SU6668 inhibits the proliferation and motility of colorectal cancer cells by inducing cycle arrest, and promotes their apoptosis
Introduction
Colorectal cancer remains one of the most common cancers worldwide, and its high incidence and mortality rates are of great concern (1,2) While individuals aged <50 years have a low incidence of colorectal cancer, this incidence greatly increases with age. The mean age at which colorectal cancer is diagnosed in developed countries is ~70 years (3). Individuals residing in Europe, North America, and Oceania have the highest incidence of colorectal cancer, while individuals residing in south and central Asia and Africa have the lowest incidence (4). Currently, several countries in Eastern Europe and East Asia, which were previously known as low-risk countries, are now reporting rapidly increasing rates of colorectal cancer. Some possible reasons for these increases may be changes in dietary patterns and risk factors, as people adopt a more “Western lifestyle” (5).
Although encouraging progress has been made in diagnosing and treating colorectal cancer during the past decade, the 5-year overall survival rate among patients with advanced disease is <10%, and treatment with chemotherapy treatment remains essential for these patients. Targeted therapies against the epidermal growth factor receptor and vascular endothelial growth factor, as well as chemotherapy regimens consisting of 5-fluorouracil, oxaliplatin (OXA), irinotecan, cetuximab, and bevacizumab are appropriate first-line treatments for colorectal cancer, and alternate treatment regimens are available upon disease progression (6). However, the majority of patients receiving aggression treatments will eventually relapse and die as a result of their disease, and thus more effective therapeutic strategies are needed.
One strategy for treating colorectal cancer involves interfering with the tumor’s ability to form new blood vessels, which occurs during a process known as angiogenesis. This process plays pivotal roles in tumor growth, invasion, and metastasis.
In preclinical testing, oral administration of SU6668 produced a significant antitumor effect on the diverse panel of human tumor xenografts of glioma, melanoma, lung, colon, ovarian, and epidermoid origin (7,8). Previous studies reported that SU6668 inhibited the liver metastasis of both human and mouse colon cancer xenografts by exerting an antiangiogenic effect (7), which was believed to constitute its mechanism of action. However, the effects of SU6668 on colorectal cancer cell proliferation, migration, colony formation, invasion, and apoptosis have remained unclear.
In the study, we found that SU6668 inhibited the proliferation and colony formation of colorectal cancer cells in a dose- and time-dependent manner. SU6668 exposure suppressed the migration and invasion abilities of colorectal cancer cells and induced their G0/G1 cell cycle arrest and apoptosis.
It is well known that Bcl-2-associated X protein (Bax) and B cell lymphoma/leukemia-2 protein (Bcl-2) play central roles in regulating apoptosis (9,10). Apoptosis is one of the most important processes that controls the numbers of cells in tissues, and is regulated by the opposing effects of Bcl-2 and Bax proteins (11). Decreased Bcl-2 protein expression coupled with increased Bax protein expression promotes cell apoptosis and inhibits cell proliferation (12). Caspase-3 is another key factor involved in apoptosis, and is regarded as the ultimate enforcer of the cell apoptosis process (13,14). In the study, the activated apoptotic genes (Bax and caspase-3) were observed in SU6668-treated cells, and compared with the same genes in control cells. Simultaneously, we found that the levels of an important anti-apoptotic protein (Bcl-2) were significantly decreased in cells treated with SU6668.
Methods
Cell culture and treatment
Human colorectal adenocarcinoma cell lines SW480 and SW620 were obtained from American Type Culture Collection (Manassas, VA, USA), and then cultured in DMEM (Invitrogen, Life Technologies, Carlsbad, CA, USA) medium supplemented with 10% fetal bovine serum (FBS) (Gibco, Carlsbad, CA, USA). The cells were maintained at 37 °C in a water-saturated atmosphere containing 5% CO2.
Cell proliferation assays
CCK-8 and EdU staining methods were used to detect cell proliferation. For the CCK-8 assays, aliquots of cells were seeded into 96-well microtiter plates (3,000 cells per well) and treated with vehicle or different concentrations of SU6668 (Selleckchem, Houston, TX, USA) for different time periods. After 24 h, the cell medium was removed from each well and replaced with fresh medium. Next, 10 µL of CCK-8 solution (Dojin Laboratories, Kumamoto, Japan) was added to each well and incubated. The absorbance of each well at 450 nm was then measured using a SpectraMax M5 microplate reader (Molecular Devices, Sunnyvale, CA, USA). For the EdU assays, cells were seeded into 6-well plates and treated with 50 µM 5-ethynyl-2'-deoxyuridine (Edu; RiboBio, Guangzhou, China) for 2 h; after which, the cells were collected and stained with reagents in an Apollo®488 EdU labeling kit (RiboBio, C10338) according to the manufacturer’s instructions. Following staining, the cells were analyzed with a FACScan flow cytometer (Becton Dickinson, Mountain View, CA, USA) equipped with Flow Plus software.
Colony formation assay
An aliquot containing 1,000 cells was seeded into each well of a 6-well plate (Corning, NY, USA). The cells were maintained in a medium containing 10% FBS, and the medium was refreshed every 2 days. The plates were incubated for 2 weeks at 37 °C in a 5% CO2 incubator, and the number of cell colonies (defined as a cluster containing >50 cells) was manually counted after staining with 0.1% crystal violet (Sigma Aldrich, St. Louis, MO, USA) solution. Each experiment was repeated 3 times.
Cell cycle assay
SW480 and SW620 cells were exposed to SU6668 for 48 h; after which, they were collected and resuspended with pre-cooled ethanol solution (70%). Afterwards, cells were stained with cell cycle staining kit (LiankeBio, Zhejiang, China) according to the manufacturer’s guideline. Then cells were immediately counted with a FACScan flow cytometer (Becton Dickinson, Mountain View, CA, USA), and the data were analyzed by Flow Plus software.
Apoptosis assay
Cells were seeded into 6-well plates and treated with 30 µM SU6668 for 48 h; after which, they were collected and double stained with Annexin V-FITC/PI (LiankeBio, Zhejiang, China) according to the manufacturer’s guideline. After staining, the cells were immediately counted with a FACScan flow cytometer (Becton Dickinson, Mountain View, CA, USA), and the data were analyzed by Flow Plus software.
Hoechst staining
Hoechst staining was performed to detect apoptotic cell death. SW480 and SW620 cells were treated with 30 µM SU6668 for 48 h; after which, they were collected and washed twice with cold PBS, and then stained in the dark with Hoechst staining buffer (Thermo Fisher Scientific, Waltham, MA, USA) for 15 min. After staining, the cells were fixed in 4% paraformaldehyde, washed, and then photographed with a fluorescence confocal microscope (Olympus FV1000; Olympus Corp., Tokyo, Japan). Each experiment was performed in triplicate.
Western blot analysis
The cells were treated with 30 µM SU6668 for 48 h, and then collected to extract their whole cell proteins with RIPA buffer (Applygen Technologies Inc., Beijing, China). The bicinchoninic acid (BCA) protein assay (Pierce Protein Biology, Waltham, MA, USA) was used to determine total protein concentrations. Aliquots of protein were separated by 10% SDS-PAGE, and the protein bands transferred onto a PVDF membrane, which was then washed in TBS-T and blocked with 5% BSA. The membrane was then incubated with primary antibodies against Bax (1:1,000), caspase-3 (1:1,000), Bcl-2 (1:1,000), and GAPDH (1:1,500) (Cell Signaling Technology, Danvers, MA, USA), vimentin (1:1,000), E-cadherin (1:1,000) (ABcam, Cambridge, UK). Next, the membrane was incubated with HRP-conjugated goat anti-rabbit secondary antibodies (1:1,000; ProMab, Richmond, CA, USA), and the stained protein bands were visualized using the enhanced chemiluminescence technique (ECL, Amersham Biosciences, Little Chalfont, UK).
Cell migration assay
Briefly, the cells were first pretreated with 30 µM SU6668 for 18 h, and then suspended in a serum-free medium for 12 h at density of 50,000 cells/mL after washed twice with PBS. An aliquot of the suspended cells was then seeded into the upper well of a Boyden chamber (6.5 mm in diameter, 8 µm pore size), and incubated for 18 h (with no serum). FBS (10%) was added to the lower well. The cells on the membrane were fixed with 3.7% formaldehyde for 20 min, and then stained with 0.1% crystal violet for 20 min. The number of migrating cells was counted after removing the upper chamber cells with a cotton swab.
Matrigel invasion assay
Cell invasion through the Matrigel membrane was quantitated using Matrigel-coated Transwell chambers (BD Biosciences, San Jose, CA, USA) according to the manufacturer’s instructions. Cells pretreated with 30 µM SU6668 were seeded into the upper Matrigel-coated chambers after cultured with serum-free medium for 12 h. Subsequently, cells were plated into upper chamber (with no serum). DMEM containing 10% FBS was added to the lower chambers. After 18 h, non-invading cells in the upper chamber were removed by scrubbing with a cotton-tipped swab. The invading cells in the lower chambers were fixed with 4% paraformaldehyde for 20 minutes, and then stained with 0.2% crystal violet for 10 minutes. Six fields for each chamber were photographed using an inverted microscope equipped with a camera, and the number of invading cells in each visual field was counted.
Real-time PCR
The effects of SU6668 on Bax, Bcl-2, vimentin, and E-cadherin gene expression were investigated via real-time PCR analyses. Briefly, total cellular RNA was extracted, and reverse transcription was performed using a Bestar qPCR RT kit (DBI Bioscience, China). To quantify gene amplification, real-time PCR was performed using an Agilent StrataGene Mx3005P Sequence Detection System and SybrGreen Master Mix (DBI Bioscience). The cycling parameters were 94 °C for 2 min, followed by 40 cycles of 94 °C for 20 s, 58 °C for 20 s, and 72 °C for 20 s. A melting curve analysis was subsequently performed. The relative expression levels of the target genes were normalized to the fold-levels in the corresponding control cells. The primer sequences used are shown in Table 1.
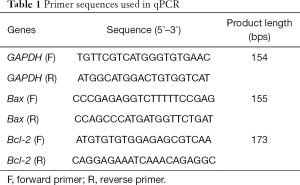
Full table
Statistical analysis
All results are presented as the mean ± SEM of at least three independent experiments. Student’s t-test was used to assess differences between two groups and one-way analysis of variance was used for multiple comparisons. All statistical analyses were performed using GraphPad Prism Software Version 3.0 (San Diego, CA, USA). A value of P<0.05 was considered statistically significant.
Results
SU6668 inhibited colorectal cancer cell proliferation and colony formation
To understand how SU6668 affects the proliferation of colorectal cancer cells, SW480 and SW620 cells were treated with different concentrations of SU6668 (15 µM, 30 µM, and 45 µM, respectively), and then tested for their proliferation. As shown in Figure 1A, SU6668 inhibited the proliferation of colorectal cancer cells in a dose-dependent manner. Because this inhibition was statistically significant at a 30-µM concentration, we chose that concentration of SU6668 for use in our subsequent experiment. Furthermore, our EdU staining results also confirmed that a 30-µM SU6668 concentration effectively inhibited colorectal cancer cell proliferation (Figure 1B,C). We then treated the SW480 and SW620 cells with 30 µM SU6668 for different time periods, and found that their proliferation was significantly suppressed after 48 h of exposure to SU6668 (Figure 1D,E). In addition, we also assessed the effect of SU6668 on the colony formation ability of colorectal cancer cells. The single colony observed in the SU6668 treatment groups was significantly less than the numbers of colonies observed in the control groups, and showed that SU6668 had significantly reduced the ability of colorectal cancer cells to colonize (Figure 1F,G).
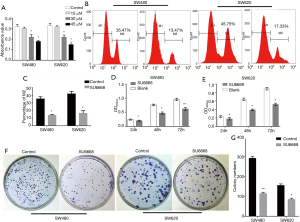
SU6668 induced G0/G1 cell cycle arrest in colorectal cancer cells
As cell proliferation is closely related to the cell cycle, the ability to regulate cell cycle progression and induce G1-phase cell cycle arrest have become major positive indicators for drugs being developed to treat malignant tumors. As SU6668 significantly inhibited the proliferation of colorectal cancer cells, we next examined whether SU6668 might influence the cell cycle distribution. Our cell cycle assay results showed that SU6668 treatment (30 µM) increased the percentage of G1 phase cells by 21.95% (SW480 cells) and 16.56% (SW620 cells), respectively (Figure 2A), while the numbers of cells in S phase decreased by 19.90% and 14.43%, respectively (Figure 2B). These results indicated that SU6668 inhibits colorectal cancer cell proliferation by inducing G1 cell cycle arrest.
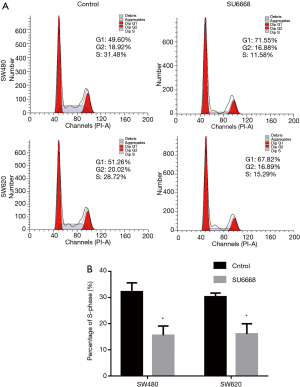
SU6668 induced apoptosis in colorectal cancer cells by activating Bax and caspase 3
As shown in Figure 3A,B, the rates of apoptosis among SW480 and SW620 cells treated with SU6668 were increased by 30.4% and 29.3%, respectively. Hoechst staining was performed to further confirm the apoptotic effect of SU6668 on colorectal cancer cells. The condensed chromatin stained by Hoechst dye in apoptotic cells showed more brightly than in normal cells. Moreover, SW480 and SW620 cells treated with SU6668 and then examined after Hoechst staining displayed more extensive apoptosis-related morphologic changes when compared with normal cells (Figure 3C). We also assessed the levels of apoptosis-associated proteins in SU6668 treated cells, and found that the mRNA levels of Bcl-2 were significantly decreased in SU6668 treated cells, and Bax exhibited a contrary tendency (Figure 3D). As Bax and Bcl-2 are major members of the Bcl-2 protein family, which plays critical roles in the mitochondrial apoptotic pathway, we investigated the cellular mitochondria to detect biochemical evidence of apoptosis. Our results revealed that Bax expression in the mitochondria was clearly increased following SU6668 treatment, while Bcl-2 expression was decreased (Figure 3E).
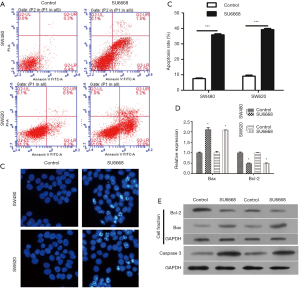
SU6668 suppressed the migration and invasion of colorectal cancer cells
To further assess the effects of SU6668 on the migration and invasion of colorectal cancer cells, we performed the cell migration and Matrigel invasion assays using cells treated with SU6668. As shown in Figure 4A,B, SU6668 attenuated the migration and invasion capabilities of SW480 and SW620 cells (Figure 4C,D). Additionally, we also detected the levels of vimentin RNA and protein (a metastasis marker) and E-cadherin RNA and protein (a metastasis suppressor) to confirm the effects of SU6668. Our results showing the inhibition of vimentin and increased E-cad activity indicated that SU6668 could suppress the migration and invasion of colorectal cancer cells (Figure 4E).
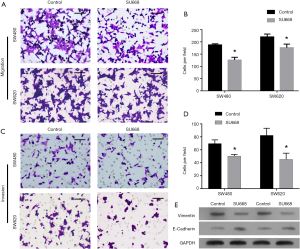
Discussion
Colorectal cancer results from gene mutations and epigenetic alterations in colon epithelial cells. These mutations and epigenetic alterations are believed to drive the development of malignant adeno-carcinomas by regulating cellular processes related to proliferation and apoptosis. Hence, we chose SU6668 as our selective inhibitor of colorectal cancer cell proliferation because its oral administration produced a significant antitumor effect on a diverse panel of human tumor xenografts of glioma, melanoma, lung, colon, ovarian, and epidermoid origin during preclinical testing. The ability of SU6668 to inhibit tumor angiogenesis and pericyte vessel coverage, and promote apoptosis of both tumor cells and endothelial cells in a colon cancer liver metastases mouse model had been proven in a previous study (1). Recent research indicates that SU6668 could inhibit prostate cancer malignant behaviors through MTDH/AKT signaling pathway (8). Consistent with our in vitro results, we found that SU6668 could inhibit the proliferation of colorectal cancer cells in a dose- and time-dependent manner, and also induce their apoptosis. Moreover, similar results were found for breast cancer cells (15).
The invasion of cancer cells is the prelude for their metastasis (16). Therefore, the targeting of invading cancer cells is critical for preventing metastasis (17). In our study, we found that SU6668 suppressed the migration and invasion of colorectal cancer cells. Interestingly, previous studies showed that SU6668 inhibited the epithelial-mesenchymal transformation (EMT) process, as based on decreased expression of E-cadherin in MCF-7 cells (15,18). The EMT process is known to be closely associated with tumor metastasis. We found that SU6668 could inhibit the EMT process, and thus inhibit the migration and invasion of colorectal cancer cells. However, the exact mechanism for this effect requires further investigation.
To effectively target invading cancer cells, it is necessary to determine their cell cycle phase, because uncontrolled cell growth, which is tightly regulated by the cell cycle, is a potential trigger for tumorigenesis or the aggressive behavior of cancer cells (19). Furthermore, it is known that in many cancers, including colorectal cancer, cellular proliferative activities frequently become increased or deregulated due to changes in cell cycle modulators (20). Although the mechanisms underlying the disordered cell cycles found in malignant tumors remain elusive, studies have shown that if the cells are arrested at a given cell-cycle phase, this arrest results in the cancer cells becoming non-proliferative. Studies have also demonstrated that a cell division cycle initiated during the G1 phase of the cell cycle leads to proliferation (21). Therefore, arresting the cell cycle at the G1 phase has become a major objective when seeking to treat malignant tumors. Hence, in this study, we explored the effect of SU6668 on the cell cycle arrest of colorectal cancer cells. Apoptosis is a biological process that is regulated by interactions between different Bcl-2 protein family members. The Bcl-2 family consists of both pro- and anti-apoptotic members, and plays critical roles in mitochondrial apoptotic pathways (22). Bax and Bcl-2 are the major members of the Bcl-2 protein family, and play central roles in determining the progression and prognosis of different human malignancies [30]. Bax protein is crucial for activation of both the intrinsic and extrinsic apoptotic pathways (23,24), and high levels of Bax expression have been correlated with longer survival times among patients with hepatic metastasis of colorectal cancer (25). In contrast, Bcl-2, a new class of proto-oncogene, suppresses cell death without affecting proliferation, and prevents apoptosis by inhibiting Bax activity (26,27). In our study, flow cytometry analyses revealed significantly increased rates of apoptosis among cells treated with SU6668. Furthermore, Hoechst staining studies revealed a higher incidence of apoptotic morphological changes in SU6668-treated cells, including chromatin condensation, apoptotic bodies, and shrunken cell nuclei. Finally, the expression of apoptosis-related proteins Bax and caspase-3 was activated by SU6668 exposure, while Bcl-2 protein expression was significantly decreased by SU6668 exposure.
Conclusions
Our study investigated the effects of SU6668 on colorectal cancer cell proliferation, migration, colony formation, invasion, and apoptosis for the purpose of developing new strategies for treating colorectal cancer. We found that SU6668 inhibited the proliferation, migration, colony formation, and invasion of colorectal cancer cells. SU6668 inhibited the proliferation of SW480 and SW620 colorectal cancer cells by inducing G1 cell cycle arrest, and induced apoptosis by regulating their apoptosis-related proteins. The apoptosis-related proteins Bax and caspase-3 found in colorectal cancer cells were activated by exposure to SU6668.
Acknowledgments
Funding: This research was supported by grants from the Natural Sciences Foundation of Shandong (ZR2013HL043).
Footnote
Conflicts of Interest: All authors have completed the ICMJE uniform disclosure form (available at http://dx.doi.org/10.21037/tcr.2017.08.38). The authors have no conflicts of interest to declare.
Ethical Statement: The authors are accountable for all aspects of the work in ensuring that questions related to the accuracy or integrity of any part of the work are appropriately investigated and resolved. The study was conducted in accordance with the Declaration of Helsinki (as revised in 2013). Institutional ethical approval and informed consent were waived.
Open Access Statement: This is an Open Access article distributed in accordance with the Creative Commons Attribution-NonCommercial-NoDerivs 4.0 International License (CC BY-NC-ND 4.0), which permits the non-commercial replication and distribution of the article with the strict proviso that no changes or edits are made and the original work is properly cited (including links to both the formal publication through the relevant DOI and the license). See: https://creativecommons.org/licenses/by-nc-nd/4.0/.
References
- Pink RC, Samuel P, Massa D, et al. The passenger strand, miR-21-3p, plays a role in mediating cisplatin resistance in ovarian cancer cells. Gynecol Oncol 2015;137:143-51. [Crossref] [PubMed]
- Wei X, Wang W, Wang L, et al. MicroRNA-21 induces 5-fluorouracil resistance in human pancreatic cancer cells by regulating PTEN and PDCD4. Cancer Med 2016;5:693-702. [Crossref] [PubMed]
- Siegel R, DeSantis C, Virgo K, et al. Cancer treatment and survivorship statistics, 2012. CA Cancer J Clin 2012;62:220-41. [Crossref] [PubMed]
- Center MM, Jemal A, Smith RA, et al. Worldwide variations in colorectal cancer. CA Cancer J Clin 2009;59:366-78. [Crossref] [PubMed]
- Brenner H, Kloor M, Pox CP. Colorectal cancer. Lancet 2014;383:1490-502. [Crossref] [PubMed]
- Au Yeung CL, Co NN, Tsuruga T, et al. Exosomal transfer of stroma-derived miR21 confers paclitaxel resistance in ovarian cancer cells through targeting APAF1. Nat Commun 2016;7:11150. [Crossref] [PubMed]
- Laird AD, Vajkoczy P, Shawver LK, et al. SU6668 is a potent antiangiogenic and antitumor agent that induces regression of established tumors. Cancer Res 2000;60:4152-60. [PubMed]
- Qian B, Yao Y, Liu C, et al. SU6668 modulates prostate cancer progression by downregulating MTDH/AKT signaling pathway. Int J Oncol 2017;50:1601-11. [Crossref] [PubMed]
- Czabotar PE, Lessene G, Strasser A, et al. Control of apoptosis by the BCL-2 protein family: implications for physiology and therapy. Nat Rev Mol Cell Biol 2014;15:49-63. [Crossref] [PubMed]
- Kvansakul M, Hinds MG. The Bcl-2 family: structures, interactions and targets for drug discovery. Apoptosis 2015;20:136-50. [Crossref] [PubMed]
- Lee MS, Ha JH, Yoon HS, et al. Structural basis for the conserved binding mechanism of MDM2-inhibiting peptides and anti-apoptotic Bcl-2 family proteins. Biochem Biophys Res Commun 2014;445:120-5. [Crossref] [PubMed]
- Petros AM, Olejniczak ET, Fesik SW. Structural biology of the Bcl-2 family of proteins. Biochim Biophys Acta 2004;1644:83-94. [Crossref] [PubMed]
- Juraver-Geslin HA, Durand BC. Early development of the neural plate: new roles for apoptosis and for one of its main effectors caspase-3. Genesis 2015;53:203-24. [Crossref] [PubMed]
- Porter AG, Jänicke RU. Emerging roles of caspase-3 in apoptosis. Cell Death Differ 1999;6:99-104. [Crossref] [PubMed]
- Wang L, Liu Z, Ma D, et al. SU6668 suppresses proliferation of triple negative breast cancer cells through down-regulating MTDH expression. Cancer Cell Int 2013;13:88. [Crossref] [PubMed]
- Sahai E. Illuminating the metastatic process. Nat Rev Cancer 2007;7:737-49. [Crossref] [PubMed]
- Goss PE, Chambers AF. Does tumour dormancy offer a therapeutic target? Nat Rev Cancer 2010;10:871-7. [Crossref] [PubMed]
- Smith BN, Bhowmick NA. Role of EMT in Metastasis and Therapy Resistance. J Clin Med 2016;5:E17 [Crossref] [PubMed]
- Hanahan D, Weinberg RA. The hallmarks of cancer. Cell 2000;100:57-70. [Crossref] [PubMed]
- Fernández PL, Jares P, Rey MJ, et al. Cell cycle regulators and their abnormalities in breast cancer. Mol Pathol 1998;51:305-9. [Crossref] [PubMed]
- Gosepath EM, Eckstein N, Hamacher A, et al. Acquired cisplatin resistance in the head-neck cancer cell line Cal27 is associated with decreased DKK1 expression and can partially be reversed by overexpression of DKK1. Int J Cancer 2008;123:2013-9. [Crossref] [PubMed]
- Cory S, Huang DC, Adams JM. The Bcl-2 family: roles in cell survival and oncogenesis. Oncogene 2003;22:8590-607. [Crossref] [PubMed]
- Jia L, Macey MG, Yin Y, et al. Subcellular distribution and redistribution of Bcl-2 family proteins in human leukemia cells undergoing apoptosis. Blood 1999;93:2353-9. [PubMed]
- Jia L, Patwari Y, Srinivasula SM, et al. Bax translocation is crucial for the sensitivity of leukaemic cells to etoposide-induced apoptosis. Oncogene 2001;20:4817-26. [Crossref] [PubMed]
- Katkoori VR, Suarez-Cuervo C, Shanmugam C, et al. Bax expression is a candidate prognostic and predictive marker of colorectal cancer. J Gastrointest Oncol 2010;1:76-89. [PubMed]
- Hector S, Prehn JH. Apoptosis signaling proteins as prognostic biomarkers in colorectal cancer: a review. Biochim Biophys Acta 2009;1795:117-29.
- Mohan S, Abdelwahab SI, Kamalidehghan B, et al. Involvement of NF-κB and Bcl2/Bax signaling pathways in the apoptosis of MCF7 cells induced by a xanthone compound Pyranocycloartobiloxanthone A. Phytomedicine 2012;19:1007-15. [Crossref] [PubMed]