When is KRAS or TP53 mutation predictive of response to immunotherapy for lung cancer?
Programmed Cell Death Protein-1 (PD-1) checkpoint immunotherapy is a relatively new systemic treatment modality for advanced non-small lung cancer (NSCLC) (1,2). To date, pembrolizumab, nivolumab and atezolizumab have been approved by the United States Food and Drug Administration for this indication either in the front-line or relapsed setting. PD-1 checkpoint, an important immunosuppressive mechanism, is exploited by some tumors to evade immune surveillance. Therefore, by blocking of the PD-1 pathway, an effective immune response against the tumors can be mounted. Nevertheless, PD-1 blockade produces tumor responses in only 20–30% of NSCLC patients. The reason for this is not entirely understood. The presence of PD-L1 protein on tumor cells, while predictive of tumor response, is not a necessary factor. To date, several factors, ranging from clinical characteristics such as smoking status to molecular markers such as tumor mutational burden (TMB) or the presence/absence of some mutations, have been linked to tumor response following PD-1 blockade immunotherapy (3).
In a recent publication (4), the presence of KRAS or TP53 mutation has been proposed as another predictive factor. Dong and colleagues from Guangdong Lung Cancer Institute, China, analyzed public datasets and found KRAS and TP53 mutations to be positively associated with PD-L1 expression. The investigators also analyzed clinical data available from 54 patients treated with PD-1 blockade immunotherapy, including 20 patients treated at their center. Of these, there were 11 patients with KRAS mutation (20.4%). It turned out that 10 out of these 11 patients achieved a durable clinical benefit or partial tumor response (herein referred to as clinical benefit). Furthermore, there were 23 patients with TP53 mutation (42.6%); 12 out of 23 patients derived clinical benefit. Among the 6 patients who had both mutated KRAS and TP53 mutations, all experienced clinical benefit. To put this into perspective, KRAS mutation was the strongest predictor of clinical benefit in this study (Table 1), with odds ratio (OR) 33.0, followed by high TMB with OR 14.0. Although the study was somewhat limited by small sample size and missing data, KRAS mutation is clearly predictive of clinical benefit in this study.
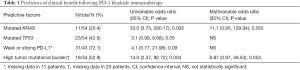
Full table
Nevertheless, an important limitation to this study is that it is not possible to demonstrate the predictive ability of KRAS mutation independently from TMB. This is because almost all patients with available data on TMB and with known KRAS mutation had a high TMB. In fact, there was only 1 patient with KRAS mutation and low TMB in the study, making it difficult to know whether KRAS mutation, by itself, will have any predictive value, when TMB is low. Indeed, this patient happened to derive no clinical benefit from treatment in this study.
The association between KRAS mutation and high TMB has been observed in other recent studies (5,6). For example, in a study of 11,662 lung cancer specimens (6), 1,556 specimens (13.3%) were KRAS-mutated. When all specimens were ranked by TMB, 30% of KRAS-mutated specimens fell into the highest TMB quartile. There is an obvious biological link between KRAS mutation and high TMB. KRAS mutation has long been known to be prevalent among smokers (7) and smoking, which represents a chronic exposure to carcinogen, can lead to an increased mutational load. Rizvi and colleagues have elegantly demonstrated the association between molecular smoking signature, high TMB, and responsiveness to PD-1 blockade immunotherapy (8). They performed a whole exome sequencing experiment in 34 NSCLC specimens and discovered that higher burden of non-synonymous somatic mutations predicted clinical benefit from pembrolizumab. The authors proposed that high TMB represented a genomic landscape which facilitated the occurrence of neoantigen-specific effector T cell responses following PD-1 blockade immunotherapy, thus making the treatment effective.
Additional evidences have lent support to this mutational landscape theory. For instance, among 64 NSCLC patients treated with PD-1 blockade immunotherapy, those with high TMB (defined as ≥15 mutations/megabase) (N=20) had a median on-treatment duration of 64 weeks compared with 17 weeks among others (P=0.01) (6). Interestingly, a number of genetic factors strongly correlated with TMB, either positively or negatively, have been identified. Specifically, high TMB is strongly associated with MSI-High status, PD-L1 amplification, KRAS mutation, non-V600E BRAF mutation, BRCA1 or BRCA2 alteration, DNA repair alteration gene (MLH1, MSH2, POLE) and lack of known drivers (including EGFR, ALK, MET, ROS-1, BRAF V600E) (9). Given the biological mechanism described above, it is likely that the observed association between KRAS or TP53 mutation and response to immunotherapy is mechanistically mediated through high TMB.
There are pros and cons in utilizing mutational status as a surrogate for high TMB to predict response to PD-1 blockade immunotherapy. The key advantage is that currently it is easier to detect specific mutations than to assess TMB. Furthermore, knowing specific mutations is useful for targeted therapy or clinical trial enrollment. However, the disadvantage is that it remains unclear which genes should be tested and how strongly will it predict clinical benefit. Furthermore, KRAS mutation is present almost exclusively in adenocarcinoma, making it less useful for other NSCLC histology. Although KRAS is not the strongest predictor of high TMB (6), its relatively high prevalence compared with other gene candidates makes it potentially useful. Further studies will still be needed to understand how to integrate the available various predictors of tumor response following PD-1 blockade immunotherapy. At this time, PD-L1 protein status which can be obtained quite quickly, easily, and inexpensively through immunohistochemistry remains the key predictor. In short, KRAS or TP53 mutation may be useful to predict response to PD-1 blockade immunotherapy in lung cancer, providing that TMB is high.
Acknowledgments
Funding: None.
Footnote
Provenance and Peer Review: This article was commissioned and reviewed by the Section Editor Runzhe Chen (Department of Thoracic/Head & Neck Medical Oncology, University of Texas MD Anderson Cancer Center, Houston, TX, USA; Department of Hematology and Oncology, Zhongda Hospital of Southeast University, Nanjing, China).
Conflicts of Interest: The author has completed the ICMJE uniform disclosure form (available at http://dx.doi.org/10.21037/tcr.2017.03.65). The author has no conflicts of interest to declare.
Ethical Statement: The author is accountable for all aspects of the work in ensuring that questions related to the accuracy or integrity of any part of the work are appropriately investigated and resolved.
Open Access Statement: This is an Open Access article distributed in accordance with the Creative Commons Attribution-NonCommercial-NoDerivs 4.0 International License (CC BY-NC-ND 4.0), which permits the non-commercial replication and distribution of the article with the strict proviso that no changes or edits are made and the original work is properly cited (including links to both the formal publication through the relevant DOI and the license). See: https://creativecommons.org/licenses/by-nc-nd/4.0/.
References
- Tanvetyanon T, Gray JE, Antonia SJ. PD-1 checkpoint blockade alone or combined PD-1 and CTLA-4 blockade as immunotherapy for lung cancer? Expert Opin Biol Ther 2017;17:305-12. [Crossref] [PubMed]
- Tanvetyanon T, Creelan BC, Antonia SJ. The safety and efficacy of nivolumab in advanced (metastatic) non-small cell lung cancer. Expert Rev Anticancer Ther 2016;16:903-10. [Crossref] [PubMed]
- Khagi Y, Kurzrock R, Patel SP. Next generation predictive biomarkers for immune checkpoint inhibition. Cancer Metastasis Rev 2016; [Epub ahead of print]. [Crossref] [PubMed]
- Dong ZY, Zhong W, Zhang XC, et al. Potential Predictive Value of TP53 and KRAS Mutation Status for Response to PD-1 Blockade Immunotherapy in Lung Adenocarcinoma. Clin Cancer Res 2016; [Epub ahead of print]. [Crossref] [PubMed]
- Davis A, Chae YK, Agte S, et al. Association of tumor mutational burden with smoking and mutation status in non-small cell lung cancer. J Clin Oncol 2017;35:abstr 24.
- Spigel DR, Schrock AB, Fabrizio D, et al. Total mutation burden (TMB) in lung cancer (LC) and relationship with response to PD-1/PD-L1 targeted therapies. J Clin Oncol 2016;34:abstr 9017.
- Ahrendt SA, Decker PA, Alawi EA, et al. Cigarette smoking is strongly associated with mutation of the K-ras gene in patients with primary adenocarcinoma of the lung. Cancer 2001;92:1525-30. [Crossref] [PubMed]
- Rizvi NA, Hellmann MD, Snyder A, et al. Cancer immunology. Mutational landscape determines sensitivity to PD-1 blockade in non-small cell lung cancer. Science 2015;348:124-8. [Crossref] [PubMed]
- Schrock A, Sharma N, Peled N, et al. Updated Dataset Assessing Tumor Mutation Burden (TMB) as a Biomarker for Response to PD-1/PD-L1 Targeted Therapies in Lung Cancer (LC). J Thorac Oncol 2017;12:S422. [Crossref]