBM treated with BNCT have been found to be favorable, with MSTs of 13-27 months (33-36).
PBT for skull base chordomas and chondrosarcomas
Chordomas are rare low-grade malignancies that often develop in the clival region. Because metastasis and dissemination are uncommon, local control by aggressive treatment is important for long-term survival. Although surgical resection is the first treatment choice, gross total resection is often difficult because of difficult access and proximity to critical structures. Therefore, adjuvant radiotherapy is almost essential for tumor control. The control of skull base chordomas or chondrosarcomas requires doses more than 56-70 Gy (37-39) that are beyond the tolerance of critical structures such as the optic nerve, chiasma, and brainstem. This makes the treatment with photon beams difficult, it has been reported that 5-year PFS with X-ray treatment remained 17-39% (40-43). In this regard, particle radiotherapy was shown to be superior to photons for delivering higher doses to the tumor while keeping lower doses to normal tissues in the clival region in the 1990s (29,44-50).
The use of PBT for treating chordoma dates back to the 1990s. In early studies, PBT was often conducted in combination with photon radiotherapy. For example, Munzenrider et al. treated 519 patients with skull base tumors, including 290 chordomas and 229 chondrosarcomas, with photon and proton radiotherapy at a total dose of 66-83 Gy (RBE) at 1.8-1.92 Gy per fraction, and 10-year local control rates (LCRs) for chordoma and chondrosarcoma were 54% and 88%, respectively. Similarly, Hug et al. treated 58 patients (33 with chordomas and 25 with chondrosarcomas) with a median total dose of 71.9 Gy (RBE) and 69.3 Gy (RBE) for chordoma and chondrosarcoma, respectively, at 1.8 Gy (RBE) per fraction and found that 5-year LCRs for chordoma and chondrosarcoma were 59% and 75%, respectively, with symptomatic late toxicities being observed in only 3 of the 58 patients.
Ares et al. reported the outcomes of 42 patients with skull base chordomas and 21 patients with chondrosarcomas treated with only PBT. The dose for chordoma and chondrosarcoma was 73.5 Gy (RBE) and 68.4 Gy (RBE), respectively, at 1.8-2.0 Gy (RBE) dose per fraction. The 5-year LCR was 81% and 94% for chordoma and chondrosarcoma, respectively, and severe toxicities were observed in 4 patients, resulting in 94% 5-year freedom from severe toxicity (44). In addition, Fuji et al. obtained a 3-year LCR and OS of 100% in 8 patients with skull base chordomas using a prescribed dose of 70 Gy (RBE) at 1.8 Gy (RBE) per fraction (51). In 96 patients with chordomas treated with carbon ion therapy at a median dose of 60 Gy (RBE) delivered in 20 fractions within 3 weeks, Shulz-Ertner et al. obtained a 5-year LCR of 70%. Meanwhile, grade 3 optic nerve neuropathy and fat plomb necrosis occurred in 4 patients and 1 patient, respectively (48). Mizoe et al. conducted a phase I/II study of carbon ion therapy for skull base chordoma with dose escalation in 4 steps of 4.8 Gy from 48 to 60.8 Gy (RBE) in 16 fractions and obtained a 5-year LCR of 100% without severe toxicities (52).
Recent development of photon radiotherapy has enabled us to achieve a confocal and precise dose distribution. Debus et al. performed stereotactic radiotherapy (SRT) in 37 patients at a dose of 66.6 Gy with a median fraction size of 1.8 Gy, whereas Foweraker et al. treated 9 chordoma patients with radiotherapy at a dose of 65 Gy in 39 fractions (53). In meta-analysis of these recent studies, most of which are small and retrospective, Maio et al. found that 5-year PFS and OS were 50.8% and 78.4%, respectively, and no significant differences in 5-year OS were observed among photon radiotherapy, gamma-knife surgery, PBT, and carbon ion therapy, but 5-year PFS was lower in gamma-knife surgery (54). Although doses of photons delivered were lower than those of protons and carbon ions, these results suggest that chordoma may possibly be controlled when a sufficient dose is delivered, regardless of the radiation quality. Further progress both in photon and particle radiotherapy is definitely required to overcome this radioresistant and invasive tumor that develop at the very complicated location.
Medial temporal lobe injury is another risk in particle radiotherapy of the middle and upper clival region, which results in amnesia with a specific memory impairment profile (55-57). Ten of 96 patients (10.4%) reportedly developed clinical symptoms or MRI changes in the temporal lobe after PBT at doses of 63-74 Gy (RBE) (58). Although a correlation between the occurrence of temporal lobe toxicities and tissue volume that received high doses has been suggested (58), a threshold dose for temporal lobe toxicity has not been clarified till date.
Collectively, at present, the treatment modality is selected on the basis of not only the tumor location, size, and shape but also the experiences of each institute. Although it takes at least 5 years to draw a meaningful result in chordomas and chondrosarcomas, clinical evidence with higher grades is highly desirable.
PBT for meningioma
Meningiomas are classified into 3 grades in the WHO classification. Most are benign or WHO grade I with an indolent course or a low rate of local recurrence. The first treatment choice for meningiomas is complete surgical resection. Condrao et al. found that 5- and 10-year LCRs after gross total resection (GTR) were 93% and 80%, respectively (59). However, complete resection with Simpson G-I or II is sometimes difficult owing to the location, size, and involvement of vial vessels or cranial nerves. In addition, it has been reported that subtotal resection (STR) is associated with a higher recurrence rate of 45-60% (60,61). In these patients, PORT significantly improves local control, although meningiomas are less sensitive to radiotherapy (61-64). Taylor et al. found that 69% of patients had recurrence after STR alone, compared with only 15% after PORT (P=0.01) (63).
As for atypical meningiomas, the role of PORT is not sufficiently proven; however, at present, PORT is recommended for all patients, regardless of GTR or STR (65-68). Since the revision of WHO grading criteria in 2000, there has been a significant increase in the number of meningiomas diagnosed as grade II (69,70). Among 114 patients diagnosed with WHO grade II meningioma, Mair et al. found a benefit of PORT only for patients who underwent STR (68). In contrast, Komotar et al. suggested that PORT improved local control even after GTR. They mentioned that recurrence was noticed only in 1 of 13 patients who received PORT, while it was noticed in 13 of 32 patients who did not receive PORT (P=0.085) (71). The typical dose for PORT is 50-54 Gy and 60 Gy for benign and atypical meningiomas, respectively. In addition, dose escalation may improve local control, particularly for atypical meningioma (72-74), and SRT is reportedly effective for treating small and unresectable meningiomas (75-79).
Hug et al. reported the outcomes of 31 patients with WHO grade 2 and 3 meningiomas treated with photon or combined proton-photon irradiation. The total doses ranged from 50 to 68 Gy (RBE) and 40 to 72 Gy (RBE) for grades 2 and 3 meningiomas, respectively. Local control was significantly improved with PBT compared with that with photon radiotherapy alone (P=0.025), and the survival rates for WHO grade 2 meningiomas were significantly improved by PBT and radiation doses >60 Gy (RBE) (74). Similarly, in a study of 24 patients with WHO II and III meningiomas treated by proton and photon beams with a median dose of 65.1 Gy (RBE), Boscos et al. found that survival was significantly associated with the total dose (72). In a recent report on the outcomes of 6 patients with WHO II and III meningiomas treated at doses of 68.4 Gy (RBE) and 72.0 Gy, respectively; Chan et al. observed local recurrence in 1 patient with WHO grade 3 meningioma but found no severe toxicity (73).
With regard to the use of SRT for meningiomas, Selch et al. found that 3-year PFS was 97.4% in a study of 41 cases of benign cavernous sinus meningioma (median tumor volume, 14.5 cc) treated with SRT (median dose, 50.4 Gy) after incomplete resection (77). In addition, in 12 cases of high-grade meningioma (tumor volume, 4.4 cc) treated with SRT at a mean marginal dose of 12-20 Gy, Kano et al. found that a marginal dose <20 Gy was a significant predictor (P=0.0139) of short-term tumor progression (5-year PFS, 29.4% vs. 63.1%) (79).
PBT is advantageous for treating tumors that are large or have a complex shape or for those adjacent to critical regions (80-85). Thus, in 46 patients with partially resected, biopsied, or recurrent meningiomas treated with a combination of photon and protons at median doses of 59 Gy (RBE), Wenkel et al. found that 5- and 10-year LCRs were 100% and 88%, respectively (84). IMRT also gives excellent dose distribution with the avoidance of surrounding organs (86). Kosaki et al. reported that the difference in dose distribution between IMRT and PBT for complex-shaped skull base meningiomas was very small. However, they mentioned that PBT allowed almost complete avoidance of the brainstem, while this region received 10-30% of the dose in IMRT (87). In an evaluation of the risk of a secondary tumor after radiotherapy for benign meningiomas, Arvot et al. found that PBT reduced this risk to less than half of that with photon radiotherapy (81). In addition, they also mentioned that PBT could significantly reduce the risk of neurocognitive, visual, and auditory complications. A summary of proton beam therapy for intracranial and skull base tumors is reported in Table 1.
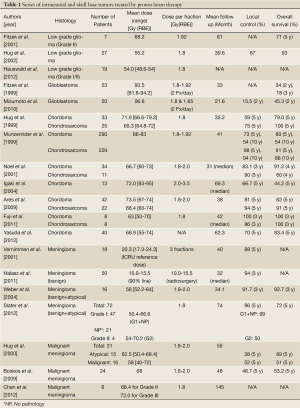
Full table
Conclusions
The role of particle beam radiotherapy for intracranial and skull base tumors has not been fully established. However, it is clear that this method has 2 important advantages compared with photon radiotherapy. First, particle beam radiotherapy makes it possible to deliver a high dose to refractory tumors such as high-grade gliomas and anaplastic or atypical meningiomas, which can improve control rates of these tumors. Second, PBT can reduce the irradiated volume of normal brain tissues, thereby significantly reducing neurotoxicity. Therefore, particle beam radiotherapy is particularly advantageous for childhood brain tumors, low-grade gliomas, and benign meningiomas that require a long-term follow-up.
Acknowledgments
Funding: None.
Footnote
Conflicts of Interest: All authors have completed the ICMJE uniform disclosure form (available at http://dx.doi.org/10.3978/j.issn.2218-676X.2013.04.08). KT serves as an unpaid editorial board member of Translational Cancer Research. The other authors have no conflicts of interest to declare.
Ethical Statement: The authors are accountable for all aspects of the work in ensuring that questions related to the accuracy or integrity of any part of the work are appropriately investigated and resolved.
Open Access Statement: This is an Open Access article distributed in accordance with the Creative Commons Attribution-NonCommercial-NoDerivs 4.0 International License (CC BY-NC-ND 4.0), which permits the non-commercial replication and distribution of the article with the strict proviso that no changes or edits are made and the original work is properly cited (including links to both the formal publication through the relevant DOI and the license). See: https://creativecommons.org/licenses/by-nc-nd/4.0/.
References
- Paganetti H, Niemierko A, Ancukiewicz M, et al. Relative biological effectiveness (RBE) values for proton beam therapy. Int J Radiat Oncol Biol Phys 2002;53:407-21. [PubMed]
- Koike S, Ando K, Oohira C, et al. Relative biologicaleffectiveness of 290 MeV/u carbon ions for the growth delay of a radioresistant murine fibrosarcoma. J Radiat Res 2002;43:247-55. [PubMed]
- Kagawa K, Murakami M, Hishikawa Y, et al. Preclinical biological assessment of proton and carbon ion beams at Hyogo Ion Beam Medical Center. Int J Radiat Oncol Biol Phys 2002;54:928-38. [PubMed]
- Shaw E, Arusell R, Scheithauer B, et al. Prospective randomized trial of low-versus high-dose radiation therapy in adults with supratentorial low-grade glioma: initial report of a North Central Cancer Treatment Group/Radiation Therapy Oncology Group/Eastern Cooperative Oncology Group study. J Clin Oncol 2002;20:2267-76. [PubMed]
- van den Bent MJ, Afra D, de Witte O, et al. Long-term efficacy of early versus delayed radiotherapy for low-grade astrocytoma and oligodendroglioma in adults: the EORTC 22845 randomised trial. Lancet 2005;366:985-90. [PubMed]
- Karim AB, Maat B, Hatlevoll R, et al. A randomized trial on dose-response in radiation therapy of low-grade cerebral glioma: European Organization for Research and Treatment of Cancer (EORTC) Study 22844. Int J Radiat Oncol Biol Phys 1996;36:549-56. [PubMed]
- Fitzek MM, Thornton AF, Harsh G 4th, et al. Dose-escalation with proton/photon irradiation for Daumas-Duport lower-grade glioma: results of an institutional phase I/II trial. Int J Radiat Oncol Biol Phys 2001;51:131-7. [PubMed]
- Hug EB, Muenter MW, Archambeau JO, et al. Conformal proton radiation therapy for pediatric low-grade astrocytomas. Strahlenther Onkol 2002;178:10-7. [PubMed]
- Hauswald H, Rieken S, Ecker S, et al. First experiences in treatment of low-grade glioma grade I and II with proton therapy. Radiat Oncol 2012;7:189. [PubMed]
- Shaw EG, Scheithauer BW, O’Fallon JR, et al. Mixed oligoastrocytomas: a survival and prognostic factor analysis. Neurosurgery 1994;34:577-82; discussion 582. [PubMed]
- Lebrun C, Fontaine D, Ramaioli A, et al. Long-term outcome of oligodendrogliomas. Neurology 2004;62:1783-7. [PubMed]
- Beltran C, Roca M, Merchant TE. On the benefits and risks of proton therapy in pediatric craniopharyngioma. Int J Radiat Oncol Biol Phys 2012;82:e281-7. [PubMed]
- Boehling NS, Grosshans DR, Bluett JB, et al. Dosimetric comparison of three-dimensional conformal proton radiotherapy, intensity-modulated proton therapy, and intensity-modulated radiotherapy for treatment of pediatric craniopharyngiomas. Int J Radiat Oncol Biol Phys 2012;82:643-52. [PubMed]
- Calaminus G, Göbel U. Proton beam therapy for loco-regional control of a recurrent mixed malignant germ cell tumor of the skull in a 22-month-old girl. Klin Padiatr 2010;222:175-9. [PubMed]
- Hug EB, Sweeney RA, Nurre PM, et al. Proton radiotherapy in management of pediatric base of skull tumors. Int J Radiat Oncol Biol Phys 2002;52:1017-24. [PubMed]
- Lee CT, Bilton SD, Famiglietti RM, et al. Treatment planning with protons for pediatric retinoblastoma,medulloblastoma,and pelvic sarcoma: how do protons compare with other conformal techniques? Int J Radiat Oncol Biol Phys 2005;63:362-72. [PubMed]
- MacDonald SM, Safai S, Trofimov A, et al. Proton radiotherapy for childhood ependymoma: initial clinical outcomes and dose comparisons. Int J Radiat Oncol Biol Phys 2008;71:979-86. [PubMed]
- MacDonald SM, Trofimov A, Safai S, et al. Proton radiotherapy for pediatric central nervous system germ cell tumors: early clinical outcomes. Int J Radiat Oncol Biol Phys 2011;79:121-9. [PubMed]
- St Clair WH, Adams JA, Bues M, et al. Advantage of protons compared to conventional X-ray or IMRT in the treatment of a pediatric patient with medulloblastoma. Int J Radiat Oncol Biol Phys 2004;58:727-34. [PubMed]
- Walker MD, Green SB, Byar DP, et al. Randomized comparisons of radiotherapy and nitrosoureas for the treatment of malignant glioma after surgery. N Engl J Med 1980;303:1323-9. [PubMed]
- Stupp R, Hegi ME, Mason WP, et al. Effects of radiotherapy with concomitant and adjuvant temozolomide versus radiotherapy alone on survival in glioblastoma in a randomised phase III study: 5-year analysis of the EORTC-NCIC trial. Lancet Oncol 2009;10:459-66. [PubMed]
- Walker MD, Alexander E Jr, Hunt WE, et al. Evaluation of BCNU and/or radiotherapy in the treatment of anaplastic gliomas. A cooperative clinical trial. J Neurosurg 1978;49:333-43. [PubMed]
- Kristiansen K, Hagen S, Kollevold T, et al. Combined modality therapy of operated astrocytomas grade III and IV. Confirmation of the value of postoperative irradiation and lack of potentiation of bleomycin on survival time: a prospective multicenter trial of the Scandinavian Glioblastoma Study Group. Cancer 1981;47:649-52. [PubMed]
- Sandberg-Wollheim M, Malmström P, Strömblad LG, et al. A randomized study of chemotherapy with procarbazine, vincristine, and lomustine with and without radiation therapy for astrocytoma grades 3 and/or 4. Cancer 1991;68:22-9. [PubMed]
- Castro JR, Saunders WM, Austin-Seymour MM, et al. A phase I-II trial of heavy charged particle irradiation of malignant glioma of the brain: a Northern California Oncology Group Study. Int J Radiat Oncol Biol Phys 1985;11:1795-800. [PubMed]
- Greiner R, Blattmann H, Thum P, et al. Anaplastic astrocytoma and glioblastoma:pion irradiation with the dynamic conformation technique at the Swiss Institute for Nuclear Research (SIN). Radiother Oncol 1990;17:37-46. [PubMed]
- Fitzek MM, Thornton AF, Rabinov JD, et al. Accelerated fractionated proton/photon irradiation to 90 cobalt gray equivalent for glioblastoma multiforme: results of a phase II prospective trial. J Neurosurg 1999;91:251-60. [PubMed]
- Mizumoto M, Tsuboi K, Igaki H, et al. Phase I/II trial of hyperfractionated concomitant boost proton radiotherapy for supratentorial glioblastoma multiforme. Int J Radiat Oncol Biol Phys 2010;77:98-105. [PubMed]
- Mizoe JE, Tsujii H, Hasegawa A, et al. Phase I/II clinical trial of carbon ion radiotherapy for malignant gliomas: combined X-ray radiotherapy, chemotherapy, and carbon ion radiotherapy. Int J Radiat Oncol Biol Phys 2007;69:390-6. [PubMed]
- Suit H. The Gray Lecture 2001: coming technical advances in radiation oncology. Int J Radiat Oncol Biol Phys 2002;53:798-809. [PubMed]
- Drappatz J, Norden AD, Wong ET, et al. Phase I study of vandetanib with radiotherapy and temozolomide for newly diagnosed glioblastoma. Int J Radiat Oncol Biol Phys 2010;78:85-90. [PubMed]
- Prados MD, Chang SM, Butowski N, et al. Phase II study of erlotinib plus temozolomide during and after radiation therapy in patients with newly diagnosed glioblastoma multiforme or gliosarcoma. J Clin Oncol 2009;27:579-84. [PubMed]
- Busse PM, Harling OK, Palmer MR, et al. A critical examination of the results from the Harvard-MIT NCT program phase I clinical trial of neutron capture therapy for intracranial disease. J Neurooncol 2003;62:111-21. [PubMed]
- Henriksson R, Capala J, Michanek A, et al. Boron neutron capture therapy (BNCT) for glioblastoma multiforme: a phase II study evaluating a prolonged high-dose of boronophenylalanine (BPA). Radiother Oncol 2008;88:183-91. [PubMed]
- Joensuu H, Kankaanranta L, Seppälä T, et al. Boron neutron capture therapy of brain tumors: clinical trials at the finnish facility using boronophenylalanine. J Neurooncol 2003;62:123-34. [PubMed]
- Kawabata S, Miyatake S, Kuroiwa T, et al. Boron neutron capture therapy for newly diagnosed glioblastoma. J Radiat Res 2009;50:51-60. [PubMed]
- Pearlman AW, Friedman M. Radical radiation therapy of chordoma. Am J Roentgenol Radium Ther Nucl Med 1970;108:332-41. [PubMed]
- Rich TA, Schiller A, Suit HD, et al. Clinical and pathologic review of 48 cases of chordoma. Cancer 1985;56:182-7. [PubMed]
- Noël G, Feuvret L, Calugaru V, et al. Chordomas of the base of the skull and upper cervical spine. One hundred patients irradiated by a 3D conformal technique combining photon and proton beams. Acta Oncol 2005;44:700-8. [PubMed]
- Catton C, O’Sullivan B, Bell R, et al. Chordoma: long-term follow-up after radical photon irradiation. Radiother Oncol 1996;41:67-72. [PubMed]
- Magrini SM, Papi MG, Marletta F, et al. Chordoma-natural history, treatment and prognosis. The Florence Radiotherapy Department experience (1956-1990) and a critical review of the literature. Acta Oncol 1992;31:847-51. [PubMed]
- Romero J, Cardenes H, la Torre A, et al. Chordoma: results of radiation therapy in eighteen patients. Radiother Oncol 1993;29:27-32. [PubMed]
- Fuller DB, Bloom JG. Radiotherapy for chordoma. Int J Radiat Oncol Biol Phys 1988;15:331-9. [PubMed]
- Ares C, Hug EB, Lomax AJ, et al. Effectiveness and safety of spot scanning proton radiation therapy for chordomas and chondrosarcomas of the skull base: first long-term report. Int J Radiat Oncol Biol Phys 2009;75:1111-8. [PubMed]
- Igaki H, Tokuuye K, Okumura T, et al. Clinical results of proton beam therapy for skull base chordoma. Int J Radiat Oncol Biol Phys 2004;60:1120-6. [PubMed]
- Munzenrider JE, Liebsch NJ. Proton therapy for tumors of the skull base. Strahlenther Onkol 1999;175:57-63. [PubMed]
- Yasuda M, Bresson D, Chibbaro S, et al. Chordomas of the skull base and cervical spine: clinical outcomes associated with a multimodal surgical resection combined with proton-beam radiation in 40 patients. Neurosurg Rev 2012;35:171-82; discussion 182-3. [PubMed]
- Schulz-Ertner D, Karger CP, Feuerhake A, et al. Effectiveness of carbon ion radiotherapy in the treatment of skull-base chordomas. Int J Radiat Oncol Biol Phys 2007;68:449-57. [PubMed]
- Stüer C, Schramm J, Schaller C. Skull base chordomas: management and results. Neurol Med Chir (Tokyo) 2006;46:118-24; discussion 124-5. [PubMed]
- Takahashi S, Kawase T, Yoshida K, et al. Skull base chordomas: efficacy of surgery followed by carbon ion radiotherapy. Acta Neurochir (Wien) 2009;151:759-69. [PubMed]
- Fuji H, Nakasu Y, Ishida Y, et al. Feasibility of proton beam therapy for chordoma and chondrosarcoma of the skull base. Skull Base 2011;21:201-6. [PubMed]
- Mizoe JE, Hasegawa A, Takagi R, et al. Carbon ion radiotherapy for skull base chordoma. Skull Base 2009;19:219-24. [PubMed]
- Foweraker KL, Burton KE, Maynard SE, et al. High-dose radiotherapy in the management of chordoma and chondrosarcoma of the skull base and cervical spine: Part 1--Clinical outcomes. Clin Oncol (R Coll Radiol) 2007;19:509-16. [PubMed]
- Di Maio S, Temkin N, Ramanathan D, et al. Current comprehensive management of cranial base chordomas: 10-year meta-analysis of observational studies. J Neurosurg 2011;115:1094-105. [PubMed]
- Cheung M, Chan AS, Law SC, et al. Cognitive function of patients with nasopharyngeal carcinoma with and without temporal lobe radionecrosis. Arch Neurol 2000;57:1347-52. [PubMed]
- Baxendale S. Amnesia in temporal lobectomy patients: historical perspective and review. Seizure 1998;7:15-24. [PubMed]
- Pehlivan B, Ares C, Lomax AJ, et al. Temporal lobe toxicity analysis after proton radiation therapy for skull base tumors. Int J Radiat Oncol Biol Phys 2012;83:1432-40. [PubMed]
- Santoni R, Liebsch N, Finkelstein DM, et al. Temporal lobe (TL) damage following surgery and high-dose photon and proton irradiation in 96 patients affected by chordomas and chondrosarcomas of the base of the skull. Int J Radiat Oncol Biol Phys 1998;41:59-68. [PubMed]
- Condra KS, Buatti JM, Mendenhall WM, et al. Benign meningiomas: primary treatment selection affects survival. Int J Radiat Oncol Biol Phys 1997;39:427-36. [PubMed]
- Mirimanoff RO, Dosoretz DE, Linggood RM, et al. Meningioma: analysis of recurrence and progression following neurosurgical resection. J Neurosurg 1985;62:18-24. [PubMed]
- Barbaro NM, Gutin PH, Wilson CB, et al. Radiation therapy in the treatment of partially resected meningiomas. Neurosurgery 1987;20:525-8. [PubMed]
- Austin-Seymour M, Urie M, Munzenrider J, et al. Considerations in fractionated proton radiation therapy: clinical potential and results. Radiother Oncol 1990;17:29-35. [PubMed]
- Taylor BW Jr, Marcus RB Jr, Friedman WA, et al. The meningioma controversy: postoperative radiation therapy. Int J Radiat Oncol Biol Phys 1988;15:299-304. [PubMed]
- Glaholm J, Bloom HJ, Crow JH. The role of radiotherapy in the management of intracranial meningiomas: the Royal Marsden Hospital experience with 186 patients. Int J Radiat Oncol Biol Phys 1990;18:755-61. [PubMed]
- Dziuk TW, Woo S, Butler EB, et al. Malignant meningioma: an indication for initial aggressive surgery and adjuvant radiotherapy. J Neurooncol 1998;37:177-88. [PubMed]
- Milosevic MF, Frost PJ, Laperriere NJ, et al. Radiotherapy for atypical or malignant intracranial meningioma. Int J Radiat Oncol Biol Phys 1996;34:817-22. [PubMed]
- Goldsmith BJ, Wara WM, Wilson CB, et al. Postoperative irradiation for subtotally resected meningiomas. A retrospective analysis of 140 patients treated from 1967 to 1990. J Neurosurg 1994;80:195-201. [PubMed]
- Mair R, Morris K, Scott I, et al. Radiotherapy for atypical meningiomas. J Neurosurg 2011;115:811-9. [PubMed]
- Pearson BE, Markert JM, Fisher WS, et al. Hitting a moving target: evolution of a treatment paradigm for atypical meningiomas amid changing diagnostic criteria. Neurosurg Focus 2008;24:E3 [PubMed]
- Smith SJ, Boddu S, Macarthur DC. Atypical meningiomas: WHO moved the goalposts? Br J Neurosurg 2007;21:588-92. [PubMed]
- Komotar RJ, Iorgulescu JB, Raper DM, et al. The role of radiotherapy following gross-total resection of atypical meningiomas. J Neurosurg 2012;117:679-86. [PubMed]
- Boskos C, Feuvret L, Noel G, et al. Combined proton and photon conformal radiotherapy for intracranial atypical and malignant meningioma. Int J Radiat Oncol Biol Phys 2009;75:399-406. [PubMed]
- Chan AW, Bernstein KD, Adams JA, et al. Dose escalation with proton radiation therapy for high-grade meningiomas. Technol Cancer Res Treat 2012;11:607-14. [PubMed]
- Hug EB, Devries A, Thornton AF, et al. Management of atypical and malignant meningiomas: role of high-dose, 3D-conformal radiation therapy. J Neurooncol 2000;48:151-60. [PubMed]
- Compter I, Zaugg K, Houben RM, et al. High symptom improvement and local tumor control using stereotactic radiotherapy when given early after diagnosis of meningioma. A multicentre study. Strahlenther Onkol 2012;188:887-93. [PubMed]
- Henzel M, Gross MW, Hamm K, et al. Significant tumor volume reduction of meningiomas after stereotactic radiotherapy: results of a prospective multicenter study. Neurosurgery 2006;59:1188-94; discussion 1194. [PubMed]
- Selch MT, Ahn E, Laskari A, et al. Stereotactic radiotherapy for treatment of cavernous sinus meningiomas. Int J Radiat Oncol Biol Phys 2004;59:101-11. [PubMed]
- Liu JK, Forman S, Hershewe GL, et al. Optic nerve sheath meningiomas: visual improvement after stereotactic radiotherapy. Neurosurgery 2002;50:950-5; discussion 955-7. [PubMed]
- Kano H, Takahashi JA, Katsuki T, et al. Stereotactic radiosurgery for atypical and anaplastic meningiomas. J Neurooncol 2007;84:41-7. [PubMed]
- Slater JD, Loredo LN, Chung A, et al. Fractionated proton radiotherapy for benign cavernous sinus meningiomas. Int J Radiat Oncol Biol Phys 2012;83:e633-7. [PubMed]
- Weber DC, Lomax AJ, Rutz HP, et al. Spot-scanning proton radiation therapy for recurrent, residual or untreated intracranial meningiomas. Radiother Oncol 2004;71:251-8. [PubMed]
- Noël G, Bollet MA, Calugaru V, et al. Functional outcome of patients with benign meningioma treated by 3D conformal irradiation with a combination of photons and protons. Int J Radiat Oncol Biol Phys 2005;62:1412-22. [PubMed]
- Vernimmen FJ, Harris JK, Wilson JA, et al. Stereotactic proton beam therapy of skull base meningiomas. Int J Radiat Oncol Biol Phys 2001;49:99-105. [PubMed]
- Wenkel E, Thornton AF, Finkelstein D, et al. Benign meningioma: partially resected, biopsied, and recurrent intracranial tumors treated with combined proton and photon radiotherapy. Int J Radiat Oncol Biol Phys 2000;48:1363-70. [PubMed]
- Halasz LM, Bussière MR, Dennis ER, et al. Proton stereotactic radiosurgery for the treatment of benign meningiomas. Int J Radiat Oncol Biol Phys 2011;81:1428-35. [PubMed]
- Maclean J, Fersht N, Bremner F, et al. Meningioma causing visual impairment: outcomes and toxicity after intensity modulated radiation therapy. Int J Radiat Oncol Biol Phys 2013;85:e179-86. [PubMed]
- Kosaki K, Ecker S, Habermehl D, et al. Comparison of intensity modulated radiotherapy (IMRT) with intensity modulated particle therapy (IMPT) using fixed beams or an ion gantry for the treatment of patients with skull base meningiomas. Radiat Oncol 2012;7:44. [PubMed]