A core of macrophages facilitates ovarian cancer metastases
Ovarian cancer (OC) spheroids require tumor-associated macrophages (TAMs) to survive anoikis
Metastases is nowadays the leading cause of death of cancer patients and of relevance, this study of Yin et al. focuses in metastases establishment during the metastatic process (1). In OC, one of the most common observed metastases is through the transcoelomic route, leading to peritoneal metastases and ascites production (2). In contrast to other epithelial tumor metastasis, cells that break away from the primary tumor can be directly transported via the peritoneal fluid. This avoids the need to cross the vascular endothelium, to survive within the circulation and to leave the blood vasculature at the final destination. However, the first challenge these tumor cells face is to survive anoikis [cell death induced by lack of extracellular matrix (ECM) support]. Mechanisms such as upregulation of RAB25 (3), overexpression of B7-H4 (4) and activation of the Src/Akt/Erk signaling pathway (5) by tumor cells have been reported to prevent anoikis and support immune surveillance escape.
Free-floating aggregates of tumor cells, known as spheroids, are detected in the ascites of OC patients. These structures were proposed to be a survival mechanism by maintaining cell-cell contact and co-stimulation under anchorage-independent growth conditions. In a later stage, these spheroids are then able to attach firmly at different sites of the peritoneum as distant metastases. Not surprisingly, the structure of the spheroids itself offers a shield that also protects them from apoptosis. In these spheroids TAMs seem to form the soil that enables tumor cell survival. However, as macrophages can also act against tumor cells, they might also themselves be shielded inside these spheroid structures from signals turning them against the tumor.
Protective properties of OC spheroids
Related to this shield, the spheroid three-dimensional structure also provides tumor cells with increased drug resistance. As the spheroids are avascular, and thus not perfused, the penetrance of drugs into the core of the spheroid is limited. Since OC cells have to survive independent of anchorage in the peritoneal cavity, the main function of these spheroids is to generate a microenvironment that can support tumor growth and cell survival. Hence, it is not surprising that cell-cell adhesion molecules play an important role on this process. Yet it is still not clear which main players are involved. One potential player might be E-cadherin, which is expressed within the tumor cells surrounding TAMs. E-cadherin upregulation seems to be specific for the malignant transformations and its presence has been shown to be important for maintaining spheroid integrity (6). However, in the context of epithelial-mesenchymal transition (EMT), others have shown that E-cadherin expression in ascitic spheroids and at metastatic sites is lower than in the primary tumor, and that low expression or absence of E-cadherin predicts poor patient survival (7). Cadherin expression switches during tumor progression being differentially expressed across the primary tumor and the metastatic site, while sharing similar functions. It is known that loss of E-cadherin is partially compensated by upregulation of N-cadherin and that both might act synergistically. While E-cadherin mediated cell-cell adhesion promotes cell survival in the absence of ECM, N-cadherin can also promote cell growth and survival in this context (8). Thus, even though E-cadherin is expressed, the previous research suggests that there might be additional mechanisms involved, independent of cadherin mediated cell-cell adhesion, in OC spheroid formation.
In this line, the study reveals that macrophages provide anchorage for tumor cells via a specific integrin interaction. Here they find high ICAM-1 expression in cancer cells, which binds Cd11b-β2integrin of TAMs (Figure 1). Previously, several integrins that mediate spheroid formation by binding to different components of the ECM were identified (9). Laminin and collagen IV-binding integrins were shown to be present in ascites and the mesothelium, were the spheroids attach before EMT and invasion. Nonetheless, a specific TAM integrin that binds tumor cells in OC is for the first time described in this study.
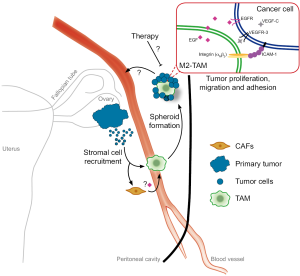
TAMs as ECM for tumor cells
As mentioned before, a so far not characterized role of TAMs in OC is shown in this study. The authors show that F4/80+ CD206+ TAMs offer structural help to tumor cells to survive and proliferate. Indeed, within the core of OC ascitic spheroids, both in human and mouse, macrophages are present. Moreover, by depleting TAMs with clodronate liposomes in tumor-bearing mice, the tumor burden was lowered and they survived longer. Vice versa, injection of “educated” TAMs together with OC cells in recipient mice supported tumor growth and let to a shorter survival. Thereby the authors prove that TAMs are essential for peritoneal spheroid formation and growth. Of clinical relevance, the presence of CD68+ cells (human macrophages) in OC spheroids negatively correlated to overall survival (OS) of patients.
In solid tumors, the function of TAMs has been extensively studied (10). Briefly, they support invasion and angiogenesis through protease or growth factor secretion and by secreting a plethora of cytokines they induce immunosuppression and metastasis. From studies in breast cancer, it is known that tumor cells enter into blood vessels often at clusters of macrophages attached to the abluminal side. Interestingly, a subpopulation of TAM that secrets EGF has been shown to guide tumor cells in the stroma towards a blood vessel, where they can then escape into the circulation. This process requires CSF-1 and EGF signaling in TAMs and tumor cells, respectively (11,12). As blocking EGF dramatically reduced the number of tumor cells entering the bloodstream, it’s natural to think that EGF (from TAMs)—EGFR (in tumor cells) signaling supports tumor cell survival in the circulation. This observation makes us question whether this can be a general mechanism of TAM-cancer cell communication. Cancer cells not only depend on macrophages as a source of different cytokines, but also attract and polarize specific macrophage populations that offer an ECM to survive in the bloodstream or in the peritoneal fluid, as shown here (Figure 1).
M2 macrophages in OC spheroids
In this study the authors describe that spheroid macrophages are polarized towards an M2-like phenotype, characterized by an upregulation of Mr (mannose receptor), Cx3cr1, Arg1 (arginase 1) and Cd163. In general the M1/M2 macrophage classification is useful but very simple. Macrophages are very plastic and can switch between continuums of different phenotypes. This M1/M2 classification shows two extreme polarizations of macrophages and reflects the “fight” and “fix” role of the respective phenotypes. In healthy conditions, M1 macrophages can inhibit cell proliferation, act phagocytically and cause tissue damage upon a stress situation such as a bacterial infection. After danger elimination, M2 macrophages arrive to promote proliferation and tissue repair. However, in a disease like cancer, tumor cells take advantage of the high plasticity of macrophages and by secreting different cytokines force them to support tumor growth (13). Both M1-like and M2-like macrophages are known to be present during cancer progression. As expected, the presence of M1-like TAMs in human tumors is associated with improvement in OS, as in lung cancer (14) or colon cancer (15), while an M2 phenotype is often associated with a poor patient survival (16). Supporting the findings of this study, in gastric cancer more M2 peritoneal macrophages were present in patients with peritoneal dissemination than in those without dissemination (17).
The M2-like phenotype of macrophages can also be enhanced by the ECM. It serves as a structural scaffold for the innate immune cell infiltration, where TAMs have been shown to polarize towards an M2 phenotype (18). Intriguingly, in this study the deposition of ECM and the role of other stromal cells, such as fibroblasts (the major “resident” cell population in the peritoneal cavity), have not been investigated and we therefore do not know if they form part of the spheroid structure (Figure 1). Cancer-associated fibroblasts (CAFs) are known to be able to secrete cytokines to recruit immune cells and to support tumor growth and metastasis (19). Interestingly, in colon cancer, CAFs are known to secrete EGF and to mediate tumor cell proliferation and survival (20). We hypothesize that CAFs might be both a source and a form of regulation of EGF in the peritoneal cavity microenvironment. Future research should also address this question.
Tumor driven macrophage recruitment via VEGF?
In line, another question not addressed by this study is the origin of these macrophages. Are they tissue-resident peritoneal macrophages or are they recruited from the bone marrow via the circulation? Monocytes and macrophages migrate towards damaged tissues under the influence of cytokines, chemokines and growth factors. Interestingly, analysis of the growth factors that TAMs provide to tumor cells revealed that EGF was exclusively produced by TAMs but also that VEGF-A was highly expressed exclusively by tumor cells. Previously, an autocrine role for VEGF/VEGFR-2 was already implicated in protecting tumor cells from anoikis (21) but we propose that in this context, the tumor-derived VEGF might be driving macrophage recruitment.
Tumor lesions are usually hypoxic leading to a strong upregulation of VEGF-A. In solid tumors, VEGF-A and the VEGF family member PlGF have been implicated in the hypoxia-induced recruitment of macrophages (22,23). Moreover, macrophage upregulate VEGF-A during tumor progression (13). Thus, it is surprising that in this model macrophages do not behave similarly. Perhaps, since the spheroids are avascular structures, they don’t require high VEGF-A secretion from macrophages to support tumor angiogenesis (24).
Additionally, a specific Tie2+, CD206+, and VEGF-A secreting population of macrophages has been described to support tumor cell intravasation in direct contact with tumor cells, acting as a bridge through the endothelium (25). Structurally, macrophages have also a “chaperone” function in angiogenesis. During embryonic development they facilitate vascular anastomosis in response to VEGF (26). Thus, even in this different setting of free-floating spheroids, one could speculate that OC cells up-regulate VEGF-A in order to recruit macrophages and later enable attachment and vascularization. Further insight on this might raise possibilities for new-targeted therapies.
VEGF-C and VEGFR3, not only players in lymphangiogenesis
VEGF-C is another member of the VEGF family expressed by cancer cells within OC spheroids. The main known function of VEGF-C is in lymphangiogenesis, where it acts via the tyrosine kinase receptor VEGFR-3 to promote survival, growth and migration of lymphatic endothelial cells (LECs). Interestingly, in the neural system VEGF-C plays a trophic role for oligodendrocyte precursor cells (27). During development Tie2+ macrophages release VEGF-C to regulate angiogenic vessel branching by regulation of EC migration (28). Here Yin and colleges demonstrate that TAM-EGF induces tumor-VEGF-C secretion that in turn activates tumor-VEGFR-3 to induce tumor cell migration (Figure 1). In line with this finding, in lung adenocarcinoma, VEGF-C and VEGFR-3 signaling enhances tumor cell mobility in vitro and metastasis in vivo. Additionally, their expression negatively correlates with patient survival and positively with clinical metastasis (29).
Integrins, mediators of cell-cell interactions in OC spheroids
Moreover, in OC spheroids VEGFR-3 activation induces tumor-ICAM-1 expression, necessary to bind via a CD11b/c αMβ2-integrin complex to TAMs (Figure 1). This supports tumor spheroid formation and might be a mechanism where tumor cells co-opt physiological functions. Under physiological conditions, ICAM-1 takes part in the local immune-surveillance response. ICAM-1 upregulation induces granulocyte infiltration and following destruction by natural killer (NK) or cytotoxic T lymphocytes (CTLs) cells. Thus, ICAM-1 is a cell adhesion molecule that takes part in the cancer cell elimination by immune cells. Yet, its expression has been positively correlated with advanced stages of human gastric cancer (30). In contrast, expression of ICAM-1 on renal and esophageal cancer associates with good prognosis most probably by boosting the host immune response (31,32).
Of clinical relevance, here they demonstrate that both inhibiting EGFR (with erlotinib) and blocking ICAM-1 (with an anti-ICAM-1 antibody) reduced mouse total body weight, ascitic volume and tumor weight and led to smaller and less spheroids.
Therapeutic relevance in the clinics: focus on the EGF-EGFR axis
The findings of this study might open a window to new therapeutic approaches in OC. Most patients with this cancer are diagnosed at advanced stages with more than 75% having peritoneal involvement, often leading to ascites and bowl obstruction (33,34). As OC is often confined in the peritoneal cavity, aggressive therapies are applied with cytoreductive surgery (peritonectomy and potentially organ resections) combined with hyperthermic intraperitoneal chemotherapy (HIPEC) (35,36). Currently, it is unclear if the addition of HIPEC really prolongs the survival of the patients. This year, the Society of Gynecologic Oncology and American Society of Clinical Oncology Clinical Practice Guideline recommends that women at low likelihood of achieving a cytoreduction of <1 cm should receive neoadjuvant chemotherapy (37). For neoadjuvant chemotherapy a platinum/taxane doublet regimen is recommended. While in the past 10 years many studies for OC treatment were focused on anti-angiogenic agents (38), only limited data exists on the targets suggested in this study. The main focus of these previous studies was on targeting the EGF-EGFR pathway and several clinical trials are currently ongoing. Different strategies have been developed to block this pathway such as EGFR inhibitors or antibodies like erlotinib, lapatinib, cetuximab and panitumumab (39-42).
Initial phase II trials were performed with the addition of cetuximab in relapsed platinum-sensitive OC patients. Here 9 out of 26 patients showed an objective response to the combined therapy of cetuximab with carboplatin, but this response rate did not meet criteria to open a further stage of the trial (40). Similarly initial treatment with the addition of cetuximab did not demonstrate prolongation of progression free survival (PFS) (43).
Analogous maintenance therapy with erlotinib after first-line chemotherapy did not prolong OC patient PFS or OS in a phase III trial (41). Also, lapatinib failed to show promising clinical activity (39,44) and panitumumab showed a moderate response rate of 18.6% in a phase II trial (42).
The discouraging results from the previous clinical studies targeting the EGF-EGFR pathway make us ponder whether the observed results by the authors are of any clinical relevance or not, even toughly high EGF expression is associated with a poor survival of OC patients. However, it needs to be taken into account that all clinical studies were performed in advance stages of the disease and often after tumor recurrence. Could there be a therapeutic window of time? It will be impossible to treat patients at the beginning of the metastatic process, but it could be imaginable to treat them early after or perioperatively when performing CRS with or without HIPEC. Similar perioperative trials are currently performed in patients with peritoneal carcinomatosis of colorectal cancer (45). Maybe by blocking the EGF/EGFR signaling after complete CRS clinicians could block initial steps in the relapse of peritoneal metastases, even though in colon cancer such an approach did not improve OS (46). HIPECs with the local intraperitoneal application of targeted therapy are not in clinical use. However, it is unclear how a therapeutic agent should penetrate into an avascular spheroid structure. Alternatively, adjuvant or perioperative EGF/EGFR targeted treatment additional to curative surgery of non-metastatic OC could help to prevent subsequent peritoneal metastases by inhibition of OC spheroids. Especially, microscopic intraoperative tumor cell shedding by the surgical trauma could be targeted.
Future therapeutic targets: still a long way to go
Therapies against the further potential targets of this study like VEGF-C, VEGFR-3 and ICAM-1 are only in very early experimental clinical trials (47,48). However, in murine tumor models it has been well reported that VEGF-C and VEGFR-3 promote metastasis to the lymphatic system and induce tumor cell motility (49). In a melanoma tumor model, which preferentially metastasizes to the lymph nodes, inhibition of VEGF-C before tumor implantation blocked tumor-associated lymphangiogenesis and metastasis (50). Moreover, blocking VEGFR-3 in a breast cancer model reduced tumor growth and metastasis (51). Also, blocking VEGFR-3 with monoclonal antibodies showed effective results as anti-angiogenic therapy (52). Thus, targeting this signaling pathway might be therapeutically relevant for certain types of tumor by mechanisms other than blocking lymphangiogenesis.
Opposite to what is proposed in this study, several efforts have been done to generate therapeutic expression vectors encoding xenogeneic ICAM-1 (53). The idea behind this treatment is to induce complete tumor rejection by CTL-mediated antitumor immunity. Supporting this, in a colon cancer liver metastasis model, a role for ICAM-1 in suppressing M2 macrophage polarization was proposed. Decreased ICAM-1 expression in colon cancer cells was related to aggressive tumors and to poor patient prognosis. Furthermore, they speculate that endogenous ICAM-1 might be a metastatic suppressor (54). Actually, a vaccine-based therapy of established murine tumors called CEA/TRICOM induced a therapeutic antitumor response without toxicity (55). Nevertheless, in other tumor models like breast cancer, ICAM-1 expression has been correlated to poor patient prognosis and proposed as a potential target (56). Altogether reflects a very puzzling situation, which requires further investigation and clearly shows the need of tumor-specific treatments.
Conclusions
In their study Yin et al. discover a novel mechanism for the formation of transcoelomic metastasis of OC. They observed that tumor cells are in close interaction with macrophages within the core of tumor spheroids. Also, they have discovered the EGF/EGFR, VEGF-C/VEGFR3 and ICAM-1 as key signaling pathways in this cancer cell-macrophage interaction. However, current clinical data from drugs targeting these pathways does not show promising results. Thus, more work will be needed to define an optimal treatment regimen.
Acknowledgments
Funding: None.
Footnote
Provenance and Peer Review: This article was commissioned and reviewed by the Section Editor Hao Feng (Experimental Surgical Research, Department of General, Visceral, Transplant, Vascular and Thoracic Surgery, Hospital of the LMU Munich, Munich, Germany).
Conflicts of Interest: All authors have completed the ICMJE uniform disclosure form (available at http://dx.doi.org/10.21037/tcr.2017.01.27). The authors have no conflicts of interest to declare.
Ethical Statement: The authors are accountable for all aspects of the work in ensuring that questions related to the accuracy or integrity of any part of the work are appropriately investigated and resolved.
Open Access Statement: This is an Open Access article distributed in accordance with the Creative Commons Attribution-NonCommercial-NoDerivs 4.0 International License (CC BY-NC-ND 4.0), which permits the non-commercial replication and distribution of the article with the strict proviso that no changes or edits are made and the original work is properly cited (including links to both the formal publication through the relevant DOI and the license). See: https://creativecommons.org/licenses/by-nc-nd/4.0/.
References
- Yin M, Li X, Tan S, et al. Tumor-associated macrophages drive spheroid formation during early transcoelomic metastasis of ovarian cancer. J Clin Invest 2016;126:4157-73. [Crossref] [PubMed]
- Tan DS, Agarwal R, Kaye SB. Mechanisms of transcoelomic metastasis in ovarian cancer. Lancet Oncol 2006;7:925-34. [Crossref] [PubMed]
- Cheng KW, Lahad JP, Kuo WL, et al. The RAB25 small GTPase determines aggressiveness of ovarian and breast cancers. Nat Med 2004;10:1251-6. [Crossref] [PubMed]
- Salceda S, Tang T, Kmet M, et al. The immunomodulatory protein B7-H4 is overexpressed in breast and ovarian cancers and promotes epithelial cell transformation. Exp Cell Res 2005;306:128-41. [Crossref] [PubMed]
- Cai Q, Yan L, Xu Y. Anoikis resistance is a critical feature of highly aggressive ovarian cancer cells. Oncogene 2015;34:3315-24. [Crossref] [PubMed]
- Choi PW, Yang J, Ng SK, et al. Loss of E-cadherin disrupts ovarian epithelial inclusion cyst formation and collective cell movement in ovarian cancer cells. Oncotarget 2016;7:4110-21. [PubMed]
- Daraï E, Scoazec JY, Walker-Combrouze F, et al. Expression of cadherins in benign, borderline, and malignant ovarian epithelial tumors: a clinicopathologic study of 60 cases. Hum Pathol 1997;28:922-8. [Crossref] [PubMed]
- Wheelock MJ, Shintani Y, Maeda M, et al. Cadherin switching. J Cell Sci 2008;121:727-35. [Crossref] [PubMed]
- Casey RC, Burleson KM, Skubitz KM, et al. Beta 1-integrins regulate the formation and adhesion of ovarian carcinoma multicellular spheroids. Am J Pathol 2001;159:2071-80. [Crossref] [PubMed]
- Qian BZ, Pollard JW. Macrophage diversity enhances tumor progression and metastasis. Cell 2010;141:39-51. [Crossref] [PubMed]
- Wyckoff J, Wang W, Lin EY, et al. A paracrine loop between tumor cells and macrophages is required for tumor cell migration in mammary tumors. Cancer Res 2004;64:7022-9. [Crossref] [PubMed]
- Goswami S, Sahai E, Wyckoff JB, et al. Macrophages promote the invasion of breast carcinoma cells via a colony-stimulating factor-1/epidermal growth factor paracrine loop. Cancer Res 2005;65:5278-83. [Crossref] [PubMed]
- Mantovani A, Sozzani S, Locati M, et al. Macrophage polarization: tumor-associated macrophages as a paradigm for polarized M2 mononuclear phagocytes. Trends Immunol 2002;23:549-55. [Crossref] [PubMed]
- Ma J, Liu L, Che G, et al. The M1 form of tumor-associated macrophages in non-small cell lung cancer is positively associated with survival time. BMC Cancer 2010;10:112. [Crossref] [PubMed]
- Edin S, Wikberg ML, Dahlin AM, et al. The distribution of macrophages with a M1 or M2 phenotype in relation to prognosis and the molecular characteristics of colorectal cancer. PLoS One 2012;7:e47045 [Crossref] [PubMed]
- Schmidt T, Ben-Batalla I, Schultze A, et al. Macrophage-tumor crosstalk: role of TAMR tyrosine kinase receptors and of their ligands. Cell Mol Life Sci 2012;69:1391-414. [Crossref] [PubMed]
- Yamaguchi T, Fushida S, Yamamoto Y, et al. Tumor-associated macrophages of the M2 phenotype contribute to progression in gastric cancer with peritoneal dissemination. Gastric Cancer 2016;19:1052-65. [Crossref] [PubMed]
- Kobayashi N, Miyoshi S, Mikami T, et al. Hyaluronan deficiency in tumor stroma impairs macrophage trafficking and tumor neovascularization. Cancer Res 2010;70:7073-83. [Crossref] [PubMed]
- Quail DF, Joyce JA. Microenvironmental regulation of tumor progression and metastasis. Nat Med 2013;19:1423-37. [Crossref] [PubMed]
- Tommelein J, Verset L, Boterberg T, et al. Cancer-associated fibroblasts connect metastasis-promoting communication in colorectal cancer. Front Oncol 2015;5:63. [Crossref] [PubMed]
- Sher I, Adham SA, Petrik J, et al. Autocrine VEGF-A/KDR loop protects epithelial ovarian carcinoma cells from anoikis. Int J Cancer 2009;124:553-61. [Crossref] [PubMed]
- Murdoch C, Giannoudis A, Lewis CE. Mechanisms regulating the recruitment of macrophages into hypoxic areas of tumors and other ischemic tissues. Blood 2004;104:2224-34. [Crossref] [PubMed]
- Chanmee T, Ontong P, Konno K, et al. Tumor-associated macrophages as major players in the tumor microenvironment. Cancers (Basel) 2014;6:1670-90. [Crossref] [PubMed]
- Lewis JS, Landers RJ, Underwood JC, et al. Expression of vascular endothelial growth factor by macrophages is up-regulated in poorly vascularized areas of breast carcinomas. J Pathol 2000;192:150-8. [Crossref] [PubMed]
- Harney AS, Arwert EN, Entenberg D, et al. Real-Time Imaging Reveals Local, Transient Vascular Permeability, and Tumor Cell Intravasation Stimulated by TIE2hi Macrophage-Derived VEGFA. Cancer Discov 2015;5:932-43. [Crossref] [PubMed]
- Schmidt T, Carmeliet P. Blood-vessel formation: Bridges that guide and unite. Nature 2010;465:697-9. [Crossref] [PubMed]
- Ruiz de Almodovar C, Lambrechts D, Mazzone M, et al. Role and therapeutic potential of VEGF in the nervous system. Physiol Rev 2009;89:607-48. [Crossref] [PubMed]
- Tammela T, Zarkada G, Nurmi H, et al. VEGFR-3 controls tip to stalk conversion at vessel fusion sites by reinforcing Notch signalling. Nat Cell Biol 2011;13:1202-13. [Crossref] [PubMed]
- Su JL, Yang PC, Shih JY, et al. The VEGF-C/Flt-4 axis promotes invasion and metastasis of cancer cells. Cancer Cell 2006;9:209-23. [Crossref] [PubMed]
- Maruo Y, Gochi A, Kaihara A, et al. ICAM-1 expression and the soluble ICAM-1 level for evaluating the metastatic potential of gastric cancer. Int J Cancer 2002;100:486-90. [Crossref] [PubMed]
- Tomita Y, Nishiyama T, Watanabe H, et al. Expression of intercellular adhesion molecule-1 (ICAM-1) on renal-cell cancer: possible significance in host immune responses. Int J Cancer 1990;46:1001-6. [Crossref] [PubMed]
- Hosch SB, Izbicki JR, Pichlmeier U, et al. Expression and prognostic significance of immunoregulatory molecules in esophageal cancer. Int J Cancer 1997;74:582-7. [Crossref] [PubMed]
- Sehouli J, Senyuva F, Fotopoulou C, et al. Intra-abdominal tumor dissemination pattern and surgical outcome in 214 patients with primary ovarian cancer. J Surg Oncol 2009;99:424-7. [Crossref] [PubMed]
- Lowe KA, Chia VM, Taylor A, et al. An international assessment of ovarian cancer incidence and mortality. Gynecol Oncol 2013;130:107-14. [Crossref] [PubMed]
- Di Giorgio A, De Iaco P, De Simone M, et al. Cytoreduction (Peritonectomy Procedures) Combined with Hyperthermic Intraperitoneal Chemotherapy (HIPEC) in Advanced Ovarian Cancer: Retrospective Italian Multicenter Observational Study of 511 Cases. Ann Surg Oncol 2016; [Epub ahead of print]. [Crossref] [PubMed]
- Sleightholm R, Foster JM, Smith L, et al. The American Society of Peritoneal Surface Malignancies Multi-Institution evaluation of 1,051 advanced ovarian cancer patients undergoing cytoreductive surgery and HIPEC: An introduction of the peritoneal surface disease severity score. J Surg Oncol 2016;114:779-84. [Crossref] [PubMed]
- Wright AA, Bohlke K, Armstrong DK, et al. Neoadjuvant Chemotherapy for Newly Diagnosed, Advanced Ovarian Cancer: Society of Gynecologic Oncology and American Society of Clinical Oncology Clinical Practice Guideline. J Clin Oncol 2016;34:3460-73. [Crossref] [PubMed]
- Ledermann JA, Raja FA. Targeted trials in ovarian cancer. Gynecol Oncol 2010;119:151-6. [Crossref] [PubMed]
- Garcia AA, Sill MW, Lankes HA, et al. A phase II evaluation of lapatinib in the treatment of persistent or recurrent epithelial ovarian or primary peritoneal carcinoma: A gynecologic oncology group study. Gynecol Oncol 2012;124:569-74. [Crossref] [PubMed]
- Secord AA, Blessing JA, Armstrong DK, et al. Phase II trial of cetuximab and carboplatin in relapsed platinum-sensitive ovarian cancer and evaluation of epidermal growth factor receptor expression: a Gynecologic Oncology Group study. Gynecol Oncol 2008;108:493-9. [Crossref] [PubMed]
- Vergote IB, Jimeno A, Joly F, et al. Randomized phase III study of erlotinib versus observation in patients with no evidence of disease progression after first-line platin-based chemotherapy for ovarian carcinoma: a European Organisation for Research and Treatment of Cancer-Gynaecological Cancer Group, and Gynecologic Cancer Intergroup study. J Clin Oncol 2014;32:320-6. [Crossref] [PubMed]
- Steffensen KD, Waldstrøm M, Pallisgård N, et al. Panitumumab and pegylated liposomal doxorubicin in platinum-resistant epithelial ovarian cancer with KRAS wild-type: the PaLiDo study, a phase II nonrandomized multicenter study. Int J Gynecol Cancer 2013;23:73-80. [Crossref] [PubMed]
- Konner J, Schilder RJ, DeRosa FA, et al. A phase II study of cetuximab/paclitaxel/carboplatin for the initial treatment of advanced-stage ovarian, primary peritoneal, or fallopian tube cancer. Gynecol Oncol 2008;110:140-5. [Crossref] [PubMed]
- Weroha SJ, Oberg AL, Ziegler KL, et al. Phase II trial of lapatinib and topotecan (LapTop) in patients with platinum-refractory/resistant ovarian and primary peritoneal carcinoma. Gynecol Oncol 2011;122:116-20. [Crossref] [PubMed]
- Glockzin G, Rochon J, Arnold D, et al. A prospective multicenter phase II study evaluating multimodality treatment of patients with peritoneal carcinomatosis arising from appendiceal and colorectal cancer: the COMBATAC trial. BMC Cancer 2013;13:67. [Crossref] [PubMed]
- Maillet M, Glehen O, Lambert J, et al. Early Postoperative Chemotherapy After Complete Cytoreduction and Hyperthermic Intraperitoneal Chemotherapy for Isolated Peritoneal Carcinomatosis of Colon Cancer: A Multicenter Study. Ann Surg Oncol 2016;23:863-9. [Crossref] [PubMed]
- Hansson M, Gimsing P, Badros A, et al. A Phase I Dose-Escalation Study of Antibody BI-505 in Relapsed/Refractory Multiple Myeloma. Clin Cancer Res 2015;21:2730-6. [Crossref] [PubMed]
- Saif MW, Knost JA, Chiorean EG, et al. Phase 1 study of the anti-vascular endothelial growth factor receptor 3 monoclonal antibody LY3022856/IMC-3C5 in patients with advanced and refractory solid tumors and advanced colorectal cancer. Cancer Chemother Pharmacol 2016;78:815-24. [Crossref] [PubMed]
- Su JL, Yen CJ, Chen PS, et al. The role of the VEGF-C/VEGFR-3 axis in cancer progression. Br J Cancer 2007;96:541-5. [Crossref] [PubMed]
- Lin J, Lalani AS, Harding TC, et al. Inhibition of lymphogenous metastasis using adeno-associated virus-mediated gene transfer of a soluble VEGFR-3 decoy receptor. Cancer Res 2005;65:6901-9. [Crossref] [PubMed]
- Varney ML, Singh RK. VEGF-C-VEGFR3/Flt4 axis regulates mammary tumor growth and metastasis in an autocrine manner. Am J Cancer Res 2015;5:616-28. [PubMed]
- Tammela T, Zarkada G, Wallgard E, et al. Blocking VEGFR-3 suppresses angiogenic sprouting and vascular network formation. Nature 2008;454:656-60. [Crossref] [PubMed]
- Kanwar JR, Berg RW, Yang Y, et al. Requirements for ICAM-1 immunogene therapy of lymphoma. Cancer Gene Ther 2003;10:468-76. [Crossref] [PubMed]
- Yang M, Liu J, Piao C, et al. ICAM-1 suppresses tumor metastasis by inhibiting macrophage M2 polarization through blockade of efferocytosis. Cell Death Dis 2015;6:e1780 [Crossref] [PubMed]
- Hodge JW, Grosenbach DW, Aarts WM, et al. Vaccine therapy of established tumors in the absence of autoimmunity. Clin Cancer Res 2003;9:1837-49. [PubMed]
- Guo P, Huang J, Wang L, et al. ICAM-1 as a molecular target for triple negative breast cancer. Proc Natl Acad Sci U S A 2014;111:14710-5. [Crossref] [PubMed]