Tumor microenvironments of NSCLC are more heterogeneous than you would think—supported by image analysis-based assessment of PD-L1 and tumor-associated immune cell densities
In the recent years, blockade of immune checkpoints to reinstitute host antitumor immunity has been extensively investigated in a variety of tumors (1). In the field of lung cancer, monoclonal antibodies targeting the programmed death 1 (PD-1) (also known as CD279) receptor and its ligand, programmed death-ligand 1 (PD-L1) (also known as B7-H1) have been most studied. High profile clinical trials have shown impressive anti-tumor activity of PD-1/PD-L1 blockade and significant improvements in overall survival (OS) of non-small cell lung cancer (NSCLC) patients in the first-line and/or second or more line settings (2-6), leading to approval of nivolumab, pembrolizumab and/or atezolizumab for treatment of NSCLC in the US, Europe and some other countries (7). Clinical trials with two other agents, durvalumab and avelumab, have also shown promising results (7).
In these clinical trials, correlation between PD-L1 protein expression in tumor cells and/or immune cells by immunohistochemistry (IHC) and clinical responses to anti PD-1/PD-L1 agents has been evaluated. Although the PD-L1 expression generally serves as a marker to predict clinical responses, only up to 45% of patients whose tumors express high levels of PD-L1 respond to the blockade (6). Therefore, other factors in the tumor microenvironment including tumor-associated lymphocytes (TILs) and tumor-associated macrophages (TAMs) may contribute to the response or resistance to the PD-1/PD-L1 blockade. In fact, it has been proposed that four different types of tumor microenvironment exist based on the presence and absence of TILs and PD-L1 expression (8,9). Further, some patients with tumors negative for PD-L1 expression have responded to those agents (2,4,5,10,11). It may be attributed in part to heterogeneity in PD-L1 expression within a tumor and/or between primary and metastatic lesions, changes in the PD-L1 expression level pre and post chemo/radiation therapies and subjectivity in interpretation of PD-L1 IHC by pathologists.
Multiple groups have correlated PD-L1 expression with clinicopathologic features and prognosis in NSCLC leading to conflicting results (Table 1) (12,13). The discrepant results may be due to differences in study cohorts (ethnicities, histologic types and tumor stages), the type of specimens evaluated [whole histologic sections vs. tissue microarrays (TMA)], the method of protein expression analysis (IHC vs. immunofluorescence), quantification assessment (image analysis vs. microscopic observation), scoring system [the intensity and extent (including H scoring) vs. extent of positive tumor cells] and the cut-off for positivity. Among the clinicopathologic features, as mentioned briefly, tumor associated immune cells (TAICs) consisting of TILs and TAMs comprise an important element of tumor microenvironment, and their characteristics and interaction with PD-L1 expressing tumor cells likely dictate response to PD-1/PD-L1 blockade (9). However, only a limited number of studies have attempted to correlate the expression of PD-L1 with TAIC density and characteristics, particularly TILs, using a limited number of IHC markers (e.g., CD8, CD45RO) or simple histologic evaluation (14). Thus, an assessment of a comprehensive panel of immune markers, ideally with an objective method, to characterize the association between PD-L1 expression, immune microenvironment, clinicopathologic features of tumors and patient outcomes is warranted to determine distinct subgroups of NSCLC.
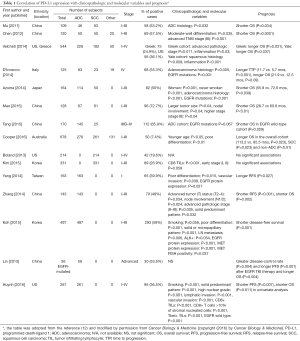
Full table
With this background, Parra and colleagues characterized the expression of a large panel of immune markers (PD-L1, PD-1, and 8 others) by IHC and assessed the TAIC densities in surgically resected NSCLC specimens [stage I–III, 146 adenocarcinomas (ADCs) and 108 squamous cell carcinomas (SCCs)] (14). In this study, the PD-L1 IHC was performed using an automated platform (BOND-MAX, Leica Microsystems) with the antibody clone E1L3N (dilution 1:100; Cell Signaling Technology), the combination that has shown similar performance in detecting PD-L1 protein expression in NSCLC to the FDA approved PD-L1 IHC assays [PD-L1 IHC 28-8 and 22C3 pharmDx assays (Agilent technologies/DAKO)] (15). To assess the expression of PD-L1 and various TAIC markers objectively, the authors applied quantitative image analysis on digitally scanned slides and correlated the findings with the clinicopathologic and molecular features and prognosis. While the results of the study confirmed the previously reported findings (the association of high PD-L1 expression and high TAIC densities with positive smoking history and solid histologic pattern in ADC patients) (12), several important findings have also been discovered.
First, the authors looked at the concordance of PD-L1 expression between the whole section and the corresponding TMA cores in a subset of the cohort to answer the question whether PD-L1 expression on the tumor cells in a biopsy could represent that of the entire tumor. Using H-scores they found that PD-L1 expression on the tumor cells in whole histologic sections correlated positively and significantly with that in TMA cores (average of 3× or 2×1.0-mm cores per case) in both ADCs and SCCs. They also observed a positive and significant correlation between the expression of PD-L1 on tumor cells and TAMs in whole sections and that in five randomly selected 1-mm areas of each section leading the conclusion that randomly selected 3× to 5×1-mm areas could serve as surrogates for whole tumor sections. The results are different from those of two prior reports that showed discordant expression of PD-L1 on tumor cells when compared between the whole tissue section and the corresponding individual TMA cores or biopsy specimen (16,17). The difference could be explained by the methods used, but it appears to be important to obtain a tissue sample from multiple random areas of the tumor to represent the entire tumor tissue, since Ilie and colleagues also found in their study that there was a trend toward larger numbers of tissue fragments in the biopsy associated with concordant results when PD-L1 expression was compared between the whole section and the corresponding biopsy specimen (16).
Second, the study has shown the differences in PD-L1 expression as well as those in the distribution and characteristics of TAICs between ADCs and SCCs. Of those, membranous staining of PD-L1 on tumor cells with a 5% cut-off was present in 23% of ADCs and 31% of SCCs, and larger numbers of SCC specimens were positive for PD-L1 expression with various cut-offs than ADCs. In addition, SCCs exhibited significantly higher PD-L1 H-score on TAMs than ADCs, while PD-L1 expression on TAMs was more frequently observed than that on tumor cells in both histologies. Of note, PD-L1 expression can be induced on TAMs by IFNγ and VEGF similar to that on tumor cells (18,19), leading to effective suppression of T cell immunity, and thus appearing to be biologically relevant and possibly associated with tumor progression. These results suggest that SCCs may have a milieu more responsive to PD-1/PD-L1 axis blockade than ADCs.
In order to show the difference in the distribution and characteristics of TAICs between the two histologies, the following immune markers were stained individually and counted both in the peritumoral and intratumoral components: CD3 (a marker for T cells), CD4 (a marker for helper T cells), CD8 and granzyme B (markers for cytotoxic T cells), CD57 (a marker for natural killer and senescent T cells), CD45RO (a marker for memory T cells), PD-1 and FOXP3 (markers for regulatory T cells) and CD68 (marker for TAMs). Interestingly, in the peritumoral compartment, SCCs exhibited higher densities of TILs expressing all markers except PD-1 than ADCs, while ADCs harbored higher densities of CD3+, CD4+ and CD57+ cells in the intratumoral compartment than SCCs. TAMs were more prominent in the peritumoral than intratumoral compartments in both histologies, but TAMs appeared to be more closely associated with tumor cells within the intratumoral compartment in ADCs than in SCCs. Furthermore, the assessment of both PD-L1 expression on tumor cells and TAIC densities in the whole section specimen found that the majority of TIL markers, but not CD68 (TAM), exhibited a significant positive correlation with the PD-L1 expression in both the intratumoral and peritumoral compartments of ADCs, while the correlation was less significant in SCCs. These findings suggest that the two major histologic types of lung cancer harbor different immune microenvironments.
When the tumor microenvironment of the study cohort was classified into four types as proposed by Taube et al. (Figure 1) (8), however, the similar fractions (1/5 to 1/4) of the tumors harbored the immune microenvironment considered to be responsive to PD-1/PD-L1 blockade in both early stage ADCs and SCCs, although the latter may have the responsive microenvironment slightly more frequently than the former. In this study, membranous staining of any intensity on 5% of the tumor cells was used as the cut-off for positive PD-L1 expression and the mean density of CD3+ TIL as the cut-off for the presence of TILs, and revealed type I (adoptive immune resistance) in 19% of ADCs and 24% of SCCs, type II (immunological ignorance) in 29% of ADCs and 26% in SCCs, type III (intrinsic induction) in 3% of ADCs and 7% of SCCs and type IV (tolerance) in 48% of ADCs and 43% in SCCs.
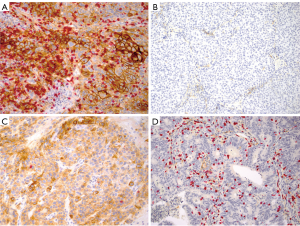
Finally it was shown that the densities of TAICs expressing several immune markers were associated with NSCLC patient outcomes, while PD-L1 expression on tumor cells was not significantly associated with the outcomes as much in multivariate analysis. In univariate analysis, however, PD-L1 H-scores on tumor cells higher than the median and greater than 5 showed non-significant associations with shorter OS in ADCs. Interestingly, SCCs showed significant correlation of higher PD-L1 expression on TAMs (> the median H-score) with shorter OS and non-significant correlation with recurrent free survival (RFS), suggesting that PD-L1+ TAMs may contribute to tumor progression as well as response to PD-1/PD-L1 blockade (20).
Importantly, the role of TAIC densities predicting patient outcomes appears to be different between ADCs and SCCs, and between intratumoral and peritumoral compartments. In SCCs, abundant CD57+ cells in the peritumoral compartment were significantly associated with longer RFS and OS, while higher CD68+ TAM densities in the intratumoral compartment showed a non-significant association with shorter RFS. Conversely, higher intratumoral CD68+ TMA densities were associated with longer RFS in ADCs. Similarly, higher intratumoral CD4+ and FOXP3+ cell densities were significantly associated with longer RFS and OS, but only in univariate analysis. Furthermore, a combined analysis of PD-L1 expression on tumor cells (H score >5) and CD4/CD8/CD68+ TAIC densities (< the median density) identified a small subset of ADC patients with shorter RFS and OS. Thus, it is important not only to evaluate TAIC markers and PD-L1 expression individually, but also to conduct combined analyses to predict patient outcomes.
While the study has demonstrated the differences in distribution and characteristics of TAICs, their association with PD-L1 expressing cells and their prognostic significance between ADCs and SCCs through comprehensive and objective analyses of PD-L1 and various TAIC marker expressions, there are a few shortcomings. In particular, a single IHC assay per section was evaluated in the study, thus co-expression of multiple TIAC markers in a single immune cell and interaction of PD-L1 positive tumor cells and/or TAMs with TAICs expressing different markers could not be assessed. For instance, the authors encountered a couple of unexpected findings: (I) the number of CD4+ and CD8+ cells combined was slightly larger than the number of CD3+ cells in both tumor types and compartments; (II) the number of cells expressing granzyme B was significantly lower than the cells expressing CD8. They only hypothesized that the presence of CD4+ non-T cells (monocytes/macrophages, eosinophils, progenitor cells and NK cells) and double CD4/CD8+ T cells (21) were attributed to the former, while the decreased proportion of granzyme B expressing CD8+ T cells in lung tumors was likely a result of yet-to-be determined soluble mediators secreted by tumor cells (22). In this context, however, multiplex IHC or immunofluorescence assays may be able to demonstrate the presence of CD4+/CD8+, CD4+/CD68+ and CD4+/CD57+ dual positive cells, and the expression of other markers in CD8+/granzyme B- T cells to gain insights. The multiplex assays could also reveal the spatial interaction between PD-L1+ tumor cells and/or TAMs and various types of TAICs facilitating the prediction of response to PD-1/PD-L1 blockade (23), and underscoring the biological significance of multiple results that were found in the study.
Another issue is the lack of treatment response data. The study cohort consisted of stage I–III NSCLCs to utilize resection specimens for multiple IHC assays. However, the similar assessment on tissue samples from advanced NSCLC patients who have been treated with anti PD-1/PD-L1 agents, preferably using a multiplex assay, would further advance our understanding of distinct tumor microenvironments in association with response to PD-1/PD-L1 blockade (24).
Acknowledgments
The author thanks Mr. Cris Kenudson for editorial support.
Funding: This work was supported by a Stand Up To Cancer-American Cancer Society Dream Team Translation Research Grant.
Footnote
Provenance and Peer Review: This article was commissioned and reviewed by the Section Editor Yi-Jiu Ren (Department of Thoracic Surgery, Shanghai Pulmonary Hospital, Tongji University School of Medicine, Shanghai, China).
Conflicts of Interest: The author has completed the ICMJE uniform disclosure form (available at http://dx.doi.org/10.21037/tcr.2017.08.10). Mari Mino-Kenudson has served as a consultant for Merrimack Pharmaceuticals, H3 Biomedicine, and Advanced Cell Diagnostics and as an advisory board member for Roche.
Ethical Statement: The authors are accountable for all aspects of the work in ensuring that questions related to the accuracy or integrity of any part of the work are appropriately investigated and resolved.
Open Access Statement: This is an Open Access article distributed in accordance with the Creative Commons Attribution-NonCommercial-NoDerivs 4.0 International License (CC BY-NC-ND 4.0), which permits the non-commercial replication and distribution of the article with the strict proviso that no changes or edits are made and the original work is properly cited (including links to both the formal publication through the relevant DOI and the license). See: https://creativecommons.org/licenses/by-nc-nd/4.0/.
References
- Pardoll DM. The blockade of immune checkpoints in cancer immunotherapy. Nat Rev Cancer 2012;12:252-64. [Crossref] [PubMed]
- Borghaei H, Paz-Ares L, Horn L, et al. Nivolumab versus Docetaxel in Advanced Nonsquamous Non-Small-Cell Lung Cancer. N Engl J Med 2015;373:1627-39. [Crossref] [PubMed]
- Brahmer J, Reckamp KL, Baas P, et al. Nivolumab versus Docetaxel in Advanced Squamous-Cell Non-Small-Cell Lung Cancer. N Engl J Med 2015;373:123-35. [Crossref] [PubMed]
- Herbst RS, Baas P, Kim DW, et al. Pembrolizumab versus docetaxel for previously treated, PD-L1-positive, advanced non-small-cell lung cancer (KEYNOTE-010): a randomised controlled trial. Lancet 2016;387:1540-50. [Crossref] [PubMed]
- Fehrenbacher L, Spira A, Ballinger M, et al. Atezolizumab versus docetaxel for patients with previously treated non-small-cell lung cancer (POPLAR): a multicentre, open-label, phase 2 randomised controlled trial. Lancet 2016;387:1837-46. [Crossref] [PubMed]
- Reck M, Rodriguez-Abreu D, Robinson AG, et al. Pembrolizumab versus Chemotherapy for PD-L1-Positive Non-Small-Cell Lung Cancer. N Engl J Med 2016;375:1823-33. [Crossref] [PubMed]
- Tsao MS, Hirsch FR, Yatabe Y. IASLC atlas of ALK and ROS1 testing in lung cancer. 2nd ed. Colorado: Editorial Rx Press, 2016.
- Taube JM, Anders RA, Young GD, et al. Colocalization of inflammatory response with B7-h1 expression in human melanocytic lesions supports an adaptive resistance mechanism of immune escape. Sci Transl Med 2012;4:127ra37 [Crossref] [PubMed]
- Teng MW, Ngiow SF, Ribas A, et al. Classifying Cancers Based on T-cell Infiltration and PD-L1. Cancer Res 2015;75:2139-45. [Crossref] [PubMed]
- Garon EB, Rizvi NA, Hui R, et al. Pembrolizumab for the treatment of non-small-cell lung cancer. N Engl J Med 2015;372:2018-28. [Crossref] [PubMed]
- Topalian SL, Hodi FS, Brahmer JR, et al. Safety, activity, and immune correlates of anti-PD-1 antibody in cancer. N Engl J Med 2012;366:2443-54. [Crossref] [PubMed]
- Mino-Kenudson M. Programmed cell death ligand-1 (PD-L1) expression by immunohistochemistry: could it be predictive and/or prognostic in non-small cell lung cancer? Cancer Biol Med 2016;13:157-70. [Crossref] [PubMed]
- Huynh TG, Morales-Oyarvide V, Campo MJ, et al. Programmed Cell Death Ligand 1 Expression in Resected Lung Adenocarcinomas: Association with Immune Microenvironment. J Thorac Oncol 2016;11:1869-78. [Crossref] [PubMed]
- Parra ER, Behrens C, Rodriguez-Canales J, et al. Image Analysis-based Assessment of PD-L1 and Tumor-Associated Immune Cells Density Supports Distinct Intratumoral Microenvironment Groups in Non-small Cell Lung Carcinoma Patients. Clin Cancer Res 2016;22:6278-89. [Crossref] [PubMed]
- Rimm DL, Han G, Taube JM, et al. A Prospective, Multi-institutional, Pathologist-Based Assessment of 4 Immunohistochemistry Assays for PD-L1 Expression in Non-Small Cell Lung Cancer. JAMA Oncol 2017; [Epub ahead of print]. [Crossref] [PubMed]
- Ilie M, Long-Mira E, Bence C, et al. Comparative study of the PD-L1 status between surgically resected specimens and matched biopsies of NSCLC patients reveal major discordances: a potential issue for anti-PD-L1 therapeutic strategies. Ann Oncol 2016;27:147-53. [Crossref] [PubMed]
- McLaughlin J, Han G, Schalper KA, et al. Quantitative Assessment of the Heterogeneity of PD-L1 Expression in Non-Small-Cell Lung Cancer. JAMA Oncol 2016;2:46-54. [Crossref] [PubMed]
- Lee SJ, Jang BC, Lee SW, et al. Interferon regulatory factor-1 is prerequisite to the constitutive expression and IFN-gamma-induced upregulation of B7-H1 (CD274). FEBS Lett 2006;580:755-62. [Crossref] [PubMed]
- Loke P, Allison JP. PD-L1 and PD-L2 are differentially regulated by Th1 and Th2 cells. Proc Natl Acad Sci U S A 2003;100:5336-41. [Crossref] [PubMed]
- Kuang DM, Zhao Q, Peng C, et al. Activated monocytes in peritumoral stroma of hepatocellular carcinoma foster immune privilege and disease progression through PD-L1. J Exp Med 2009;206:1327-37. [Crossref] [PubMed]
- Parel Y, Chizzolini C. CD4+ CD8+ double positive (DP) T cells in health and disease. Autoimmun Rev 2004;3:215-20. [Crossref] [PubMed]
- Hodge G, Barnawi J, Jurisevic C, et al. Lung cancer is associated with decreased expression of perforin, granzyme B and interferon (IFN)-gamma by infiltrating lung tissue T cells, natural killer (NK) T-like and NK cells. Clin Exp Immunol 2014;178:79-85. [Crossref] [PubMed]
- Gibney GT, Weiner LM, Atkins MB. Predictive biomarkers for checkpoint inhibitor-based immunotherapy. Lancet Oncol 2016;17:e542-51. [Crossref] [PubMed]
- Gettinger S, Choi J, Mani NL, et al. Predictive Value of Measuring Somatic Mutations and Tumor Infiltrating Lymphocytes for PD-1 Axis Therapy in Non-Small Cell Lung Cancer (NSCLC). J Thorac Oncol 2017;12:S430-1. [Crossref]