An update on immunotherapies including chimeric antigen receptor T-cells therapy and stem cell transplantation in chronic lymphocytic leukemia
Introduction
Since 1893, when William B. Coley published his work on using a streptococcus toxin to stimulate an immune response against a patient’s tumor, investigators have been seeking ways to utilize the immune system in the fight against cancer (1). Over 100 years later, engaging and activating the immune system has become a vital part of treating cancer. Monoclonal antibodies (MoAbs), immune checkpoint inhibitors, allogeneic stem cell transplantation, chimeric antigen receptor T-cells (CAR-T), bispecific antibodies and immunomodulatory agents, among others, are a growing part in the arsenal against hematologic malignancies.
Chronic lymphocytic leukemia (CLL) is the most common leukemia in adults, and the treatment landscape has been changing rapidly. Therapeutics has evolved from steroids as the only known option in the 1950s, to chemoimmunotherapy combinations, to the kinase inhibitors, novel antibodies and cellular therapies of today. Alkylating agents, such as chlorambucil and cyclophosphamide, were added to treatment regimens in the 1970s, and in the 1990s, fludarabine became part of the standard treatment regimens (2). The monoclonal antibody rituximab has been a part of standard treatment regimens since 2005 (3,4), and second generation, glycoengineered MoAbs have now been approved (5). Novel agents such as idelalisib, ibrutinib, and venetoclax, which target the B-cell receptor (BCR) and B-cell lymphoma 2 (BCL2) pathways, were added to the therapeutic options in the past few years (6-9).
Despite all of the recent advances, the only potentially curative therapy available is an allogeneic stem cell transplant (SCT) (10). The current understanding is that the graft versus leukemia (GVL) effect can permanently eradicate the CLL clone (11). Unfortunately, the exceedingly high rates of morbidity and mortality associated with this procedure, in combination with an ever expanding list of available novel therapies, has limited the use of allogeneic SCT to a few, highly selected patients (12).
Genetically engineered T-cells, harnessing the power of the T-cell vs. leukemia effect, have been tested successfully in a limited number of CLL patients (12-14). Additionally, other immunotherapies such as checkpoint blocking agents, are now being tested in CLL patients (15). This review will discuss immune directed therapies available, and in clinical trials, for CLL.
Biology and targets
CLL is a disease of both B and T-cell immune dysregulation (16-20). T-cells, while not malignant in CLL, are noted to be less responsive to mitogenic stimuli (16). This may be in part due to downregulation by the programmed death-1/programmed death ligand-1 (PD-1/PD-L1) axis (16). Not only are the T-cells in the microenvironment unable to respond to the aberrant CLL cells, but there is evidence that through interleukin 21 (IL-21) and cluster differentiation 40 ligand (CD40L) signaling, certain T-cells may promote CLL proliferation (21). The pro-survival protein BCL2 is also highly expressed in CLL, due in part to the stimulation from CD40 (22). All combined, these lead to an incompetent immune system and immortal cancer cells.
As with the associated T-cells, other aspects of the microenvironment are also an important part of the biology of the disease. Negative prognostic markers in CLL include T-cell receptor (TCR) zeta-chain associated protein kinase 70 kDa (ZAP-70) and cluster differentiation 38 (CD38) positivity. These are both involved in the signaling pathways regulating migration and survival of leukemic cells in the microenvironment (23,24). Deaglio et al. showed that CD38 and ZAP-70 positivity is associated with increased migration in response to the stromal derived factor 1α (SDF-1α), also known as C-X-C motif chemokine ligand 12 (CXCL12) (25). SDF-1α is also the sole ligand for C-X-C chemokine receptor type 4 (CXCR4), which is highly expressed on malignant cells (26).
The CXCR4/CXCL12 chemokine system is, as noted, an important microenvironment adhesion and migration signal. Blocking the CXCR4/CXCL12 ligation has widely been used in peripheral blood stem cell collection (27), and has also been studied as a possible chemotherapy sensitizer (28,29). Idelalisib and ibrutinib, which are approved for use in CLL, have been found to down regulate CXCR4 (30), which may also make them chemotherapy and immunotherapy sensitizers (29-32).
With mounting evidence that the microenvironment is utilized by the CLL clone to avoid immune surveillance and cytotoxic therapies (33), agents directed at both the microenvironment and immune checkpoint blockade may be important therapeutic targets in CLL.
Checkpoint inhibition in CLL
Immune checkpoint inhibition targeting cytotoxic T-lymphocyte-associated protein 4 (CTLA-4), PD-1 and PD-L1, have been successful in treating melanoma (34), non-small cell lung cancer (35), and other malignancies (36,37). The success in hematologic malignancies has been promising thus far as single agents in certain diseases (38,39). In CLL though, the data with PD-1/PD-L1 axis blockade is limited.
Ansell et al. showed an 87% overall response rate (ORR), and 17% complete response (CR) in patients with refractory Hodgkin’s disease treated with the anti PD-1 monoclonal antibody nivolumab (40). In multiple myeloma, on the other hand, early studies with single agent PD-1 blockade showed primarily stable disease (SD) (38). In a phase Ib study looking across multiple hematologic malignancies with 27 patients with multiple myeloma, there was 1 (4%) CR and 17 (63%) with SD (38). Early results in myeloma from the KEYNOTE-23 study and a phase II study by Badros et al., demonstrated that when combined with other immune modulating agents, there is a synergistic effect. The KEYNOTE-23 trial showed a 76% response rate when pembrolizumab was combined with lenalidomide in a population previously refractory to lenalidomide (41). Badros et al. reported a 50% objective response rate in a multiple refractory population when pembrolizumab was combined with pomalidomide (42).
In CLL, in vitro and mouse model experiments indicate that the T-cells express PD-1 as a sign of “T-cell exhaustion” and immune dysfunction as mentioned above (18,43). This was found to be reversible with PD-1 blockade (43). Despite this, there is very little in vivo data on checkpoint blockade in CLL. Ding et al. reported interim phase II data on 16 patients with relapsed/refractory CLL or Richter’s transformation who were treated with pembrolizumab monotherapy (15). Of the five patients with Richter’s transformation, four patients responded, including one CR, while the two patients with CLL had SD (15).
There are several ongoing trials combining PD-1 blockade with other agents. The Mayo Clinic and National Cancer Institute (NCI) are conducting a trial (ClinicalTrials.gov identifier NCT02332980) which combines pembrolizumab with either idelalisib or ibrutinib in patients with CLL. Bristol-Myers Squibb is sponsoring a phase I/II trial (ClinicalTrials.gov identifier NCT02061761) combining BMS-986016, an antibody to lymphocyte activation gene 3 (LAG3), with nivolumab in patients with advanced hematologic malignancies, including CLL. A phase I/II trial (ClinicalTrials.gov identifier NCT02535286) at the University of Pennsylvania combining pembrolizumab with ublituximab (a novel, glycoengineered anti-CD20 monoclonal antibody) and TGR-1202 [an oral, once a day phosphoinositide 3-kinase (PI3Kδ) inhibitor] is also ongoing. Other trials combining PD-1 and PD-L1 with targeted agents are listed in Table 1.
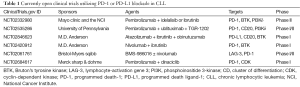
Full table
Allogeneic stem cell transplantation in CLL
SCT is the forerunner of all immuno-cellular therapies, and has been utilized in the treatment of several hematologic malignancies (44,45). Because of the advanced age of most patients with CLL (median age at presentation is 70 years old) (46), and the presence of comorbid conditions, donor derived stem cell transplantation has historically played a limited role in the management of patients with CLL (12). With the approvals of agents such as BCR signal transduction inhibitors and BCL2 inhibitory agents, the role of SCT is likely to be further diminished (12). However, the SCT literature stands as powerful proof that T-cell mediated therapies can be administered to patients with CLL with curative intent (47-50).
The Society for European Bone Marrow Transplantation (EBMT) released consensus guidelines for allogeneic transplant in CLL in 2007 (51). The consensus opinion was that allogeneic SCT is a procedure with evidence-based efficacy in poor-risk CLL. They indicated that it is a reasonable treatment option for younger patients with non-response or early relapse (within 12 months) after purine analogs, or relapse within 24 months after having achieved a response with purine-analog-based combination therapy or autologous transplantation, and in patients with p53 abnormalities requiring treatment. They went on to state, “…whenever possible, allo-SCT should be performed within disease-specific prospective clinical protocols in order to continuously refine transplant indications according to new developments in risk assessment and treatment of CLL” (51).
In September of 2016, the Guidelines Committee of the American Society for Blood and Marrow Transplantation released updated recommendations for when to utilize allogeneic stem cell transplantation in CLL (10). The guidelines noted that there was a dearth of randomized clinical trials on which to base the recommendations (10). The 2016 guidelines recommended against HCT as front line consolidation, but did recommend transplantation in the setting of disease refractory to second line therapy, including BCR targeted therapy (10). If after BCR directed therapy, the disease responds to BCL-2 inhibition, transplant is still recommended (10). Transplant is also recommended in the setting of Richter’s transformation after response to anthracycline based therapy (10). Autologous transplantation is not considered an effective part of therapy in CLL and was not recommended (10,52).
For CLL, myeloablative conditioning (MAC) carries an unacceptably high mortality rate (11,53). Peres et al. reported a 100-day mortality of 27% with full intensity conditioning versus only 14% with reduced intensity conditioning (RIC) regimens (53). Also of note the relapse rate with a RIC regimen remains comparable to using a MAC regimen (11). Given this, the more common approach in the case of allogeneic transplantation for CLL is to use various RIC or non-myeloablative regimens (12).
Further demonstrating the value of SCT, Dreger et al. reported on phase II data in which 90 CLL patients treated with allogeneic HCT were followed for a median of 46 months. The 4-year non-relapse mortality (NRM), event-free survival (EFS), and overall survival (OS) were 23%, 42%, and 65%, respectively (48). At 1 year, 52 patients were evaluable for minimal residual disease (MRD). Of these 27 (52%) were MRD negative, indicating that the immune response may be able to clear the disease (48). Dreger et al. also published 6-year follow-up for the study in 2013. In that analysis they noted a 60% OS that was independent of the disease as stratified by risk (54).
Autologous and allogeneic SCT in the setting of Richter’s transformation (RT) was recently reviewed by Ayers and Mato (55). Based on the available data (56-58), they concluded that stem cell transplantation was an efficacious option in this setting (55). The trials they cited showed promising results for both autologous and allogeneic transplant in the setting of RT, including improved survival (55). This is in contrast to CLL where autologous SCT is not considered standard practice (52,59).
Tsimberidou et al. described 148 patients with biopsy proven RT, of which 20 underwent transplantation after a CR, CR unconfirmed, or partial response (PR) (58). The cumulative survival at 3 years was 75% among patients undergoing allogeneic SCT, compared with 27% among patients without SCT after therapy, and 21% for patients undergoing either autologous or allogeneic SCT as salvage therapy (58).
Rozovski et al. looked at patients with CLL and Richter’s transformation who had been treated with allogeneic transplant. Sixteen of 52 patients (30%) transplanted for CLL developed RT after transplant. The opposite effect also occurred with 4 of 20 patients (20%) who had RT before transplantation then relapsed with CLL, but no signs of aggressive lymphoma (57). While this may indicate various sensitivities to chemotherapy, it was proposed to be a variable GVL effect between CLL and RT.
Evidence of a GVL effect in CLL is also supported by the donor lymphocyte infusion (DLI) literature, where responses to DLI have been noted. As an example, Gribben et al. reported on seven CLL patients who relapsed after allogeneic transplantation, six patients responded when treated with DLI. Historically, the response rate to DLI in CLL is estimated to be 47% (11). Emerging data for the use of ibrutinib in the setting of progression post allogeneic SCT also looks promising for inducing the GVL effect (12), and may be used in conjunction with DLI or other cellular therapies in the future.
Thus far, no manipulation of the allogeneic graft has been able to completely separate the GVL effect from graft versus host disease (GVHD) or other treatment related toxicities. This quest has inspired a new field of autologous graft manipulations in the form of CAR-T cells and cytotoxic T-lymphocytes expanded against tumor antigens (60).
Adoptive cellular therapies (ACT)
ACT utilize techniques where populations of cells are collected, expanded, and then reinfused. ACT covers less specific therapies such as tumor infiltrating lymphocytes (TIL), which are expanded un-manipulated cells, as well as therapies in which the cells are specifically expanded against tumor antigens, or are genetically modified, as in the case of CAR T-cell therapies.
Antigen specific T-cells
Hardy et al., in 2012, published a trial of 8 patients with CLL who had relapsed after allogeneic transplantation. Withdrawal of immune suppression and DLI had been done prior, without success. All patients had lesions harvested and TILs expanded with CD3/CD28 coated beads. Of the eight patients treated, there were two transient responses and two mixed responses (61). Despite prior allogeneic stem cell transplantation, GVHD was not observed with TIL therapy (61). The results are suggestive of a population of cells in the tumor microenvironment with specificity to tumor antigens.
Adoptive immunotherapy using autologous CD3/CD28-costimulated T-cells (ACTC) expanded ex vivo was also used in a trial by Schuster et al. examining immune reconstitution following fludarabine-based therapy (62). In this trial, 34 patients with CLL were enrolled; 18 previously untreated patients and 16 patients with relapsed or refractory disease. Ten patients did not receive ACTC infusion due to various complications prior to the infusion. Two patients had progressive disease less than 90 days after ACTC and one patient was lost to follow-up. For the remaining patients, CD4 and CD8 reconstitution was more rapid than in historical controls. ACTC production was found to be feasible, well tolerated, and resulted in acceleration of CD4+ and CD8+ cell recovery after fludarabine-based chemotherapy compared to historical controls. The data examining PFS compared to historical controls is not yet mature. The trial is ongoing (ClinicalTrials.gov identifier, NCT01013441) in long-term follow up.
CAR T-cells
The quest for a transplant with maximal GVL without GVHD lead to development of autologous T-cells engineered to induce a GVL effect. CAR T-cells are genetically modified to target malignant cells. One process for creating CAR T-cells starts with collecting the cells by steady-state leukapheresis (12). The cells then may undergo subset selection. After this, the cells undergo gene transfer with the chimeric antigen receptor. The most common method for doing this is via a retroviral vector, such as a lentivirus, but other methods include electroporation and transposons (12). The cells are then expanded through short-term culture and, once completed, cells are reinfused into the host. Overall, the process takes 12–14 days (12).
In CLL, the most studied target utilizing CAR T-cell technologies is cluster of differentiation (CD) 19 (12,13). This CAR has a CD19 antibody antigen recognition domain and an intracellular domain with the CD3-zeta chain bound to CD137 (41BB) in single chimeric protein (63). The 41BB domain acts as a costimulatory signal when the CAR is engaged (63). Activation of this signal is thought to enhance the in vivo expansion of the T-cells (63).
The CD19 antigen was selected as the ideal target in CLL because it is highly conserved on the CLL cancer cells and non-malignant B-cells throughout their development. It is not thought to be found on hematopoietic stem cells, or other cells in the body. While B-cell aplasia, which often is found after treatment with CD19 CAR T-cells, leaves patients immunosuppressed due to hypogammaglobulinemia, this can for the most part be overcome with replacement of pooled antibodies, which is unique to this target and malignancy (13,63).
Successful use of the CART19 cells (CTL019) in 3 patients with CLL was first described in 2011 by Porter et al. at the University of Pennsylvania (63). In 2015, Porter et al. reported expanded results from the initial CLL trial using the CTL019 CAR-modified T-cells.(13) The ORR in these heavily pretreated, relapsed and/or refractory patients was 8 of 14 (57%), with 4 complete remissions and four partial remissions (13). As noted previously, there was a correlation between the in vivo expansion of the CAR T-cells and clinical responses. In the first two patients achieving CR, the CAR T-cells persisted and remained functional beyond 4 years. No patient who achieved a CR relapsed in that time period (13). As of July 2015, over 45 patients with CLL were treated at the University of Pennsylvania with CTL019 T- cells (12).
At the NCI, eight patients with CLL, across two trials, were reported receiving autologous CD19 CAR T-cells (64,65). Of these, seven responded; four of which were in CR at the last evaluation. The CAR used in these trials differed from CTL019 in that the NCI anti-CD19 CAR contained the variable regions of a murine anti–human CD19 antibody, a portion of the CD28 molecule as the costimulatory domain, and the signaling domain of the CD3 molecule.
The group at the NCI also reported on 20 patients progressing after allogenic SCT who were treated with allogeneic CD19 CAR T-cells made from the original graft source. Five of the patients had CLL, and of these, there was one CR, one PR, one SD, and two PD (66). No patient in this series experienced GVHD related to CAR T infusion. The CAR T technologies developed at the NCI are now being further developed by Kite Pharma as KTE-C19 (67), and are in trials in combination with checkpoint inhibitors for aggressive NHL (ClinicalTrials.gov identifier NCT02926833). There are plans to start trials with CLL in the near future (http://kitepharma.com/pipeline/).
Brentjens et al. at Memorial Sloan Kettering (MSK) have reported on their experience in treating patients with CLL with CAR T-cells (68). They reported on 10 patients, 8 of which had CLL treated with 19-28z T-cells (CAR T-cells with CD19 antibody domain and an intracytoplasmic CD3 domain with a CD28 costimulatory domain) (68). Of the eight patients with CLL, one was not evaluable, one had an objective response then SD, two had SD, one had progressive disease, three had no response. There were no CRs in this series (68).
One of the major issues encountered in patients treated with CAR T therapy is cytokine release syndrome (CRS) (13,63). Patients with this syndrome can experience fevers, cardiac dysfunction including stress cardiomyopathy, adult respiratory distress syndrome, neurologic toxicity including delirium and seizures, renal failure, hepatic failure, and disseminated intravascular coagulation (69). Macrophage activation syndrome/hemophagocytic lymphohistiocytosis has overlap with CRS symptomatology and has been reported in the setting of CRS (70). Management strategies include supportive care for low grade CRS to steroids and anti-IL6 antibody tocilizumab for higher grades of the syndrome (70).
Given the pervasiveness and accessibility of the CD19 target on the surface of the malignant cells made it an ideal target. CD19 is not however restricted to malignant B-cells, and thus successful patients may experience B-cell aplasia as long as the CAR T-cells persist. Patients become agammaglobulinemic and reliant on monthly infusions of intravenous immunoglobulin (IVIG) for immune protection (63).
Other targets such as the immunoglobulin M (IgM) Fc receptor (FcµR) are being studied (71). In vitro this appears to be more specific for the malignant B-cells, sparing many of the normal B cells (71). Another ongoing trial (ClinicalTrials.gov identifier, NCT02194374) being carried out at MD Anderson Cancer Center, uses CAR T-cells targeted towards receptor tyrosine kinase-like orphan receptor-1 (ROR1). This target is more specifically expressed on populations of malignant B-cells as compared to the normal immune system (72).
Di Stasi et al. devised a CAR T “safety switch” for managing GVHD which could be applied to other CAR T therapies (73). In their study, they created an inducible T-cell safety switch that is based on the fusion of human caspase 9 to a modified human FK-binding protein. When exposed to a synthetic dimerizing drug, the inducible caspase 9 (iCasp9) is activated and leads to the rapid death of cells expressing this construct (73). Five patients received the genetically modified T-cells and four were treated with the dimerizing drug for their GVHD. After a single dose, more than 90% of the modified T-cells were eliminated within 30 minutes, and the GVHD resolved without recurrence (73).
Ma et al. described a method for controlling CARs with soluble intermediary “switch” molecules (74). In their study, they created an anti-fluorescein isothiocyanate (FITC) CAR T-cells and anti-CD19 AB-FITC, as well as an anti-CD22 AB-FITC (74). The potential benefits of this approach are the ability to turn off or attenuate the effects of the CAR T-cell in the setting of toxicity, as well as to redirect CAR T-cells in the setting of immune escape, as can happen in B-ALL (74). This strategy also offers the ability to target multiple antigens at once by infusing multiple switch molecules, such as both an anti-CD19 AB-FITC and an anti-CD22 AB-FITC. With both intermediary switch molecules present the in vivo population of anti-FITC CAR T-cells would be activated to both targets (74). This method is being further developed at the California Institute for Biomedical Research with different CAR-Ts and intermediary molecules (75).
The Bruton’s tyrosine kinase (BTK) inhibitor ibrutinib is now being studied following allogeneic stem cell transplantation and CAR T therapies. Ryan et al. reported successful treatment of five patients with ibrutinib following allogeneic transplantation. One of the patients discontinued therapy and remained MRD negative. The patient’s GVHD also resolved, indicating donor immune modulation towards GVL and away from GVHD (76). This may be due in part to the disruption of the bone marrow microenvironment, where CLL tends to be protected from cellular and cytotoxic therapies (13,31), and/or downregulation of PD-1 expression on the T-cells (77).
Fraietta et al. reported a similar effect in in vitro and murine model of CAR T-cells treated following administration of ibrutinib, where enhanced engraftment of the infused cells was observed (77). Five cycles of ibrutinib therapy improved the expansion of CD19-directed CAR T-cells and decreased the expression of PD-1 on T-cells and of membrane glycoprotein MRC OX-2 (also known as MOX2 or CD200), on B-CLL cells (77). High CD200 expression has been associated with poor outcomes (78). A trial (ClinicalTrials.gov identifier NCT02640209) examining the combination of autologous anti-CD19 CAR-T and ibrutinib in patients with relapsed or refractory CLL or small lymphocytic lymphoma (SLL) is ongoing.
Conclusions
Immunotherapy in CLL is rapidly expanding, and the landscape and guidelines are adjusting to the new landscape. Checkpoint inhibition will likely soon find its way into the algorithm for treatment as part of a combination with immunomodulatory medications, and CART is making strides towards becoming more widely available. Each of these therapies has proven to be effective in certain populations of patients.
The goal moving forward will entail being able to identify which patients will respond best to which agents, and in what sequence, as well has how best to combine the therapies. The intracellular signaling in the malignant cells through BCL2 and other pathways promotes growth and survival. Upregulation of these signals appears to primarily come from the tumor environment, including T-cells and other mononuclear cells. Being able to control these signals and disrupt the microenvironment may ultimately expose the malignant clone in immunological sanctuary sites to the immune system and enhance immune surveillance abilities to eradicate malignancy. Ultimately, targeting the microenvironment and pathways in the malignant cells will likely be combined with cellular therapies to enhance their effectiveness. With these advances, new treatment approaches for CLL and RT with curative intention may be on the horizon.
Acknowledgments
The authors would like to thank Lisa Gashonia for her contribution to this manuscript.
Funding: None.
Footnote
Provenance and Peer Review: This article was commissioned by the Guest Editor (Marin Feldman Xavier) for the series “Advances on Clinical Immunotherapy” published in Translational Cancer Research. The article has undergone external peer review.
Conflicts of Interest: Both authors have completed the ICMJE uniform disclosure form (available at http://dx.doi.org/10.21037/tcr.2017.01.04). The series “Advances on Clinical Immunotherapy” was commissioned by the editorial office without any funding or sponsorship. The authors have no other conflicts of interest to declare.
Ethical Statement: The authors are accountable for all aspects of the work in ensuring that questions related to the accuracy or integrity of any part of the work are appropriately investigated and resolved.
Open Access Statement: This is an Open Access article distributed in accordance with the Creative Commons Attribution-NonCommercial-NoDerivs 4.0 International License (CC BY-NC-ND 4.0), which permits the non-commercial replication and distribution of the article with the strict proviso that no changes or edits are made and the original work is properly cited (including links to both the formal publication through the relevant DOI and the license). See: https://creativecommons.org/licenses/by-nc-nd/4.0/.
References
- McCarthy EF. The toxins of William B. Coley and the treatment of bone and soft-tissue sarcomas. Iowa Orthop J 2006;26:154-8. [PubMed]
- Keating MJ, O'Brien S, Lerner S, et al. Long-term follow-up of patients with chronic lymphocytic leukemia (CLL) receiving fludarabine regimens as initial therapy. Blood 1998;92:1165-71. [PubMed]
- Keating MJ. Early Results of a Chemoimmunotherapy Regimen of Fludarabine, Cyclophosphamide, and Rituximab As Initial Therapy for Chronic Lymphocytic Leukemia. J Clin Oncol 2005;23:4079-88. [Crossref] [PubMed]
- Stilgenbauer S, Schnaiter A, Paschka P, et al. Gene mutations and treatment outcome in chronic lymphocytic leukemia: results from the CLL8 trial. Blood 2014;123:3247-54. [Crossref] [PubMed]
- Goede V, Fischer K, Busch R, et al. Obinutuzumab plus Chlorambucil in Patients with CLL and Coexisting Conditions. N Engl J Med 2014;370:1101-10. [Crossref] [PubMed]
- Byrd JC, Furman RR, Coutre SE, et al. Targeting BTK with Ibrutinib in Relapsed Chronic Lymphocytic Leukemia. N Engl J Med 2013;369:32-42. [Crossref] [PubMed]
- Byrd JC, Harrington B, O'Brien S, et al. Acalabrutinib (ACP-196) in Relapsed Chronic Lymphocytic Leukemia. N Engl J Med 2016;374:323-32. [Crossref] [PubMed]
- Roberts AW, Davids MS, Pagel JM, et al. Targeting BCL2 with Venetoclax in Relapsed Chronic Lymphocytic Leukemia. N Engl J Med 2016;374:311-22. [Crossref] [PubMed]
- Furman RR, Sharman JP, Coutre SE, et al. Idelalisib and Rituximab in Relapsed Chronic Lymphocytic Leukemia. N Engl J Med 2014;370:997-1007. [Crossref] [PubMed]
- Kharfan-Dabaja MA, Kumar A, Hamadani M, et al. Clinical Practice Recommendations for Use of Allogeneic Hematopoietic Cell Transplantation in Chronic Lymphocytic Leukemia on Behalf of the Guidelines Committee of the American Society for Blood and Marrow Transplantation. Biol Blood Marrow Transplant 2016;22:2117-25. [Crossref] [PubMed]
- Ben-Bassat I, Raanani P, Gale RP. Graft-versus-leukemia in chronic lymphocytic leukemia. Bone Marrow Transplant 2007;39:441-6. [Crossref] [PubMed]
- Mato A, Porter DL. A drive through cellular therapy for CLL in 2015: allogeneic cell transplantation and CARs. Blood 2015;126:478-85. [Crossref] [PubMed]
- Porter DL, Hwang WT, Frey NV, et al. Chimeric antigen receptor T cells persist and induce sustained remissions in relapsed refractory chronic lymphocytic leukemia. Sci Transl Med 2015;7:303ra139-9. [Crossref] [PubMed]
- Porter DL, Frey NV, Melenhorst JJ, et al. Randomized, Phase II Dose Optimization Study of Chimeric Antigen Receptor Modified T Cells Directed Against CD19 (CTL019) in Patients with Relapsed, Refractory CLL. Blood 2014;124:1982.
- Ding W, Dong H, Call TG, et al. PD-1 Blockade with Pembrolizumab (MK-3475) in Relapsed/Refractory CLL Including Richter Transformation: An Early Efficacy Report from a Phase 2 Trial (MC1485). Blood 2015;126:834.
- Brusa D, Serra S, Coscia M, et al. The PD-1/PD-L1 axis contributes to T-cell dysfunction in chronic lymphocytic leukemia. Haematologica 2013;98:953-63. [Crossref] [PubMed]
- Wu J, Xu X, Lee EJ, et al. Phenotypic alteration of CD8+ T cells in chronic lymphocytic leukemia is associated with epigenetic reprogramming. Oncotarget 2016;7:40558-70. [PubMed]
- Riches JC, Davies JK, McClanahan F, et al. T cells from CLL patients exhibit features of T-cell exhaustion but retain capacity for cytokine production. Blood 2013;121:1612-21. [Crossref] [PubMed]
- Nicholas NS, Apollonio B, Ramsay AG. Tumor microenvironment (TME)-driven immune suppression in B cell malignancy. Biochim Biophys Acta 2016;1863:471-82.
- Urquiza M, Melo-Cardenas J, Aguillon R, et al. Intratumoral Injection of Ad-ISF35 (Chimeric CD154) Breaks Tolerance and Induces Lymphoma Tumor Regression. Hum Gene Ther 2015;26:14-25. [Crossref] [PubMed]
- Pascutti MF, Jak M, Tromp JM, et al. IL-21 and CD40L signals from autologous T cells can induce antigen-independent proliferation of CLL cells. Blood 2013;122:3010-9. [Crossref] [PubMed]
- Vogler M, Butterworth M, Majid A, et al. Concurrent up-regulation of BCL-XL and BCL2A1 induces approximately 1000-fold resistance to ABT-737 in chronic lymphocytic leukemia. Blood 2009;113:4403-13. [Crossref] [PubMed]
- Gribben JG. How I treat CLL up front. Blood 2010;115:187-97. [Crossref] [PubMed]
- Lafarge ST, Johnston JB, Gibson SB, et al. Adhesion of ZAP-70+ chronic lymphocytic leukemia cells to stromal cells is enhanced by cytokines and blocked by inhibitors of the PI3-kinase pathway. Leuk Res 2014;38:109-15. [Crossref] [PubMed]
- Deaglio S, Vaisitti T, Aydin S, et al. CD38 and ZAP-70 are functionally linked and mark CLL cells with high migratory potential. Blood 2007;110:4012-21. [Crossref] [PubMed]
- Kashyap MK, Kumar D, Jones H, et al. Ulocuplumab (BMS-936564 / MDX1338): a fully human anti-CXCR4 antibody induces cell death in chronic lymphocytic leukemia mediated through a reactive oxygen species-dependent pathway. Oncotarget 2016;7:2809-22. [PubMed]
- Kouroukis CT, Varela NP, Bredeson C, et al. Plerixafor for autologous stem-cell mobilization and transplantation for patients in Ontario. Curr Oncol 2016;23:e409-30. [Crossref] [PubMed]
- Uy GL, Rettig MP, Motabi IH, et al. A phase 1/2 study of chemosensitization with the CXCR4 antagonist plerixafor in relapsed or refractory acute myeloid leukemia. Blood 2012;119:3917-24. [Crossref] [PubMed]
- Reinholdt L, Laursen MB, Schmitz A, et al. The CXCR4 antagonist plerixafor enhances the effect of rituximab in diffuse large B-cell lymphoma cell lines. Biomark Res 2016;4:12. [Crossref] [PubMed]
- Chen SS, Chang BY, Chang S, et al. BTK inhibition results in impaired CXCR4 chemokine receptor surface expression, signaling and function in chronic lymphocytic leukemia. Leukemia 2016;30:833-43. [Crossref] [PubMed]
- Chanan-Khan A, Cramer P, Demirkan F, et al. Ibrutinib combined with bendamustine and rituximab compared with placebo, bendamustine, and rituximab for previously treated chronic lymphocytic leukaemia or small lymphocytic lymphoma (HELIOS): a randomised, double-blind, phase 3 study. Lancet Oncol 2016;17:200-11. [Crossref] [PubMed]
- Azab AK, Runnels JM, Pitsillides C, et al. CXCR4 inhibitor AMD3100 disrupts the interaction of multiple myeloma cells with the bone marrow microenvironment and enhances their sensitivity to therapy. Blood 2009;113:4341-51. [Crossref] [PubMed]
- Burger JA. The CLL Cell Microenvironment. In: Malek S. editor. Advances in Chronic Lymphocytic Leukemia. New York, NY: Springer New York, 2013:25-45.
- Larkin J, Chiarion-Sileni V, Gonzalez R, et al. Combined Nivolumab and Ipilimumab or Monotherapy in Untreated Melanoma. N Engl J Med 2015;373:23-34. [Crossref] [PubMed]
- Garon EB, Rizvi NA, Hui R, et al. Pembrolizumab for the Treatment of Non–Small-Cell Lung Cancer. N Engl J Med 2015;372:2018-28. [Crossref] [PubMed]
- Motzer RJ, Escudier B, McDermott DF, et al. Nivolumab versus Everolimus in Advanced Renal-Cell Carcinoma. N Engl J Med 2015;373:1803-13. [Crossref] [PubMed]
- Rosenberg JE, Hoffman-Censits J, Powles T, et al. Atezolizumab in patients with locally advanced and metastatic urothelial carcinoma who have progressed following treatment with platinum-based chemotherapy: a single-arm, multicentre, phase 2 trial. Lancet 2016;387:1909-20. [Crossref] [PubMed]
- Lesokhin AM, Ansell SM, Armand P, et al. Nivolumab in Patients With Relapsed or Refractory Hematologic Malignancy: Preliminary Results of a Phase Ib Study. J Clin Oncol 2016;34:2698-704. [Crossref] [PubMed]
- Armand P. Immune checkpoint blockade in hematologic malignancies. Blood 2015;125:3393-400. [Crossref] [PubMed]
- Ansell SM, Lesokhin AM, Borrello I, et al. PD-1 Blockade with Nivolumab in Relapsed or Refractory Hodgkin's Lymphoma. N Engl J Med 2015;372:311-9. [Crossref] [PubMed]
- Mateos MV, Orlowski RZ, Siegel D, et al. Pembrolizumab in combination with lenalidomide and low-dose dexamethasone for relapsed/refractory multiple myeloma (RRMM): Final efficacy and safety analysis. J Clin Oncol 2016;34:abstr 8010.
- Badros AZ, Kocoglu MH, Ma N, et al. A Phase II Study of Anti PD-1 Antibody Pembrolizumab, Pomalidomide and Dexamethasone in Patients with Relapsed/Refractory Multiple Myeloma (RRMM). Blood 2015;126:506.
- McClanahan F, Riches JC, Miller S, et al. Mechanisms of PD-L1/PD-1–mediated CD8 T-cell dysfunction in the context of aging-related immune defects in the Eµ-TCL1 CLL mouse model. Blood 2015;126:212-21. [Crossref] [PubMed]
- Majhail NS, Farnia SH, Carpenter PA, et al. Indications for Autologous and Allogeneic Hematopoietic Cell Transplantation: Guidelines from the American Society for Blood and Marrow Transplantation. Biol Blood Marrow Transplant 2015;21:1863-9. [Crossref] [PubMed]
- Baron F, Beguin Y. Nonmyeloablative allogeneic hematopoietic stem cell transplantation. J Hematother Stem Cell Res 2002;11:243-63. [Crossref] [PubMed]
- Diehl LF, Karnell LH, Menck HR. The American College of Surgeons Commission on Cancer and the American Cancer Society. The National Cancer Data Base report on age, gender, treatment, and outcomes of patients with chronic lymphocytic leukemia. Cancer 1999;86:2684-92. [Crossref] [PubMed]
- Sorror ML, Storer BE, Sandmaier BM, et al. Five-Year Follow-Up of Patients With Advanced Chronic Lymphocytic Leukemia Treated With Allogeneic Hematopoietic Cell Transplantation After Nonmyeloablative Conditioning. J Clin Oncol 2008;26:4912-20. [Crossref] [PubMed]
- Dreger P, Dohner H, Ritgen M, et al. Allogeneic stem cell transplantation provides durable disease control in poor-risk chronic lymphocytic leukemia: long-term clinical and MRD results of the German CLL Study Group CLL3X trial. Blood 2010;116:2438-47. [Crossref] [PubMed]
- Brown JR, Kim HT, Armand P, et al. Long-term follow-up of reduced-intensity allogeneic stem cell transplantation for chronic lymphocytic leukemia: prognostic model to predict outcome. Leukemia 2013;27:362-9. [Crossref] [PubMed]
- Khouri IF, Bassett R, Poindexter N, et al. Nonmyeloablative allogeneic stem cell transplantation in relapsed/refractory chronic lymphocytic leukemia. Cancer 2011;117:4679-88. [Crossref] [PubMed]
- Dreger P, Corradini P, Kimby E, et al. Indications for allogeneic stem cell transplantation in chronic lymphocytic leukemia: the EBMT transplant consensus. Leukemia 2007;21:12-7. [Crossref] [PubMed]
- Reljic T, Kumar A, Djulbegovic B, et al. High-dose therapy and autologous hematopoietic cell transplantation as front-line consolidation in chronic lymphocytic leukemia: a systematic review. Bone Marrow Transplant 2015;50:1069-74. [Crossref] [PubMed]
- Peres E, Braun T, Krijanovski O, et al. Reduced intensity versus full myeloablative stem cell transplant for advanced CLL. Bone Marrow Transplant 2009;44:579-83. [Crossref] [PubMed]
- Dreger P, Schnaiter A, Zenz T, et al. TP53, SF3B1, and NOTCH1 mutations and outcome of allotransplantation for chronic lymphocytic leukemia: six-year follow-up of the GCLLSG CLL3X trial. Blood 2013;121:3284-8. [Crossref] [PubMed]
- Ayers EC, Mato AR. Richter's Transformation in the Era of Kinase Inhibitor Therapy: A Review. Clin Lymphoma Myeloma Leuk 2017;17:1-6. [PubMed]
- Cwynarski K, van Biezen A, de Wreede L, et al. Autologous and Allogeneic Stem-Cell Transplantation for Transformed Chronic Lymphocytic Leukemia (Richter's Syndrome): A Retrospective Analysis From the Chronic Lymphocytic Leukemia Subcommittee of the Chronic Leukemia Working Party and Lymphoma Working Party of the European Group for Blood and Marrow Transplantation. J Clin Oncol 2012;30:2211-7. [Crossref] [PubMed]
- Rozovski U, Benjamini O, Jain P, et al. Outcomes of Patients With Chronic Lymphocytic Leukemia and Richter's Transformation After Transplantation Failure. J Clin Oncol 2015;33:1557-63. [Crossref] [PubMed]
- Tsimberidou AM, O'Brien S, Khouri I, et al. Clinical outcomes and prognostic factors in patients with Richter's syndrome treated with chemotherapy or chemoimmunotherapy with or without stem-cell transplantation. J Clin Oncol 2006;24:2343-51. [Crossref] [PubMed]
- Michallet M, Dreger P, Sutton L, et al. Autologous hematopoietic stem cell transplantation in chronic lymphocytic leukemia: results of European intergroup randomized trial comparing autografting versus observation. Blood 2011;117:1516-21. [Crossref] [PubMed]
- Gerdemann U, Katari U, Christin AS, et al. Cytotoxic T lymphocytes simultaneously targeting multiple tumor-associated antigens to treat EBV negative lymphoma. Mol Ther 2011;19:2258-68. [Crossref] [PubMed]
- Hardy NM, Fellowes V, Rose JJ, et al. Costimulated tumor-infiltrating lymphocytes are a feasible and safe alternative donor cell therapy for relapse after allogeneic stem cell transplantation. Blood 2012;119:2956-9. [Crossref] [PubMed]
- Schuster SJ, Hosing CM, Shpall EJ, et al. Adoptive Immunotherapy With Autologous CD3/CD28-Costimulated T-Cells After Fludarabine-Based Chemotherapy In Patients With Chronic Lymphocytic Leukemia. Blood 2013;122:2874.
- Porter DL, Levine BL, Kalos M, et al. Chimeric Antigen Receptor–Modified T Cells in Chronic Lymphoid Leukemia. N Engl J Med 2011;365:725-33. [Crossref] [PubMed]
- Kochenderfer JN, Dudley ME, Feldman SA, et al. B-cell depletion and remissions of malignancy along with cytokine-associated toxicity in a clinical trial of anti-CD19 chimeric-antigen-receptor-transduced T cells. Blood 2012;119:2709-20. [Crossref] [PubMed]
- Kochenderfer JN, Dudley ME, Kassim SH, et al. Chemotherapy-Refractory Diffuse Large B-Cell Lymphoma and Indolent B-Cell Malignancies Can Be Effectively Treated With Autologous T Cells Expressing an Anti-CD19 Chimeric Antigen Receptor. J Clin Oncol 2015;33:540-9. [Crossref] [PubMed]
- Brudno JN, Somerville RP, Shi V, et al. Allogeneic T Cells That Express an Anti-CD19 Chimeric Antigen Receptor Induce Remissions of B-Cell Malignancies That Progress After Allogeneic Hematopoietic Stem-Cell Transplantation Without Causing Graft-Versus-Host Disease. J Clin Oncol 2016;34:1112-21. [Crossref] [PubMed]
- Locke FL, Neelapu SS, Bartlett NL, et al. Phase 1 Clinical Results of the ZUMA-1 (KTE-C19-101) Study: A Phase 1-2 Multi-Center Study Evaluating the Safety and Efficacy of Anti-CD19 CAR T Cells (KTE-C19) in Subjects with Refractory Aggressive Non-Hodgkin Lymphoma (NHL). Blood 2015;126:3991.
- Brentjens RJ, Rivière I, Park JH, et al. Safety and persistence of adoptively transferred autologous CD19-targeted T cells in patients with relapsed or chemotherapy refractory B-cell leukemias. Blood 2011;118:4817-28. [Crossref] [PubMed]
- Lee DW, Gardner R, Porter DL, et al. Current concepts in the diagnosis and management of cytokine release syndrome. Blood 2014;124:188-95. [Crossref] [PubMed]
- Fitzgerald JC, Weiss SL, Maude SL, et al. Cytokine Release Syndrome After Chimeric Antigen Receptor T Cell Therapy for Acute Lymphoblastic Leukemia. Crit Care Med 2017;45:e124-e131. [Crossref] [PubMed]
- Faitschuk E, Hombach AA, Frenzel LP, et al. Chimeric antigen receptor T cells targeting Fc µ receptor selectively eliminate CLL cells while sparing healthy B cells. Blood 2016;128:1711-22. [Crossref] [PubMed]
- Deniger DC, Yu J, Huls MH, et al. Sleeping Beauty Transposition of Chimeric Antigen Receptors Targeting Receptor Tyrosine Kinase-Like Orphan Receptor-1 (ROR1) into Diverse Memory T-Cell Populations. PLoS One 2015;10:e0128151 [Crossref] [PubMed]
- Di Stasi A, Tey SK, Dotti G, et al. Inducible Apoptosis as a Safety Switch for Adoptive Cell Therapy. N Engl J Med 2011;365:1673-83. [Crossref] [PubMed]
- Ma JS, Kim JY, Kazane SA, et al. Versatile strategy for controlling the specificity and activity of engineered T cells. Proc Natl Acad Sci USA 2016;113:E450-8. [Crossref] [PubMed]
- Rodgers DT, Mazagova M, Hampton EN, et al. Switch-mediated activation and retargeting of CAR-T cells for B-cell malignancies. Proc Natl Acad Sci USA 2016;113:E459-68. [Crossref] [PubMed]
- Ryan CE, Logan AC, Rezvani A, et al. Ibrutinib Treatment of Relapsed CLL Following Allogeneic Transplantation: Sustained Disease Response and Promising Donor Immune Modulation. Blood 2014;124:1186-6.
- Fraietta JA, Beckwith KA, Patel PR, et al. Ibrutinib enhances chimeric antigen receptor T-cell engraftment and efficacy in leukemia. Blood 2016;127:1117-27. [Crossref] [PubMed]
- Alapat D, Coviello-Malle J, Owens R, et al. Diagnostic usefulness and prognostic impact of CD200 expression in lymphoid malignancies and plasma cell myeloma. Am J Clin Pathol 2012;137:93-100. [Crossref] [PubMed]