Complement and disease: better no factor H than bad factor H
Within just a few minutes the complement system (1) can label a foreign cell that has invaded the bloodstream with millions of copies of C3b. Destruction or clearance of the C3b-coated invader quickly follows. C3b is the activated cleavage product of C3, an abundant serum protein. Numerous diseases have been linked to collateral damage from this aggressive process (2). Recently, Ueda et al. (3) presented a striking example of a mouse model, based on a single amino-acid replacement, in which uncontrolled C3b deposition on host tissue led to renal failure, stroke and retinopathy.
The abrupt and rapid expansion of C3b copy number is fuelled by multiple cycles of a positive-feedback loop (Figure 1) in which each nascent C3b molecule promotes conversion of C3 into more C3b molecules. While amplification of C3b can occur in the fluid phase, this is considered largely futile and consumes C3. More importantly, nascent C3b can transiently utilise its thioester group to bind covalently to nearby surfaces. The resulting clusters of surface-deposited C3b molecules trigger further steps in the complement cascade including release of the pro-inflammatory peptide C5a and formation of the membrane-attack complex (MAC).
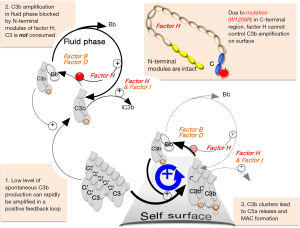
The explosive nature of C3b amplification helps tackle pathogenic microbes that linger only briefly in the bloodstream. But the launch of a lag-free response to diverse threats is risky. It entails spontaneous generation of a trickle of C3b molecules that bind to any nearby surface thus priming it for C3b amplification. This indiscriminate and “always-on” mode of action necessitates the selective protection of self surfaces. Protection is achieved by a set of soluble and membrane-associated complement-regulating proteins. Most of these (4) work by suppressing C3b amplification on self surfaces. Soluble regulators also prevent futile fluid-phase C3 consumption. On damaged or “altered self”, regulators permit controlled C3b deposition and some induce degradation of C3b to iC3b and C3dg, which promote non-inflammatory clearance. Regulators do not block C3b amplification on “nonself”, e.g., microbial surfaces, indeed soluble regulators resist hijack by microbes.
Prominent amongst soluble regulators of spontaneous C3b formation and amplification is the 155-KDa glycoprotein, factor H (FH) (5). While the N-terminal region of FH is responsible for shutting down C3b amplification regardless of context, the C-terminal region ensures FH focuses its regulatory efforts on self surfaces rather than nonself ones (6,7). Ueda et al. graphically demonstrated the severity of disease phenotype that can arise when FH is mutated in a way that permits C3b clusters to form on host-tissue surfaces.
Some cancer cells are recognised as nonself or altered self and are susceptible to complement-mediated attack or clearance (8). To avoid this, cancer cells may overexpress FH and other complement regulators (9,10) or (like some bacteria) may attempt capture of circulating FH for their own protection (11). FH is a possible diagnostic biomarker and potential target for anticancer therapy (12). Autoantibodies from lung cancer patients that targeted the C-terminal region of FH inhibited its binding to lung tumour cells, increased C3b deposition and caused complement-dependent tumour cell lysis (13). This points to a potential anti-FH antibody-based therapy. Conversely, an inflammatory microenvironment favours the development of many tumours. In such cases, cancer cells may be disadvantaged rather than advantaged by the recruitment of FH (14) since its anti-inflammatory properties may suppress tumour growth
FH has 20 modules (Figure 1) and 40 disulfides so it is difficult to produce recombinantly (15). But it is abundant in blood. Its roles are highlighted by the consequences of its absence in FH-knockout mice (16). In FH−/− mice, high levels of C3b, iC3b and C3dg are deposited in glomeruli, and serum C3 levels are depleted due to unrestrained fluid-phase conversion of C3 to C3b. This phenotype resembles C3 glomerulopathy (C3G), a human kidney disease seen in individuals whose FH has mutations, predominantly in its N-terminal modules. People with certain mutations in FH C-terminal modules (17), or mice in which the FH C-terminal region has been deleted (18), are predisposed to atypical haemolytic uraemic syndrome, a different kidney disease that is a form of thrombotic microangiopathy.
Damage arising from a complete absence of FH is moderated by fluid-phase (unregulated) consumption of C3. Conversely, FH with an intact N terminus and a mutated C terminus could be more injurious given that fluid-phase levels of C3 (the precursor of C3b) would be retained, but self surfaces would have lost part of their protection against C3b amplification (Figure 1). Many disease-related mutations cluster in CCPs 19 and 20. In heterozygous individuals, the phenotypic consequences of such a mutation on one allele may be masked by the presence of the “good” allele. And there are other membrane-associated regulators that might be expected to compensate for the loss of FH. Ueda et al. have now revealed the devastating consequences of homozygosity for a C-terminal mutation in FH.
To design their model, they speculated that the aHUS-linked FH mutation W1183R (19) in humans could be recapitulated in murine FH since this Trp residue is conserved between mice and men. In HEK293 cells they made murine CFH with the equivalent mutation, W1206R. They confirmed that this version of FH regulates complement activation in the fluid phase (because it has an intact N terminus) but does not interact with self-surfaces. Then they generated mutant mice, using homologous recombination in C57BL/6J embryonic stem cells. The resultant homozygous animals, carrying the mutation W1206R (FHR/R), were used to assess the consequences.
The severity of the outcome exceeded expectations. At 3–4 weeks of age, the FHR/R mice were smaller and thinner than FHR/W or FHW/W littermates. FHR/R mice carrying this mutation did not just develop renal thrombotic microangiopathy but also developed systemic thrombophilia involving large blood vessels in the brain, liver, lung, spleen and kidney. Nearly one third of the tested mice displayed symptoms of stroke and ischemic retinopathy. The onset of organ thrombosis and renal injury occurred within the first week of life, sudden death was observed on numerous occasions, and just under half of the FHR/R animals (but none of the FHW/R animals) died within 30 weeks.
Presumably in the absence of FH protection on host surfaces, C3b amplification is not fully controlled, despite the presence of other membrane-associated complement regulators. The balance is tipped in favor of amplification, clusters of C3b form and the consequent C5a production and MAC formation causes injury to vascular endothelial and smooth muscle cells, creating a prothrombotic state of the vessel wall. MAC and C5a might also directly activate platelets and neutrophils or monocytes, respectively, leading to release of tissue factor from the stimulated leukocytes, platelet and leukocyte aggregation, and clot formation in both capillaries and large blood vessels.
Severe retinal injury was seen in most FHR/R mice. While there is no known connection between retinopathy and the W1183R mutation in human FH, FH dysfunction has been strongly linked to the pathogenesis of AMD. For example R1210C, at the C-terminus of FH, originally found in some aHUS patients, is a highly penetrant mutation for dry AMD (20). Ueda et al. did not characterize the specific pathology of retinopathy in these animals so could not demonstrate direct complement-mediated attack on retinal cells. The observed retinal injury could be secondary to vascular occlusion.
To establish that the observed phenotype was indeed a consequence of uncontrolled C3b deposition, they crossed their FHR/R mice with mice in which either C3 or factor D (FD; another key protein in the positive-feedback loop for C3b amplification, Figure 1) had been knocked out. The FHR/RC3−/− and FHR/RFD−/− mice had none of the symptoms displayed by their FHR/R littermates. They showed normal kidney function, platelet count and haemoglobin levels, their livers were thrombus free, and they had normal-looking retina and normal retinal blood flow. They lived normally and did not develop stroke symptoms.
The rarity of disease-linked mutations in the N-terminal and C-terminal regions of FH found in C3G and aHUS patients ensure that there are few homozygous cases. Nonetheless, by impairing FH activity on self surface while leaving its fluid-phase regulatory efficacy intact, the authors have revealed a surprising role of FH in preventing macrovascular thrombosis as well as thrombotic microangiopathy and renal disease. The data suggest that dysfunction or variation of FH activity on the cell surface could be implicated in human thrombotic disorders such as stroke and retinal arterial or venous occlusion, in addition to primary or secondary thrombotic microangiopathy. They also suggest that targeting the C terminus with a blocking antibody in cancer therapy could have unwanted consequences.
Acknowledgments
Funding: None.
Footnote
Provenance and Peer Review: This article was commissioned and reviewed by the Section Editor Peipei Xu (Department of Hematology, The Affiliated Drum Tower Hospital of Nanjing, University Medical School, Nanjing, China).
Conflicts of Interest: The author has completed the ICMJE uniform disclosure form (available at http://dx.doi.org/10.21037/tcr.2017.05.24). The author has no conflicts of interest to declare.
Ethical Statement: The author is accountable for all aspects of the work in ensuring that questions related to the accuracy or integrity of any part of the work are appropriately investigated and resolved.
Open Access Statement: This is an Open Access article distributed in accordance with the Creative Commons Attribution-NonCommercial-NoDerivs 4.0 International License (CC BY-NC-ND 4.0), which permits the non-commercial replication and distribution of the article with the strict proviso that no changes or edits are made and the original work is properly cited (including links to both the formal publication through the relevant DOI and the license). See: https://creativecommons.org/licenses/by-nc-nd/4.0/.
References
- Ricklin D, Hajishengallis G, Yang K, et al. Complement: a key system for immune surveillance and homeostasis. Nat Immunol 2010;11:785-97. [Crossref] [PubMed]
- Ricklin D, Lambris JD. Complement in immune and inflammatory disorders: pathophysiological mechanisms. J Immunol 2013;190:3831-8. [Crossref] [PubMed]
- Ueda Y, Mohammed I, Song D, et al. Murine systemic thrombophilia and hemolytic uremic syndrome from a factor H point mutation. Blood 2017;129:1184-96. [Crossref] [PubMed]
- Kirkitadze MD, Barlow PN. Structure and flexibility of the multiple domain proteins that regulate complement activation. Immunol Rev 2001;180:146-61. [Crossref] [PubMed]
- Makou E, Herbert AP, Barlow PN. Functional anatomy of complement factor H. Biochemistry 2013;52:3949-62. [Crossref] [PubMed]
- Ferreira VP, Herbert AP, Hocking HG, et al. Critical role of the C-terminal domains of factor H in regulating complement activation at cell surfaces. J Immunol 2006;177:6308-16. [Crossref] [PubMed]
- Schmidt CQ, Herbert AP, Kavanagh D, et al. A new map of glycosaminoglycan and C3b binding sites on factor H. J Immunol 2008;181:2610-9. [Crossref] [PubMed]
- Mamidi S, Hone S, Kirschfink M. The complement system in cancer: Ambivalence between tumour destruction and promotion. Immunobiology 2017;222:45-54. [Crossref] [PubMed]
- Jurianz K, Ziegler S, Donin N, et al. K562 erythroleukemic cells are equipped with multiple mechanisms of resistance to lysis by complement. Int J Cancer 2001;93:848-54. [Crossref] [PubMed]
- Ajona D, Castano Z, Garayoa M, et al. Expression of complement factor H by lung cancer cells: effects on the activation of the alternative pathway of complement. Cancer Res 2004;64:6310-8. [Crossref] [PubMed]
- Junnikkala S, Jokiranta TS, Friese MA, et al. Exceptional resistance of human H2 glioblastoma cells to complement-mediated killing by expression and utilization of factor H and factor H-like protein 1. J Immunol 2000;164:6075-81. [Crossref] [PubMed]
- Riihila PM, Nissinen LM, Ala-aho R, et al. Complement factor H: a biomarker for progression of cutaneous squamous cell carcinoma. J Invest Dermatol 2014;134:498-506. [Crossref] [PubMed]
- Campa MJ, Gottlin EB, Bushey RT, et al. Complement factor H antibodies from lung cancer patients induce complement-dependent lysis of tumor cells, suggesting a novel immunotherapeutic strategy. Cancer Immunol Res 2015;3:1325-32. [Crossref] [PubMed]
- Bonavita E, Gentile S, Rubino M, et al. PTX3 is an extrinsic oncosuppressor regulating complement-dependent inflammation in cancer. Cell 2015;160:700-14. [Crossref] [PubMed]
- Schmidt CQ, Slingsby FC, Richards A, et al. Production of biologically active complement factor H in therapeutically useful quantities. Protein Expr Purif 2011;76:254-63. [Crossref] [PubMed]
- Pickering MC, Cook HT, Warren J, et al. Uncontrolled C3 activation causes membranoproliferative glomerulonephritis in mice deficient in complement factor H. Nat Genet 2002;31:424-8. [PubMed]
- Kavanagh D, Goodship T. Genetics and complement in atypical HUS. Pediatr Nephrol 2010;25:2431-42. [Crossref] [PubMed]
- Pickering MC, de Jorge EG, Martinez-Barricarte R, et al. Spontaneous hemolytic uremic syndrome triggered by complement factor H lacking surface recognition domains. J Exp Med 2007;204:1249-56. [Crossref] [PubMed]
- Ferreira VP, Herbert AP, Cortes C, et al. The binding of factor H to a complex of physiological polyanions and C3b on cells is impaired in atypical hemolytic uremic syndrome. J Immunol 2009;182:7009-18. [Crossref] [PubMed]
- Raychaudhuri S, Iartchouk O, Chin K, et al. A rare penetrant mutation in CFH confers high risk of age-related macular degeneration. Nat Genet 2011;43:1232-6. [Crossref] [PubMed]