Inhibiting C5a/C5aR axis reduces myeloid-derived suppressor cells and enhances PD-1 blockade therapy in lung cancer
PD-1 immune checkpoint blockade therapy in lung cancer
In the US (1), and the world, lung cancer remains the leading cause of cancer death. Lung cancer causes more deaths than the next three most common cancers of the colon, breast and prostate combined. In the US an estimated 160,000 Americans and worldwide more than 1.1 million people die from lung cancer annually (2). Approximately 220,000 new cases occur every year in the US. Although previously thought to be non-immunogenic and non-responsive to immune-based therapies, recent ground-breaking studies in lung cancer with immune checkpoint blockade therapy reveal robust anti-tumor activity and durable responses in previously treated patients with progressive locally advanced or metastatic non-small cell lung cancer (NSCLC). This has also changed the treatment paradigm leading to immune checkpoint blockade as a frontline therapy for advanced stage lung cancer. Studies in NSCLC and melanoma patient-derived tumor specimens reveal that responses to immune checkpoint blockade therapy rely on tumor infiltration of activated T effector cells (3-7). Immune inhibitory molecules are upregulated on activated T cells in tumors causing a down regulation of anti-tumor activity. The programmed cell death protein 1 (PD-1; also known as CD279) is an inhibitory receptor that regulates immune responses. The PD-1 receptor interaction with the PD-L1 and PD-L2 ligands deliver inhibitory signals that regulate the balance between T cell activation and tolerance. Recent studies reveal responses in approximately 20% of NSCLC patients treated with inhibitors of the PD-1 checkpoint (3-6,8,9). Progress in our understanding of immune biology provide insights on the multiple pathways that modulate T cell and antigen presenting cell activities, hence PD-1/PD-L1 blockade alone is insufficient to address and mitigate all the deficiencies that contribute to the down regulation of anti-tumor immune responses in the tumor microenvironment (TME). This has led to concerted efforts into evaluating rational combinations with radiation therapy, chemotherapy, targeted therapy or vaccines to improve durable complete responses in cancer patients treated with PD-1/PD-L1 blockade therapy. Early investigations are encouraging suggesting that some of the combinations are more beneficial than monotherapy alone. With continued support of these research endeavors it is anticipated that increased immune based treatment options will be available for lung cancer. A number of combinations with PD-1/PD-L1 are under investigation for various cancer types. In this editorial we will focus on the combined efficacy of complement peptide C5a signaling inhibition and PD-1 blockade in preclinical lung cancer models based on the observations of Ajona et al. (10).
The complement system
The complement system is part of innate immunity consisting of at least 30 distinct plasma proteins that when activated opsonize pathogens, induce a series of inflammatory responses and form the membrane attack complex (MAC) that culminate in the elimination of pathogens. Much of the understanding of complement activation is derived from studies on pathogen clearance that defined three distinct mechanisms of complement activation on the pathogen surface that include the classical, alternative and the lectin pathways. Although these pathways are initiated by different molecules, they converge to generate the same set of effector molecules. The classical pathway is initiated during an adaptive immune response by the binding of C1q to Fc portion of the antibody-antigen complexes on targets, which links the effector mechanisms of innate and adaptive immunity. The alternative pathway is initiated by a spontaneously activated complement component C3 that binds to the surface of a pathogen. Finally the mannan-binding lectin pathway is initiated by binding of the mannan-binding lectin, a serum protein, to mannose-containing carbohydrates on bacteria or viruses. Each pathway follows a sequence of reactions to generate a protease called C3 convertase. These reactions are known as the ‘early’ events of complement activation, and consist of triggered-enzyme cascades in which inactive complement proteins are successively cleaved to yield two fragments, the larger of which is an active serine protease. The active protease is retained at the pathogen surface, and this ensures that the next complement protein in the pathway is also cleaved and activated at the pathogen surface. Hence C3b acts as an opsonin that enhances the phagocytosis of the C3b bound targets. By contrast, the small peptide fragments C3a, C4a and C5a are released from the site of the reaction and act as soluble mediators of inflammation. The small complement fragments C3a, C4a and C5a are differentially active: C5a is more active than C3a, which is more active than C4a. C3a, C4a and C5a cause local inflammatory responses by acting directly on local blood vessels, stimulating increased: blood flow, vascular permeability, and binding of phagocytes to endothelial cells. In addition C5a and C3a activate mast cells to release histamine and TNF-α that contribute to the inflammatory response. C5a directly increases the activity of the phagocytes through the G protein C5a receptor expressed by macrophages and neutrophils. On the other hand, the membrane bound complement proteins C5b-C9 form the MAC on targets that lead to their lysis.
Complement activation in lung cancer
Genetic and epigenetic changes that lead to the formation of malignant cells cause alterations in the cell surface glyco- and lipoproteins that activate the complement cascade. Several reports support the capacity of complement to recognize malignant cells. For example lung cancer cell lines deposit C5 and generate C5a more efficiently than bronchial epithelial cells (11). Moreover, a significant increase in C5a was found in the plasma samples of patients with NSCLC, suggesting that the local generation of C5a within tumors may be followed by its systemic diffusion (11). Elevated levels of the complement activation product C4d in bronchial fluids allows for the diagnosis of lung cancer (12). In primary lung tumors, C3c can be detected by immunohistochemistry (13). C3c and C4 are elevated in patients with lung cancer (14) and complement levels correlate with tumor size (15). On the other hand, the capacity of lung cancer cell lines to produce C5a in the absence of an exogenous source of complement components (i.e., serum), suggests that, apart from the traditional pathways of complement activation, cancer cells may have the capacity to activate complement by an extrinsic activation mechanism (11). The production of anaphylatoxin C5a by cancer cells may be mediated by soluble and membrane-bound pro-teases, such as serine proteases of the coagulation and fibrinolysis systems or cell-bound proteases (16-18). The question that these observations raise is that if complement is activated in the TME, why does it not lead to tumor clearance? Studies have shown that malignant tumor cells are protected from such complement-mediated lysis because they over express complement regulators that limit complement activation and deposition in situ and, therefore, formation of the MAC (19,20). It has been postulated that the ability of the MAC to lyse foreign and host cells might enhance the efficacy of cancer immunotherapies involving monoclonal antibodies (mAbs) specific for particular tumor antigens, as complement proteins enhance antibody-dependent cytotoxicity (21,22). However it is now being realized that the peptide anaphylatoxin complement-activation product C5a is involved in promoting tumor growth.
C5a-mediated immune suppression in lung cancer
The TME consists of tumor cells, stroma, blood vessels, immune infiltrates and the extracellular matrix. Genetic alterations in oncogenes and tumor suppressor genes or epigenetic changes in the tumor that modulate tumor growth and invasion into the surrounding tissue orchestrate the persistence of inflammatory infiltrates. These cellular infiltrates modulate tumor development and progression. The tumor infiltrates vary by size and composition in diverse tumor types and at different stages of tumor development. Contributing to the immune suppression in the TME are myeloid-derived suppressor cells (MDSC). Studies in murine lung cancer models have shown that the anaphylatoxin C5a in the TME recruits monocytes. The tumor then programs the monocytes to become MDSC, thus sustaining a dysregulated inflammation that promotes tumor progression (23). C5a may have a key role as a chemoattractant for a subpopulation of MDSC that is morphologically related to neutrophils polymorphonuclear MDSC and as an activator of the production of reactive oxygen and nitrogen species in the monocyte-like subpopulation (23) that inhibit T cell responses. The role of C5a in the immunosuppressive function of MDSC was confirmed ex vivo when isolated MDSCs from C5aR-deficient mice were unable to inhibit T-cell proliferation (23). Moreover, in the LLC lung cancer mouse model, the blockade of C5a signaling reduced MDSC and down regulated the expression of key immunosuppressive molecules ARG1, IL-10, IL-6, CTLA4, LAG3, and PDL1 within the tumors (11). MDSC negatively modulate immune responses and promote tumor progression and metastases (24). MDSC are a heterogeneous population of immature myeloid cells that consists of myeloid progenitors and precursors of macrophages, granulocytes and dendritic cells. In mice, MDSC are identified by antibodies that detect cell surface expression of Gr1 and CD11b. Increases in the number of MDSC evoke strong natural suppressive activity in cancer patients or tumor-bearing mice. Gr1+CD11b+ immune suppressive cells are capable of inhibiting the T cell proliferative response induced by alloantigens, CD3 ligation, or various mitogens, and can also inhibit IL-2 utilization as well as NK cell activity. These observations indicate that progressive tumor growth is associated with the down-regulation of T cell responses and that the MDSC are involved in negative immunoregulatory mechanisms. In murine tumor models, increases in MDSC in the tumors, spleens, bone marrow and blood down regulate T cell responses (24). The immune modulatory role of MDSC in lung cancer was shown in a recent study, demonstrating that MDSC depletion augments anti-tumor activity in lung cancer (25).
Combined inhibition of C5a/C5aR and PD-1 enhances anti-tumor responses in lung cancer
Ajona et al. in their publication “A Combined PD-1-C5a Blockade Synergistically Protects Against Lung Cancer Growth and Metastasis” hypothesized that a combined inhibition of C5a/C5aR1 and PD-1 signaling may have an anti-tumor synergistic effect. RMP1-14 antibody was used to block PD-1 and an L-aptamer to inhibit signaling of complement C5a with its receptor C5aR. The authors rationale to target C5a was to down regulate immune suppression mediated by MDSC as C5a contributes to lung cancer progression by promoting an immunosuppressive microenvironment in which MDSC are involved. Utilizing several syngeneic models of lung cancer the authors demonstrated that the combination of C5a and PD-1 blockade markedly reduces tumor growth and metastasis, and leads to prolonged survival. As depicted in Figure 1, the anti-tumor effect was accompanied by a reciprocal alteration in the frequency of CD8 T cells and MDSC within the tumors, which they suggested may result in a more complete reversal of CD8 T-cell exhaustion. Based on the experimental results the authors concluded that C5a blockade creates a more permissive environment for immune-mediated tumor rejection and, consequently, its combined administration with anti-PD-1 antibodies synergistically impairs lung cancer growth and metastasis.
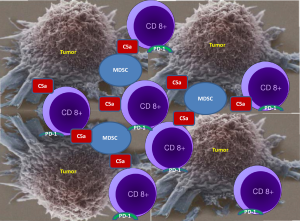
Commentary
With the success of PD-1 immune checkpoint blockade therapy for cancer, it is important to understand the role of complement activation in response to therapy. Although activated complement proteins have the potential to inhibit tumor growth, it is now being realized that the complement-activation peptide C5a is involved in promoting tumor growth in the context of recruitment/activation of MDSC. MDSC are key immune suppressive cells that down regulate T cell activity in the TME. In addition to mAb therapy induced complement activation, neoplastic transformation is accompanied by an increased capacity of the malignant cells to activate complement. However inhibitory mechanisms of complement activation allow cancer cells to escape from complement-mediated elimination and impede the clinical efficacy of mAb-based cancer immune therapies. PD-1/PD-L1 blockade is not able to reduce the T cell suppressive activity of the TME, which is induced by the accumulation of MDSC. Blocking C5a signaling reduces MDSC in the TME and synergizes with PD-1 blockade therapy in lung cancer. The study by Ajona et al. provides support for further evaluation of anti-PD-1 and anti-C5a agents as a novel combination therapeutic strategy for lung cancer. Studies are warranted to determine the generality of Ajona et al. observations for the broad applicability of the combined approach. Challenges that need to be overcome for the overall clinical success of this combinatorial approach, include appropriate scheduling, dosage, sequencing of the therapeutic agents and manageable toxicity profiles. In addition further analysis of the pathways by which cancer cells activate complement and induce immune suppression will provide insights on the interplay between complement and cancer and identify new diagnostic biomarkers and molecular targets for combined immune based anti-cancer therapies.
Acknowledgments
Funding: Drs. Sharma and Dubinett are supported by the University of California Los Angeles Lung Cancer Program, Department of Veterans Affairs Medical Research Funds, National Institutes of Health (NIH) Grants (RO1 CA95686, RO1 CA126944 and P50 CA90388), National Center for Advancing Translational Sciences, Grant UL1TR000124, and Tobacco Related Disease Program Award Program of University of California (15RT-0207 and 20FT0082). The content is solely the responsibility of the authors and does not necessarily represent the official views of the NIH. The funders had no role in decision to publish, or preparation of the editorial. The authors do not have interest (equity holdings or stock options) in any corporate entity dealing with the material or the subject matter of this contribution.
Footnote
Provenance and Peer Review: This article was commissioned and reviewed by the Section Editor Wei Xu (Division of Respiratory Disease, Department of Geriatrics, the First Affiliated Hospital of Nanjing Medical University, Nanjing, China).
Conflicts of Interest: Both authors have completed the ICMJE uniform disclosure form (available at http://dx.doi.org/10.21037/tcr.2017.06.08). The authors have no conflicts of interest to declare.
Ethical Statement: The authors are accountable for all aspects of the work in ensuring that questions related to the accuracy or integrity of any part of the work are appropriately investigated and resolved.
Open Access Statement: This is an Open Access article distributed in accordance with the Creative Commons Attribution-NonCommercial-NoDerivs 4.0 International License (CC BY-NC-ND 4.0), which permits the non-commercial replication and distribution of the article with the strict proviso that no changes or edits are made and the original work is properly cited (including links to both the formal publication through the relevant DOI and the license). See: https://creativecommons.org/licenses/by-nc-nd/4.0/.
References
- Jemal A, Siegel R, Ward E, et al. Cancer Statistics, 2007. CA Cancer J Clin 2007;57:43-66. [Crossref] [PubMed]
- Jemal A, Siegel R, Ward E, et al. Cancer statistics, 2009. CA Cancer J Clin 2009;59:225-49. [Crossref] [PubMed]
- Herbst RS, Soria JC, Kowanetz M, et al. Predictive correlates of response to the anti-PD-L1 antibody MPDL3280A in cancer patients. Nature 2014;515:563-7. [Crossref] [PubMed]
- Brahmer JR, Tykodi SS, Chow LQ, et al. Safety and activity of anti-PD-L1 antibody in patients with advanced cancer. N Engl J Med 2012;366:2455-65. [Crossref] [PubMed]
- Gettinger SN, Horn L, Gandhi L, et al. Overall Survival and Long-Term Safety of Nivolumab (Anti-Programmed Death 1 Antibody, BMS-936558, ONO-4538) in Patients With Previously Treated Advanced Non-Small-Cell Lung Cancer. J Clin Oncol 2015;33:2004-12. [Crossref] [PubMed]
- Soria JC, Marabelle A, Brahmer JR, et al. Immune checkpoint modulation for non-small cell lung cancer. Clin Cancer Res 2015;21:2256-62. [Crossref] [PubMed]
- Tumeh PC, Harview CL, Yearley JH, et al. PD-1 blockade induces responses by inhibiting adaptive immune resistance. Nature 2014;515:568-71. [Crossref] [PubMed]
- Carreno BM, Magrini V, Becker-Hapak M, et al. Cancer immunotherapy. A dendritic cell vaccine increases the breadth and diversity of melanoma neoantigen-specific T cells. Science 2015;348:803-8. [Crossref] [PubMed]
- Garon EB, Rizvi NA, Hui R, et al. Pembrolizumab for the treatment of non-small-cell lung cancer. N Engl J Med 2015;372:2018-28. [Crossref] [PubMed]
- Ajona D, Ortiz-Espinosa S, Moreno H, et al. A Combined PD-1/C5a Blockade Synergistically Protects against Lung Cancer Growth and Metastasis. Cancer Discov 2017; [Epub ahead of print]. [Crossref] [PubMed]
- Corrales L, Ajona D, Rafail S, et al. Anaphylatoxin C5a creates a favorable microenvironment for lung cancer progression. J Immunol 2012;189:4674-83. [Crossref] [PubMed]
- Ajona D, Razquin C, Pastor MD, et al. Elevated levels of the complement activation product C4d in bronchial fluids for the diagnosis of lung cancer. PLoS One 2015;10:e0119878 [Crossref] [PubMed]
- Niehans GA, Cherwitz DL, Staley NA, et al. Human carcinomas variably express the complement inhibitory proteins CD46 (membrane cofactor protein), CD55 (decay-accelerating factor), and CD59 (protectin). Am J Pathol 1996;149:129-42. [PubMed]
- Gmiński J, Mykała-Cieśla J, Machalski M, et al. Immunoglobulins and complement components levels in patients with lung cancer. Rom J Intern Med 1992;30:39-44. [PubMed]
- Nishioka K, Kawamura K, Hirayama T, et al. The complement system in tumor immunity: significance of elevated levels of complement in tumor bearing hosts. Ann N Y Acad Sci 1976;276:303-15. [Crossref] [PubMed]
- Huber-Lang M, Younkin EM, Sarma JV, et al. Generation of C5a by phagocytic cells. Am J Pathol 2002;161:1849-59. [Crossref] [PubMed]
- Huber-Lang M, Sarma JV, Zetoune FS, et al. Generation of C5a in the absence of C3: a new complement activation pathway. Nat Med 2006;12:682-7. [Crossref] [PubMed]
- Amara U, Rittirsch D, Flierl M, et al. Interaction between the coagulation and complement system. Adv Exp Med Biol 2008;632:71-9. [Crossref] [PubMed]
- Gorter A, Meri S. Immune evasion of tumor cells using membrane-bound complement regulatory proteins. Immunol Today 1999;20:576-82. [Crossref] [PubMed]
- Donin N, Jurianz K, Ziporen L, et al. Complement resistance of human carcinoma cells depends on membrane regulatory proteins, protein kinases and sialic acid. Clin Exp Immunol 2003;131:254-63. [Crossref] [PubMed]
- Macor P, Tedesco F. Complement as effector system in cancer immunotherapy. Immunol Lett 2007;111:6-13. [Crossref] [PubMed]
- Gelderman KA, Tomlinson S, Ross GD, et al. Complement function in mAb-mediated cancer immunotherapy. Trends Immunol 2004;25:158-64. [Crossref] [PubMed]
- Markiewski MM, DeAngelis RA, Benencia F, et al. Modulation of the antitumor immune response by complement. Nat Immunol 2008;9:1225-35. [Crossref] [PubMed]
- Srivastava MK, Andersson Å, Zhu L, et al. Myeloid suppressor cells and immune modulation in lung cancer. Immunotherapy 2012;4:291-304. [Crossref] [PubMed]
- Srivastava MK, Zhu L, Harris-White M, et al. Myeloid suppressor cell depletion augments antitumor activity in lung cancer. PLoS One 2012;7:e40677 [Crossref] [PubMed]