Radiobiological characterization of the very high dose rate and dose per pulse electron beams produced by an IORT (intra operative radiation therapy) dedicated linac
IntroductionOther Section
IORT is a cancer treatment modality in which a large radiation dose is delivered to the target volume in a single fraction at the time of the surgical resection of the tumour. It allows for tumour bed sterilization or for a direct irradiation of the tumour, when it cannot be entirely resected. Recently, a renewed interest on this technique, which was proposed for the first time at the beginning of last century (1), has grown following the development of dedicated small linear accelerators that can be placed in the operating theatre and easily moved from one room to another. Hence, treatments are made possible in a delicate environment, such as a sterilised room, coping at the same time with the radioprotection aspects concerning the medical staff. Consequently, a notable increase in terms of number of treated patients, new clinical protocols and scientific publications has ensued (2).
IORT rationale, technique and some radiobiological aspects related mainly to its peculiarity to deliver the entire treatment in a single large dose have been discussed in detail in some review papers (3-6). Briefly, the advantages of such a technique with respect to the conventional radiotherapy (irradiation with external beams and with fractionated doses) can be mainly summarized as follow: potential improvement of the tumour local control, reduced absorbed dose delivered to normal tissues, a gain in the therapeutic ratio, namely the index for tumour response for a fixed level of normal tissue damage. Furthermore, prolonged radiation treatments can be avoided after surgery. This is accomplished by defining the treatment target once in the surgical room and by the possibility of directly displacing or shielding healthy tissues hence achieving a well-conformed irradiation.
The very high dose rate allows for treatments to be completed within a few tens of seconds (a total dose of about 20 Gy is delivered in approximately 1 minute), which is a very important feature as anesthetized patients are treated. The beams produced by dedicated accelerators are characterizes by a high entrance dose and a low photon contamination, resulting in a good coverage of the target (typically, for an IORT treatment, the target begins at the surface of the patient), and in a reduced dose to healthy tissue behind the target. In the case of the Novac7 accelerator (Hitesys SpA, Italy), for example, the entrance dose is around 90% and the photon contamination less than 1% (7).
The main limit of the IORT resides in the impossibility to develop a plan before the treatment itself. Since the target volume is defined directly in the operating theatre, just before the irradiation, the electron beam energy, the beam collimator, named “applicator”, and the total dose have to be chosen at that very moment of the surgery to ensure the best target dose distribution.
The calculation of the monitor units needed to deliver the prescribed dose to the patient is based on measurements performed in reference conditions, according to (8). The only commercial treatment planning system dedicated to the IORT (9) is based merely on the CT images of the patient acquired in the preparatory phase (pre-surgery), and its use is limited given the morphology changes of the tissue to be irradiated resulting from the surgical operation. For this reason, dosimetry in reference conditions assumes a crucial role.
Concerning the biological effects due to dose rates, they have been clearly assessed in the range (0.01–10) Gy/min in terms of repair of sub-lethal damage (10). At higher dose rate, some pioneering studies in the 1960s (11,12) showed some reduced biological effect due to the high dose rate, which could be explained with oxygen consumption by radiation and the important free radical formation due to the high dose rate giving rise to a probable recombination. Nias et al. showed instead no differences in radiosensitivity of HeLa cells after irradiation with 10 ns electron pulses (13).
More recent studies are difficult to compare, owing to their different irradiation conditions. For example, Schulz et al. (14) studied the clonogenic survival of Chinese hamster lung cell after 250 kV X-rays and a pulsed 30 MeV electron beam with a dose rate in the range (0.5–5) ×108 Gy/s. They found the electron beam biologically less effective than X-rays, but they could not relate their results to oxygen consumption. In similar experimental condition [35 MeV electron beam and (0.25–2.5) ×108 Gy/s]. Purdie et al. (15) found opposite results. They measured a significant decrease in human kidney cell survival as a function of the dose rate both comparing γ-rays irradiation at different dose rate, and comparing the reference radiation to the pulsed electron beam. Early skin reaction in mouse has also been studied (16) with the result of an increased biological effect up to the dose rate of 0.96×108 Gy/s followed by a decrease at even higher values, probably due to oxygen effects. Zackrisson et al. (17) did not find any significant difference in the RBE in their study of clonogenic survival of V-79 cell line performed with 50 MeV electrons and 3.8×102 Gy/s mean dose rate and reference radiation. With the modern remarkable development of the laser acceleration of particles for potential application in radiotherapy, even higher pulse dose rates have very recently become available (dose rate ≥109 Gy/s). Electrons accelerated with this technique have been tentatively radiobiologically characterised and no differences have been found thus far with respect to electrons accelerated by a conventional Linac (18). The biological effectiveness of laser driven protons has also been investigated on clonogenic survival with similar results using the V-79 cell line by Doria et al. at dose rates exceeding 109 Gy/s as a single exposure (19).
In conclusion, a coherent picture does not exist on the biological effects of electron beams in condition of very high dose per pulse and high dose rate, particularly as far as the clinical ranges are concerned.
In an IORT treatment, if the RBE is a function of the dose rate and/or the dose per pulse, it will depend on the energy selected during the surgical operation, according to the depth of the tissue target, and on the applicator, chosen according to the lateral dimensions of the lesion. In fact the dose rate and the dose per pulse produced in a IORT treatment vary with the employed energy and applicator (20).
On the other hand, RBE is prescribed only on the basis of the tumour cell type. Therefore, the knowledge of the dependence of the RBE as a function of the dose rate and/or the dose per pulse is interesting from a scientific point of view, and, even more, from the clinical point of view, when an intra-operative radiation treatment is performed with dedicated Linacs.
In this framework, we characterised an IORT beam delivered by the Novac7 accelerator, measuring, at different dose rates and doses per pulse, the clonogenic survival of the MCF7 cell line, a tumour cell line from breast tissue, being early breast carcinoma one of the typical pathology treated with IORT.
As a comparison with the standard breast cancer radiotherapy treatment, we have also experimental investigated the RBE of a conventional 6-MeV electron beam (reference electron beam) and a conventional 6-MV X-rays (reference beam used for the standard breast cancer radiotherapy treatment).
MethodsOther Section
Cell lines and cultures
For the radiobiological characterisation of the IORT beams we chose the MCF-7 cell line. It is derived from a malignant adenocarcinoma of the breast tissue of a Caucasian woman. Cells were grown in D-MEM:F12 supplemented with 5% foetal bovine serum, 0.7% antibiotics, and 0.5% fungizone. Under these conditions, the Plating Efficiency (PE) was ~80%, and the doubling time, evaluated from the growth curve, was Td =(30±2) h. At least 24 h prior to exposure to photons or electrons, cells were seeded on standard T12.5 tissue culture flasks at about 105 cells flask so that a cell monolayer was formed by the time of irradiation.
Irradiation facilities
The IORT accelerator used for our study is the Novac7 (Hytesis, Italy) in operation at the Azienda Ospedaliero-Universitaria Pisana in Pisa (Italy). It is a mobile Linac, which can deliver pulsed electron beams with nominal energy of 3, 5, 7 and 9 MeV. The characteristics of the Novac7 are very different with respect to the conventional Linacs used for standard radiotherapy: its head is devoid of magnetic lenses and scattering foils, and the collimation and the flatness of the electron beams are defined by means of special PMMA tools of cylindrical shape, named applicators (21). As a consequence, the dosimetric characteristics of the produced beams are quite peculiar. In particular, the dose rate and the dose per pulse are larger than those produced by conventional Linacs (22): the dose per pulse varies with the energy and the applicator type in the range from 3 to 13 cGy/pulse resulting in a clinical dose rate in the range from 9 to 39 Gy/min, being 5 Hz the pulse frequency in the clinical conditions.
For comparison, irradiations were also performed with a conventional radiotherapy linear accelerator (Clinac® DHX, Varian), providing a 6 MeV pulsed electron beam and 6 MV X-rays.
The Novac7 linac allows to vary the pulse frequency in the range 1–30 Hz (not necessarily for clinical practice). As a consequence, it is possible to obtain different values of dose-rate at a fixed value of dose per pulse.
Dosimetry
To optimize dosimetry and the uniformity of the cell dose distribution, the cell monolayer was irradiated at the maximum dose depth of a solid water layer. The flasks were completely filled with the cell culture medium, and placed into a special PMMA phantom, conveniently designed (Figure 1). A build-up layer of solid water was then positioned on the top of the flasks. The cell absorbed dose was measured with Gafchromic EBT films, directly positioned inside the same type of flasks used for the cell irradiations; the procedure of calibration, irradiation and post-irradiation analysis of the Gafchromic films is described in (7,20,23). The accuracy on the dose measurements was 3%.
The correction factor accounting for possible changes of the accelerator output between the Gafchromic film irradiation and the cells irradiation was quantified performing dose measurements with a Markus ionization chamber before each irradiation. In the case of conventional beams the factor was found negligible (<0.5%), while in the case of the IORT beams it resulted of the order of 7%, introducing an additional dosimetric uncertainty of about 3% (20,21,24,25). Accordingly, the estimated overall dose uncertainty was around 3% for conventional beams and around 5% for the IORT ones.
Irradiations
The nominal energy selected for the electron beam from the Novac7 Linac was 7 MeV, and irradiations were performed at the dose rates of 5, 25, and 75 Gy/min using the 6 cm diameter applicator (dose per pulse =7.4 cGy/pulse), and at the dose rates of 25 and 75 Gy/min using the 10 cm diameter applicator (dose per pulse =4.2 cGy/pulse).
The reference irradiations (electrons and X-rays) from the conventional radiotherapy linear accelerator were carried out at the dose rate of 2 Gy/min and at a dose per pulse of 0.1 cGy/pulse. Electrons were considered as the reference clinical electron beam, while the X-rays were the reference of the present work, since they are used for the conventional treatment of the mammary carcinoma.
Each experimental cell survival curve was obtained by delivering doses of 0.5, 1, 1.5, 2, 3, 4 and 5 Gy. All the irradiation parameters are summarised in Table 1.
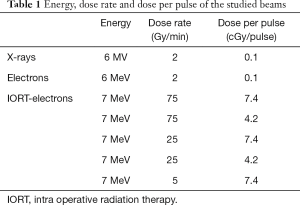
Full table
Cell inactivation and analysis of survival data
Cell inactivation was measured by assessing loss of reproductive potential by means of a standard clonogenic assay. After irradiation, cells were trypsinized, diluted and counted to plate three replicates per dose for colony formation. According to the expected dose-dependent decrease in surviving fraction, inoculated cell numbers ranged between 250 and 80,000 in order to obtain between 100–200 colonies per disk/flask. After a period of incubation of 10 days at 37 °C in a 5% CO2 atmosphere, colonies were fixed in methanol for 5 minutes at room temperature and then stained for 30 min by Giemsa solution. Cells were considered as survivors if they had formed a clone containing at least 50 cells (25).
Two independent experiments were carried out for each curve at different dose rates or different doses per pulse. The surviving fractions S(D) were determined as the ratio between the measured plating efficiency at dose D, PE(D), normalised to the PE at the zero dose. The surviving fraction S(D) at each dose was then evaluated as the mean of the results of the independent experiments with its standard error. Data were fitted with a linear quadratic model according to the formula:
S(D) = exp (−
ResultsOther Section
The survival curves corresponding to the reference irradiations (6 MV X-rays and 6 MeV conventional electrons) are reported in Figure 2.
For the typical radiotherapy dose rate (2 Gy/min), electrons turned out to be less effective than X-rays. In particular, the α parameter, reported in Table 2 together with the fitting parameters of all the measured survival curves, was larger for the X-ray survival curve with respect to that of conventional electrons, while the β parameters were within the experimental errors.
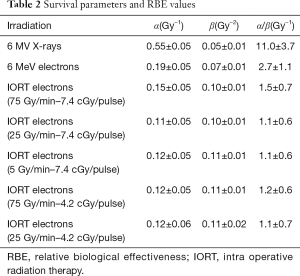
Full table
In Figure 3 we report the results of the different irradiations at the IORT dedicated Linac, performed at the dose per pulse of 7.4 cGy/pulse together, with the dose-response curve for the conventional electrons. The α fit parameters presented almost the same value for all the measured curves, within the experimental errors.
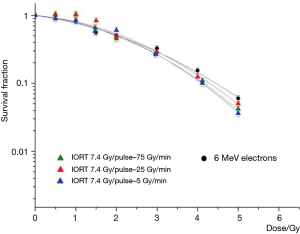
Nevertheless, a slight increase of the β-value for the high dose rate IORT beams with respect to the conventional electrons was present, according to the fit parameters reported in Table 2. No difference in effectiveness was found between the IORT beams by varying the dose rate.
In Figure 4 the results of the dose response survival curves of IORT beams are reported together with those corresponding at the same dose rate, but at different dose per pulse (4.2 and 7.4 cGy/pulse) to evaluate the possible effect related to the dose per pulse. Similarly, no differences in the beam effectiveness emerged that are attributable to the dose per pulse variations.
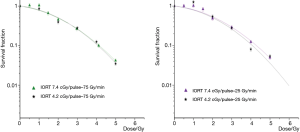
DiscussionOther Section
At the typical radiotherapy dose rate (2 Gy/min), electrons turned out to be less effective than the 6 MV X-rays. In particular, the α parameter was larger for the X-ray survival curve with respect to that of conventional electrons, while the β parameters were within the experimental errors.
This can be related to the different energy spectra of the two radiations. The 6 MeV electron energy spectrum shows a continuously increasing trend up to a peak representing the most probable beam energy (around 6 MeV) followed by a quite sharp decrease as discussed in (6), where the simulated energy spectra of the electrons are reported at the exit of collimator. On the other hand, the 6 MV X-ray energy spectrum is characterized by a peak at low energy followed by a continuous decrease before reaching the nominal energy as shown in Figure 5, where the photon energy spectrum with a nominal energy of 6 MV, simulated with EGSnrc/BEAMnrc Monte Carlo package (26), is reported for a 10×10 cm2 field (reference field for dosimetry in our measurements). The peak, representing the most probable energy is around 0.5 MeV, while the average energy is around 1.7 MeV. For completeness in Figure 5 we also show the simulated energy spectrum for the 7 MeV electrons from the Novac7 accelerator (7).
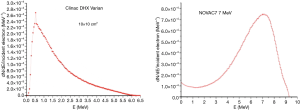
From a dosimetric point of view, the relevant point is the energy spectrum of the secondary electrons from the indirectly ionizing X-ray reference beam, whose energy spectrum results further degraded with respect to the primary radiation. The linear energy transfer (LET) of electrons in water as a function of energy remains almost constant in the energy range from 1 to 10 MeV, after an initial decrease. Consequently, the average LET exhibited by the secondary electrons from the X-rays was bigger than the one of the 6 MeV conventional electrons, resulting in an increase in the biological effectiveness of the reference radiation. It is then reasonable to assume that the higher LET of that radiation accounts for the increased experimental value of the α parameter of the X-ray survival curve.
Concerning the different irradiations at the IORT dedicated Linac, the survival curves show almost the same value for the α fit parameter, within the experimental errors.
Indeed, α is closely related to the lethal intra-track effects of radiation, hence to the LET, which in turn depends on the energy, and the energy spectrum of the 7 MeV IORT beams is quite similar to that of the conventional electron beam used in this work (7,27).
The slight increase of the β-value for the high dose rate IORT beams with respect to the conventional electrons can be explained by considering that cell damage due to the contribution of two-track events becomes more likely for high dose rate irradiations. A similar trend was not observed as a function of the different dose rates at the IORT beams, probably due to saturation effects. From the therapeutic point of view, it is important to note that the choice of the applicator and/or the energy during an IORT treatment affecting the dose rate and the dose per pulse, does not seem to change the radiobiologic characteristics of the beam.
An analogous conclusion can be drawn for the irradiations performed at the dose per pulse values of 4.2 cGy/pulse, and dose rates of 75 and 2 Gy/min where no differences attributable to the dose per pulse variations emerged.
ConclusionsOther Section
We presented a study for the radiobiological characterization of an electron beam produced by the Novac7 Linac (Hytesis SpA, Italy) employed for Intra Operative Radiation Therapy. The clonogenical essay of the MCF-7 cell line was performed for different dose rates at two values of dose per pulse, covering the typical values of dose rate and dose per pulse clinically used. X-rays and electrons from a conventional Linac accelerator for radiotherapy were also considered as references. We found the 6 MV X-rays be more effective with respect to the 6 MeV electrons, as the former radiation quality exhibits a more degraded energy spectrum for the secondary electrons. This suggests the need to perform new experiments to better compare the RBE of an IORT treatment (performed with electron beams in a single dose fraction) with those of the standard radiotherapy treatment.
Concerning the IORT beam exposures, the survival curves did not show any dependence on the dose-rate and the dose per pulse in the parameter range for the clinical exploitation of the Novac7 beams. This is an important clinical finding, as it means that changes in the use of the applicators and of the employed energy, with the corresponding changes in the dose rate and dose per pulse, do not seem to affect the radiobiologic properties of the beam.
The comparison with the conventional electron irradiations showed no differences as far as the α parameter is concerned, while slight different β parameters were found. This was expected since the energy spectra of the different beams were almost equivalent from the dosimetric point of view, and suggests a saturation effect at dose rate and dose per pulse values lower than those in use in the IORT.
AcknowledgmentsOther Section
Funding: This work was financially supported by the Grant BIORT by Istituto Nazionale di Fisica Nucleare (INFN).
FootnoteOther Section
Provenance and Peer Review: This article was commissioned by the Guest Editors (Marco Durante, Giusi I. Forte, Giorgio Russo) for the series “Radiobiological models towards a personalized radiation oncology” published in Translational Cancer Research. The article has undergone external peer review.
Conflicts of Interest: All authors have completed the ICMJE uniform disclosure form (available at http://dx.doi.org/10.21037/tcr.2017.05.21). The series “Radiobiological models towards a personalized radiation oncology” was commissioned by the editorial office without any funding or sponsorship. The authors have no other conflicts of interest to declare.
Ethical Statement: The authors are accountable for all aspects of the work in ensuring that questions related to the accuracy or integrity of any part of the work are appropriately investigated and resolved. The study was conducted in accordance with the Declaration of Helsinki (as revised in 2013). Institutional ethical approval and informed consent were waived.
Open Access Statement: This is an Open Access article distributed in accordance with the Creative Commons Attribution-NonCommercial-NoDerivs 4.0 International License (CC BY-NC-ND 4.0), which permits the non-commercial replication and distribution of the article with the strict proviso that no changes or edits are made and the original work is properly cited (including links to both the formal publication through the relevant DOI and the license). See: https://creativecommons.org/licenses/by-nc-nd/4.0/.
ReferencesOther Section
- Comas C, Prio A. Irradiation roentgen intra-abdominale, après intervention chirurgicale dans un cas de cancer de l’uterus, Congres International d’Electrologie, Imprenta Francesca Badia, Barcelona, 1907:5-14.
- Gunderson LL, Willet CG, Harrison LB, et al. Intraoperative Irradiation: Techniques and Results - Second Edition. Totowa, New Jersey: Humana Press, 2011.
- Valentini V, Balducci M, Tortoreto F, et al. Intraoperative radiotherapy: current thinking. Eur J Surg Oncol 2002;28:180-5. [Crossref] [PubMed]
- Calvo FA, Meirino RM, Orecchia R. Intraoperative radiation therapy part 2. Clinical results. Crit Rev Oncol Hematol 2006;59:116-27. [Crossref] [PubMed]
- Herskind C, Ma L, Liu Q, et al. Biology of high single doses of IORT: RBE, 5 R's, and other biological aspects. Radiat Oncol 2017;12:24. [Crossref] [PubMed]
- Willett CG, Czito BG, Tyler DS. Intraoperative radiation therapy. J Clin Oncol 2007;25:971-7. [Crossref] [PubMed]
- Righi S, Karaj E, Felici G, et al. Dosimetric characteristics of electron beams produced by two mobile accelerators, Novac7 and Liac, for intraoperative radiation therapy through Monte Carlo simulation. J Appl Clin Med Phys 2013;14:3678. [Crossref] [PubMed]
- Beddar AS, Biggs PJ, Chang S, et al. Intraoperative radiation therapy using mobile electron linear accelerators: report of AAPM Radiation Therapy Committee Task Group No. 72. Med Phys 2006;33:1476-89. [Crossref] [PubMed]
- Available online: http://www.gmv.com/en/Healthcare/radiance/
- Hall EJ. Radiobiology for Radiologist, 5th Ed. Lippincott Williams & Wilkins, 2000:66-74.
- Town CD. Radiobiology. Effect of high dose rates on survival of mammalian cells. Nature 1967;215:847-8. [Crossref] [PubMed]
- Berry RJ, Hall EJ, Forster DW, et al. Survival of mammalian cells exposed to x rays at ultra-high dose-rates. Br J Radiol 1969;42:102-7. [Crossref] [PubMed]
- Nias AH, Swallow AJ, Keene JP, et al. Survival of HeLa cells from 10 nanosecond pulses of electrons. Int J Radiat Biol Relat Stud Phys Chem Med 1970;17:595-8. [Crossref] [PubMed]
- Schulz RJ, Nath R, Testa JR. The effects of ultra-high dose rates on survival and sublethal repair in Chinese-hamster cells. Int J Radiat Biol Relat Stud Phys Chem Med 1978;33:81-8. [Crossref] [PubMed]
- Purdie JW, Inhaber ER, Klassen NV. Increased sensitivity of mammalian cells irradiated at high dose rates under oxic conditions. Int J Radiat Biol Relat Stud Phys Chem Med 1980;37:331-5. [Crossref] [PubMed]
- Inada T, Nishio H, Amino S, et al. High dose-rate dependence of early skin reaction in mouse. Int J Radiat Biol Relat Stud Phys Chem Med 1980;38:139-45. [Crossref] [PubMed]
- Zackrisson BU, Nyström UH, Ostbergh P. Biological response in vitro to pulsed high dose rate electrons from a clinical accelerator. Acta Oncol 1991;30:747-51. [Crossref] [PubMed]
- Laschinsky L, Baumann M, Beyreuther E, et al. Radiobiological effectiveness of laser accelerated electrons in comparison to electron beams from a conventional linear accelerator. J Radiat Res 2012;53:395-403. [Crossref] [PubMed]
- Doria D, Kakolee KF, Kar S, et al. Biological effectiveness on live cells of laser driven protons at dose rates exceeding 109 Gy/s. AIP Advances 2012;2:011209 [Crossref]
- Di Martino F, Giannelli M, Traino AC, et al. Ion recombination correction for very high dose-per-pulse high-energy electron beams. Med Phys 2005;32:2204-10. [Crossref]
- Ciocca M, Cantone MC, Veronese I, et al. Application of failure mode and effects analysis to intraoperative radiation therapy using mobile electron linear accelerators. Int J Radiat Oncol Biol Phys 2012;82:e305-11. [Crossref] [PubMed]
- Galimberti V, Ciocca M, Leonardi MC, et al. Is electron beam intraoperative radiotherapy (ELIOT) safe in pregnant women with early breast cancer? In vivo dosimetry to assess fetal dose. Ann Surg Oncol 2009;16:100-5. [Crossref] [PubMed]
- Karaj E, Righi S, Di Martino F. Absolute dose measurements by means of a small cylindrical ionization chamber for very high dose per pulse high energy electron beams. Med Phys 2007;34:952-8. [Crossref] [PubMed]
- Laitano RF, Guerra AS, Pimpinella M, et al. Charge collection efficiency in ionization chambers exposed to electron beams with high dose per pulse. Phys Med Biol 2006;51:6419-36. [Crossref] [PubMed]
- Cella L, Liuzzi R, Salvatore M. The Italian affair: the employment of parallel-plate ionization chambers for dose measurements in high dose-per-pulse IORT electron beams. Med Phys 2010;37:2918-24. [Crossref]
- Kawrakow I, Rogers DW. The EGSnrc code system: Monte Carlo Simulation of Electron and Photon Transport. National Research Council of Canada Report No. PIRS-701 2006.
- Ding GX, Rogers DW. Energy spectra, angular spread and dose distributions of electron beams from various accelerators used in radiotherapy, National Research Council of Canada, Report No PIRS-0439, Ottawa (1995). Available online: http://www.irs.inms.nrc.ca/inms/irs/papers/PIRS439/pirs439.html