Rogue one: another faction of the Wnt empire implicated in assisting GBM progression
A diagnosis of glioblastoma multiforme (GBM), currently entails a dire prognosis: notorious for its potent propensity to spread across the encompassing brain parenchyma and the near omnipresence of recurrence, GBM has held on to its throne as one of the most lethal malignancies with a relentless tenacity over the decades, despite advances in diagnostic techniques and the advent of aggressive multimodality treatment regimens (1,2). The median survival of patients remains dismal, less than two years, defying even three-pronged therapies with surgical resection, radiotherapy, and chemotherapy (3). A heterogeneous tapestry of different cell types comprises this primary brain tumor, giving it the latter part of its name, multiforme (4). In addition to the transformed astrocytes from which GBM is derived, and the endothelial cells associated with its characteristically rich vascularization, there exists a distinct cohort of undifferentiated multipotent cells ensconced within the tumor (5). It is theorized that these quasi-stem cells drive the distinctive features of GBM, such as multimodality therapeutic resistance, invasiveness, neoangiogenesis, vasculogenic mimicry and recurrence, which render attempts to treat it a veritable quagmire (6,7). According to the Cancer Stem Cell (CSC) hypothesis, these Glioma Stem Cells (GSCs) are maintained as a niche within the tumor, similar to tissue-specific somatic stem cells (8).
In the November 2016 issue of Cell, Hu et al. illuminate some of the aberrations in GSCs compared to neural stem/progenitor cells (NSCs); the latter play a pivotal role in the normal embryonic development of the nervous system, differentiating into neurons and supporting glial, and some persisting to become adult stem cells (9,10). GSCs show marked similarities to NSCs: both express stem cell markers, the capacity to self-renew, that is to maintain the undifferentiated state through numerous rounds of cell division, and the potency to differentiate into multiple lineages (10). Indeed, studies have implicated transformed NSCs as an origin of malignant astrocytomas (11). In addition to the characteristic features mentioned earlier, these stem cell-like properties bestow upon GSCs a robust potential for tumor initiation. In contrast to NSCs however, GSCs have been documented to undergo trans-differentiation, suggesting an inability to commit to a final differentiated state, which could confer the volatility required to withstand multiple therapeutic stresses and its concomitant selection pressures (12,13). It seems increasingly likely that these GSCs, whose markedly enhanced plasticity enables them to evade and adapt to the initial round of treatment that may succeed in shrinking the primary tumor, drive GBM’s recurrence with radio- and chemoresistance (14,15). Hence, the elucidation of what sets these GSCs apart is of paramount importance and a worthwhile avenue for research. To that end, the authors established a de novo GBM model derived from human NSCs (hNSCs) that would allow for the detection of genes possibly contributing to the anomalous developmental plasticity of GSCs (Figure 1). These hNSCs were transduced to overexpress the proto-oncogene c-Myc, in order to immortalize them for ease of culture in vitro (16). c-Myc is robustly overexpressed in many cancers, including GBM (2), and the hNSCs employed demonstrated many of the properties shared between NSCs and GSCs. The hNSCs were oncogenically transformed by lentiviral co-transduction of a mutant form of p53 (p53DN), which exerts a dominant-negative effect on the tumor suppressor function of wild-type p53, and a lipidated, constitutively active variant of the serine/threonine kinase, AKT. This approach yielded an ideal model to investigate GSCs, due to the parallels between GSCs and NSCs. Furthermore, the transductions with p53DN and N-myristolated AKT (myr-Akt) served to emulate the deregulation of TP53-ARF-MDM2 and PTEN-PI3K-AKT pathways respectively, as seen in the majority of GBM cases (17,18). The loss of p53 function, which compromises the G1 to S phase checkpoint and negates cell cycle arrest in response to DNA damage, combine with the enhanced activity of AKT and c-Myc overexpression to drive gliomagenesis, by enabling cells to evade apoptosis, accumulate mutations, proliferate and indefinitely postpone terminal differentiation. Only doubly infected hNSCs (p53DN-AKT-hNSCs), expressing both p53DN and myr-AKT, exhibited anchorage-independent growth, a hallmark of carcinogenesis, as evidenced by the formation of colonies in soft agar. These p53DN-AKT-hNSCs readily formed tumors with classical GBM features following intracranial injection in immunocompromised mice, unlike both of the singly transduced hNSCs expressing either p53DN or myr-AKT. In order to control for the innate sequence non-specificity of lentiviral integrase (19) and any other biases introduced by the line of hNSCs from which their model was derived, the authors transduced c-Myc, p53DN and myr-AKT simultaneously in a different primary human NSC line, which demonstrated similar tumorigenic potential following intracranial injection in mice, hence confirming the reproducibility of their de novo model for GBM.
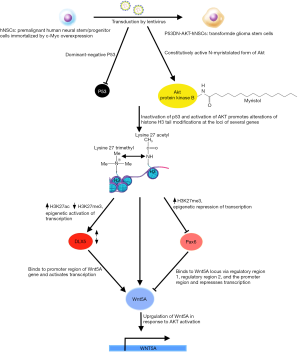
The tumors derived from p53DN-AKT-hNSCs evidently induced the formation of glioma stem cells (iGSCs), evidenced by their abilities to trigger tumor reformation and differentiate into glial and neuronal linages, albeit likely retaining the capacity for opportunistic self-renewal and transdifferentiation. Of more intrigue, however, is what differentiates GSCs from their non-malignant counterparts, that is the ability of the former to give rise to endothelial cells (ECs), a testament to the aberrantly heightened plasticity of GSCs compared to NSCs. This is of particular clinical relevance as evidence suggests that when patients are treated with therapies targeting angiogenesis, such as VEGF-inhibitors like bevacizumab, GBM tumors compensate by increased differentiation of GSCs to ECs (20,21). The neovascularization of GBM feeds the exponential growth of the tumor and its invasiveness, therefore representing an attractive target for novel therapies. If the mechanism behind the generation of GCS-derived endothelial-like cells (GdECs), which most likely contributes to the pathogenesis of GBM, were to be delineated, novel therapies could be devised, which, in conjunction with existing anti-angiogenic chemotherapies, could impede neovascularization and hence halt tumorigenesis. To that end, the authors compared differences in gene expression between the parental NSCs and the iGSCs. In order to narrow their search for the culprits contributing to the stemness of their oncogene-induced model, the authors used bioinformatics, first identifying signaling pathways involved in stem cells using the Molecular Signatures Database (MSigDB), which they used to guide their transcriptomic gene set enrichment analysis (GSEA) that revealed a statistically significant upregulation of EC signaling following oncogenic induction (22). Genome-wide chromatin immunoprecipitation sequencing (Chip-seq) was utilized to detect epigenetic changes by revealing the post-translational modification states of the N-terminal Lysine of H3 histone proteins at the loci of genes of interest. This showed the epigenetic the activation of EC signaling, as many genes involved in the pathway showed a dynamic switch from repressive H3K27 trimethylation (me3) to activating H3K27 acetylation (ac) (23). These prompted the authors to confirm the expression of EC phenotypes by iGSCs, such as the expression of the EC markers VE-Cadherin (CD-144) and PECAM-1 (CD31), using fluorescence-activated cell sorting (FACs) and fluorescent acetylated-low density lipoprotein (Dil-AcLDL) uptake under NSC and EC culture conditions respectively. 12.8% and 5.8% of these p53DN-AKT-hNSC derived iGSCs expressed CD144 and CD31 respectively, along with other classical EC markers and functional features, like increased uptake of acetylated-LDL. With FACSs analysis revealing the co-expression of CD144 with the stem cell marker CD133 and EC formation in vivo in tumors, these findings together confirm the presence of GdECs derived from this de novo model (24).
In order to home in on exactly what transpired to elicit this differentiation, the authors focused on genes associated with high AKT activity, given the correlation of high AKT activation with poor prognosis, and of course, the central role of constitutive AKT activation in the oncogenic transformation of their model. The intersection of genes associated with high AKT activity and the genes that were epigenetically activated upon transformation of their model narrowed the list of culprits down to only eight genes. Individual knockdowns of each of these eight genes by RNA interference in CD133+/CD144+ p53DN-AKT-NSCs strongly implicated WNT5A as a driver of the process. This suspicion was bolstered using the WNT5A antagonist, BOX5, which markedly reduced the proportion of CD133+/CD144+ p53DN-AKT-NSCs and their EC-like property of tubular network formation. Furthermore, complimentary to the activating alterations in the gene locus of WNT5A were similar changes at the locus of its transcriptional activator, DLX5, which was shown to have a DNA binding motif specific to the promoter region of the WNT5A gene. On the other hand, PAX6, which also possesses a DNA binding site specific for regulatory regions of the WNT5A gene but represses transcription had contrary, repressive histone mark alterations. Doubtlessly, these had the combined effect of activating WNT expression.
WNT5A is a lipid-modified secreted glycoprotein that serves as a ligand of the Wnt signal transduction pathway, which has long been associated with carcinogenesis. The normal functions of the Wnt pathway are critical during embryogenesis and include promoting rapid cell proliferation, with members of the pathway being widely expressed in mouse cleavage stage embryos. It goes without saying that loss of regulation of this pathway has a strong potential to cause cancer. However, there are a variety of Wnt signaling pathways with a plethora of often opposing functions. While the canonical Wnt signaling pathway is dependent on β-catenin and has been and implicated in many cancers (25), including gliomas (26,27), and is even correlated with worse prognoses (28), this is likely not involved in the process of GdEC formation: WNT5A is a typical non-canonical Wnt ligand and, curiously enough, inhibition of canonical Wnt signaling is one of its known properties (17). This highlights a glaring paradox in the role of WNT5A in tumor progression, as previous research has shown the canonical pathway to be intimately involved in stem/progenitor cells and cancer stem cells alike. There is no evidence to suggest that WNT5A hinders GBM’s formation of stem cells, and on the contrary, immunohistochemical and transcriptomic analysis of paired primary/recurrent GBM patient samples reveal WNT5A to be consistently upregulated in recurrent tumors, with a symmetric increase in the incidence of GdECs. As recurrent tumors are well known to be more plastic and comprised of a higher proportion of GSCs (29), this hints that WNT5A is functioning through a different, as yet unidentified pathway, which has no effect on the canonical Wnt signaling. It could also mean that GSCs simply upregulate β-catenin expression to offset the effect of WNT5A. The authors to not delve into which particular Wnt signaling WNT5A invokes in this context, but Cheng et al. demonstrated that WNT5A regulates endothelial cell proliferation and migration during angiogenesis by activating the Ca+/calmodulin-dependent protein kinase II (30), and it is reasonable to assume that something similar is occurring here.
WNT5A is secreted before binding to an extracellular domain of its G-protein coupled receptor, frizzled, which in turn activates the cytoplasmic protein disheveled that relays the signal to downstream effectors of the pathway, which in this context leads to an increase in cytosolic Ca+ concentrations and the activation of the mTOR/S6K pathway (31). Hence, it may mediate its effect in an autocrine or paracrine manner. WNT5A is robustly epigenetically activated in CD133+/CD144+ cells sorted from p53DN-AKT-NSCs, hinting towards an autocrine mode of action. It would be interesting to see which of the iGSCs express the receptor for WNT5A to reveal whether or not paracrine signaling also plays a role. Paracrine signaling certainly plays a role in the recruitment of host ECs by GdECs, something the authors also accuse WNT5A of enabling in this context. This recruitment, they say, forms a vascular-like niche, which is reminiscent of the generation of vascular pericytes by GSCs reported by Lin Cheng and colleagues, making it quite possible that WNT5A plays a role in the latter phenomenon as well (30).
The standard of care for GBM today involves maximal surgical resection followed by radiotherapy and chemotherapy in the form of the DNA alkylating agent temozolomide (32). It remains to be seen how the process of WNT5A mediated GSC differentiation to EC is affected by stress form these therapies. Of particular interest is the extent to which GdECs retain the stemness of their progenitors. Given its known inhibition of the canonical Wnt pathway, which helps in the maintenance of the GSC pool, WNT5A may favor transdifferentiation at the expense of GSC’s multipotency and self-renewal (Figure 2). The transdifferentiation capacity of GSCs, which could help it evade therapies targeting the Wnt pathway, can be understood by lineage tracing experiments: by utilizing the Cre-lox system, for example, a reporter system can be established to reveal the fate of CD133+/CD144+ cells in real time under conditions of different therapeutic stresses. Likewise, similar fate-mapping experiments can give a detailed depiction of GSC evolution in vivo, something that remains to be accomplished for GBM in a clinical setting.
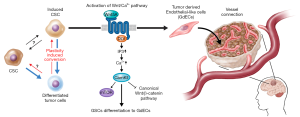
The CRISPR-Cas9 system of introducing indels into the genome in a sequence-specific manner has emerged from its infancy and is currently a powerful tool that can be used to perform a genome-wide screen with commercially available functional genetic single RNA (sgRNA) libraries (33). This can create knockout mutations in almost all human genes and may be used to reveal genes whose loss of function mutations contribute to the transition of GSCs to EC-like cells. This can be done by analyzing the sgRNA populations by reverse-transcriptase quantitate PCR to reveal which sequences from the original library are enriched in the EC-like cells compared to the GSCs from which they originate, following transduction of the latter by the gene encoding the Cas-9 protein and the sgRNA library. Often, this kind of an assay will have hits for many genes besides those that are known oncogenic drivers. This de novo approach of identifying culprit mutations compliment the bioinformatics-guided approach utilized by the authors to arrive at WNT5A.
Baoli Hu and colleagues have presented extensive data that leaves no room for doubt about WNT5A’s aid to tumor neovascularization in GBM patients, including compelling evidence that GdECs are indeed incorporated into host blood vessels. Even though they do not pinpoint the source of host ECs, their proposed model for a vascular-like niche spurring GBM’s breach of the surrounding brain tissue and recurrence, is supported by an increase of WNT5A expression in peritumoral regions compared to their matched intratumoral counterparts from clinical samples. However, the finer mechanistic details of WNT5A induced differentiation of GSCs to GdECs, and the maintenance of peritumoral satellite lesions are yet to be revealed. It is important for the exact pathways by which these processes come about to be elucidated in their entirety, as this will reveal all the essential components that could potentially be targeted by novel drugs, alleviating the challenge of designing drugs that must overcome the blood-brain barrier.
Acknowledgments
Funding: This work was supported by the National Cancer Institute (R00 CA160775) and the American Cancer Society (RSG-16-034-01-DDC) grant.
Footnote
Provenance and Peer Review: This article was commissioned and reviewed by the Section Editor Lichao Sun (State Key Laboratory of Molecular Oncology, National Cancer Center (NCC)/Cancer Hospital, Chinese Academy of Medical Sciences (CAMS), Peking Union Medical College, Beijing, China).
Conflicts of Interest: All authors have completed the ICMJE uniform disclosure form (available at http://dx.doi.org/10.21037/tcr.2017.03.29). The authors have no conflicts of interest to declare.
Ethical Statement: The authors are accountable for all aspects of the work in ensuring that questions related to the accuracy or integrity of any part of the work are appropriately investigated and resolved.
Open Access Statement: This is an Open Access article distributed in accordance with the Creative Commons Attribution-NonCommercial-NoDerivs 4.0 International License (CC BY-NC-ND 4.0), which permits the non-commercial replication and distribution of the article with the strict proviso that no changes or edits are made and the original work is properly cited (including links to both the formal publication through the relevant DOI and the license). See: https://creativecommons.org/licenses/by-nc-nd/4.0/.
References
- Wen PY, Kesari S. Malignant gliomas in adults. N Engl J Med 2008;359:492-507. [Crossref] [PubMed]
- Tran B, Rosenthal MA. Survival comparison between glioblastoma multiforme and other incurable cancers. J Clin Neurosci 2010;17:417-21. [Crossref] [PubMed]
- Lacroix M, Abi-Said D, Fourney DR, et al. A multivariate analysis of 416 patients with glioblastoma multiforme: prognosis, extent of resection, and survival. J Neurosurg 2001;95:190-8. [Crossref] [PubMed]
- Berkman RA, Clark WC, Saxena A, et al. Clonal composition of glioblastoma multiforme. J Neurosurg 1992;77:432-7. [Crossref] [PubMed]
- Yuan X, Curtin J, Xiong Y, et al. Isolation of cancer stem cells from adult glioblastoma multiforme. Oncogene 2004;23:9392-400. [Crossref] [PubMed]
- Bao S, Wu Q, McLendon RE, et al. Glioma stem cells promote radioresistance by preferential activation of the DNA damage response. Nature 2006;444:756-60. [Crossref] [PubMed]
- Eyler CE, Rich JN. Survival of the fittest: cancer stem cells in therapeutic resistance and angiogenesis. J Clin Oncol 2008;26:2839-45. [Crossref] [PubMed]
- Singh SK, Clarke ID, Terasaki M, et al. Identification of a cancer stem cell in human brain tumors. Cancer Res 2003;63:5821-8. [PubMed]
- Gage FH. Mammalian neural stem cells. Science 2000;287:1433-8. [Crossref] [PubMed]
- Cheng L, Huang Z, Zhou W, et al. Glioblastoma stem cells generate vascular pericytes to support vessel function and tumor growth. Cell 2013;153:139-52. [Crossref] [PubMed]
- Llaguno SA, Chen J, Kwon CH, et al. Neural and cancer stem cells in tumor suppressor mouse models of malignant astrocytoma. Cold Spring Harb Symp Quant Biol 2008;73:421-6. [Crossref] [PubMed]
- Soda Y, Marumoto T, Friedmann-Morvinski D, et al. Transdifferentiation of glioblastoma cells into vascular endothelial cells. Proc Natl Acad Sci U S A 2011;108:4274-80. [Crossref] [PubMed]
- Scully S, Francescone R, Faibish M, et al. Transdifferentiation of glioblastoma stem-like cells into mural cells drives vasculogenic mimicry in glioblastomas. J Neurosci 2012;32:12950-60. [Crossref] [PubMed]
- Qiang L, Wu T, Zhang HW, et al. HIF-1alpha is critical for hypoxia-mediated maintenance of glioblastoma stem cells by activating Notch signaling pathway. Cell Death Differ 2012;19:284-94. [Crossref] [PubMed]
- Eramo A, Ricci-Vitiani L, Zeuner A, et al. Chemotherapy resistance of glioblastoma stem cells. Cell Death Differ 2006;13:1238-41. [Crossref] [PubMed]
- Dang CV. c-Myc target genes involved in cell growth, apoptosis, and metabolism. Mol Cell Biol 1999;19:1-11. [Crossref] [PubMed]
- Zhu HH, Zhu XY, Zhou MH, et al. Effect of WNT5A on epithelial-mesenchymal transition and its correlation with tumor invasion and metastasis in nasopharyngeal carcinoma. Asian Pac J Trop Med 2014;7:488-91. [Crossref] [PubMed]
- Holland EC, Celestino J, Dai C, et al. Combined activation of Ras and Akt in neural progenitors induces glioblastoma formation in mice. Nat Genet 2000;25:55-7. [Crossref] [PubMed]
- Marini B, Kertesz-Farkas A, Ali H, et al. Nuclear architecture dictates HIV-1 integration site selection. Nature 2015;521:227-31. [Crossref] [PubMed]
- Bergers G, Hanahan D. Modes of resistance to anti-angiogenic therapy. Nat Rev Cancer 2008;8:592-603. [Crossref] [PubMed]
- Boer JC, Walenkamp AM, den Dunnen WF. Recruitment of bone marrow derived cells during anti-angiogenic therapy in GBM: the potential of combination strategies. Crit Rev Oncol Hematol 2014;92:38-48. [Crossref] [PubMed]
- Subramanian A, Tamayo P, Mootha VK, et al. Gene set enrichment analysis: a knowledge-based approach for interpreting genome-wide expression profiles. Proc Natl Acad Sci U S A 2005;102:15545-50. [Crossref] [PubMed]
- Bernstein BE, Mikkelsen TS, Xie X, et al. A bivalent chromatin structure marks key developmental genes in embryonic stem cells. Cell 2006;125:315-26. [Crossref] [PubMed]
- Wang R, Chadalavada K, Wilshire J, et al. Glioblastoma stem-like cells give rise to tumour endothelium. Nature 2010;468:829-33. [Crossref] [PubMed]
- Fodde R, Brabletz T. Wnt/beta-catenin signaling in cancer stemness and malignant behavior. Curr Opin Cell Biol 2007;19:150-8. [Crossref] [PubMed]
- Rheinbay E, Suva ML, Gillespie SM, et al. An aberrant transcription factor network essential for Wnt signaling and stem cell maintenance in glioblastoma. Cell Rep 2013;3:1567-79. [Crossref] [PubMed]
- Gong A, Huang S. FoxM1 and Wnt/beta-catenin signaling in glioma stem cells. Cancer Res 2012;72:5658-62. [Crossref] [PubMed]
- Rossi M, Magnoni L, Miracco C, et al. beta-catenin and Gli1 are prognostic markers in glioblastoma. Cancer Biol Ther 2011;11:753-61. [Crossref] [PubMed]
- Lee G, Auffinger B, Guo D, et al. Dedifferentiation of Glioma Cells to Glioma Stem-like Cells By Therapeutic Stress-induced HIF Signaling in the Recurrent GBM Model. Mol Cancer Ther 2016;15:3064-76. [Crossref] [PubMed]
- Cheng CW, Yeh JC, Fan TP, et al. Wnt5a-mediated non-canonical Wnt signalling regulates human endothelial cell proliferation and migration. Biochem Biophys Res Commun 2008;365:285-90. [Crossref] [PubMed]
- Semenov MV, Habas R, Macdonald BT, et al. SnapShot: Noncanonical Wnt Signaling Pathways. Cell 2007;131:1378. [Crossref] [PubMed]
- Stupp R, Hegi ME, Gilbert MR, et al. Chemoradiotherapy in malignant glioma: standard of care and future directions. J Clin Oncol 2007;25:4127-36. [Crossref] [PubMed]
- Toledo CM, Ding Y, Hoellerbauer P, et al. Genome-wide CRISPR-Cas9 Screens Reveal Loss of Redundancy between PKMYT1 and WEE1 in Glioblastoma Stem-like Cells. Cell Rep 2015;13:2425-39. [Crossref] [PubMed]