Intensity-modulated radiotherapy, volume-modulated arc therapy and helical tomotherapy for locally advanced nasopharyngeal carcinoma: a dosimetric comparison
Introduction
Nasopharyngeal carcinoma (NPC) has been one of the most common cancers in southern China, particularly in the provinces of Guangdong, Guangxi and Hunan. For a long time, radiation therapy (RT) has remained a primary treatment modality for non-metastatic NPC due to its structural proximity to tumor with high radiation sensitivity. Over the last decade, many encouraging advances have been made in improving therapeutic index of RT for NPC. Of note, the development of intensity-modulated radiation therapy (IMRT) has greatly improved the quality-of-life for NPC patients (1-3). However, IMRT has some inherent drawbacks. The plan of a step-and-shoot IMRT (SaS-IMRT) delivered by a linear accelerator is generally so complex that approximately 7 to 9 beams are usually required for achieving a decent dose distribution within target. It greatly increased delivery time and monitor units (MUs). Firstly, prolonged delivery time decreased efficiency and increased intrafraction setup errors during treatment (4,5). Secondly, high number of MUs boosted scattered radiation and integral radiation dose so as to elevate the risks of secondary tumors in patients (6).
In 2007, volumetric-modulated arc therapy (VMAT) was first introduced to overcome the above drawbacks of IMRT. VMAT can generate precise conformal dose distribution by variability of multi-leaf collimator (MLC) position, dose rate and gantry rotation speed with continuous modulation during a single 360°rotation. Compared with IMRT, VMAT could improve dose distribution, reduce dose to organs at risk (OARs) and shorten delivery time (7).
A newly developed technique, Hi-Art helical tomotherapy (HT), combines inverse planning of intensity modulation and a full 360° radiation beam direction (8). Capable of calculating the MLC position every 7 degree of rotation, HT offers a better dosage conformity as compared with IMRT and results in higher tumor control probability (TCP) and lower normal tissue complication probability (NTCP) (9). Consolidating the tasks of treatment planning, verification and delivery into one single entity, it may treat different target sizes and areas. Its scope of stereotactic treatment varies from small local tumors to whole body. However, a greater number of MUs and more treatment time are required.
HT has been widely used for cancer treatment in the world. The first unit was installed at our hospital in October, 2015. As demonstrated by existing researches, HT offered improved dose homogeneity in target and normal tissues for NPC as compared with IMRT (10). Over the last decade, IMRT and VMAT were employed mostly for NPC patients at our hospital. And it is imperative for clinicians to improve cure rate for locally advanced NPC patients without compromising quality-of-life. Here the authors intended to evaluate the dosimetric quality and effectiveness of HT for locally advanced NPC patients as compared with VMAT and IMRT. Our findings shall guide us to optimize HT clinically for NPC patients.
Methods
Patient characteristics
We conducted a retrospective study on 30 patients with locally advanced NPC who were randomly selected from our department. There were 22 males and 8 females with an average age of 37.1 (range, 23 to 57) years. All treatment regimens were designed with IMRT, VMAT and HT. Based upon the 7th AJCC/UICC staging system, their clinical stages were III (n=13) and IV (n=17). All subjects were free of distant metastases and none of them received any previous RT. All radiation procedures and potential adverse effects were fully explained. And a written consent form was signed.
Simulation and immobilization
All patients were scanned by a Siemens Plus4 Spiral computed tomography (CT) simulator for 3 mm slice thicknesses in a supine position with a head-and-shoulder thermoplastic mask. Scanning started from head to manubriosternal joint. And CT datasets were imported via a DICOM network into treatment planning system (TPS).
Target delineation and dose prescription
The same attending physician contoured the targets and OARs on the axial slices of planning CT scan. And target volumes were determined by the International Commission on Radiation Units & Measurements (ICRU) Reports 50 & 62. CTV1 was defined as the high-risk regions of microscopic extension encompassing whole nasopharynx and all neck nodes, included gross tumor (GTVnx) and regional metastatic nodes (GTVnd). The CTV2, defined as the low-risk region of neck nodes below CTV1, included low jugular and supraclavicular regions without lymph node metastasis. The GTVnx, GTVnd, CTV1 and CTV2 were all expanded by a 3-mm margin in all three directions for PGTVnx, PGTVnd, PTV1 and PTV2 to account for patient setup errors. OARs included brain stem, spinal cord, lens, optic nerves, optic chiasm, parotid glands, temporomandibular joints (TMJs), temporal lobes and other non-specified tissues.
The same medical physicist designed treatment plans and all three plans were redesigned for minimizing the influence of inter-operator variations on planning. IMRT and VMAT plans were created on Varian Eclipse TPS (Varian Medical System, USA) while HT plans formulated on TomoTherapy Hi-Art Planning Station.
All plans were delivered on a 6-MV linear accelerator. The dose prescriptions were 73.92 Gy/33 f to PGTVnx, 69.96 Gy/33 f to PGTVnd, 60.06 Gy/33 f to PTV1 and 50.96 Gy/28 f to PTV2. OARs and targets dose constraints from the protocol of Radiation Therapy Oncology Group (RTOG)-0615 were as follows: brain stem (max 54 Gy), spinal cord (max 45 Gy), no more than 50% of parotids (30 Gy) or a mean dose of at least one side of parotid glands (<26 Gy). The dose to other OARs was minimized within a reasonable range to ensure the best coverage of target area (Table 1). For targets, the treatment goals were that the maximal dose would not exceed 110% and 100% of prescribed dose would cover 95% of planning target volume (PTV) volume.
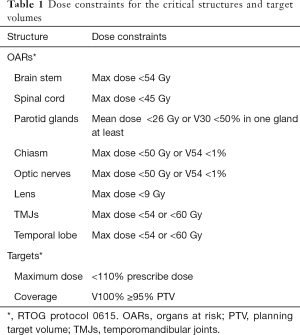
Full table
Planning techniques
IMRT plan
Dynamic intensity modulated radiation therapy (IMRT-Sliding window) was used. And IMRT plans were generated with 9 coplanar fields (9F-IMRT). A medical physicist would customize the position, size and angle of collimator. Dose Volume Optimizer (Varian Eclipse version 11.0.31) algorithm of Eclipse TPS was utilized for 9F-IMRT plan optimization. The plans were iteratively optimized by inverse planning software for optimal PTV coverage and OARs sparing. Final dose distribution was calculated by the Anisotropic Analytical Algorithm (AAA, version 11.031) dose algorithm with a calculation grid size of 2.5 mm.
VMAT plan
VMAT plans were generated using two complementary coplanar arcs of 360° (one counter-clockwise from 179° to 181°, one clockwise from 181° to 179°). A VMAT double-arc plan (2ARC-VMAT) was optimized by progressive resolution of Eclipse TPS (version 11.0.31). The optimization objectives of 2ARC-VMAT plans were the same as those of 9F-IMRT plans. For minimizing leakage, tongue and groove effects, collimator angle varied between 0° and 15° according to the shape of target. Other planning parameters were MLC motion speed 0 to 2.5 cm/s, gantry rotation speed 0.5 to 4.8 degrees/s and dosing rate 0 to 600 MU/min. The final dose distribution was calculated by AAA algorithm with a grid size of 2.5 mm.
HT plan
HT plans were optimized with TomoTherapy Hi-Art Software (Version 2.0.7) (Accuray, Madison, WI, USA). Three major parameters were set by operator: a field width of 2.5 cm, a pitch of 0.287 and a modulation factor of 2.1–2.6. A collapsed cone convolution model was employed for dose calculating with a grid size of 1.95 mm.
Dose-volume histograms (DVHs) and dose comparisons
A standard DVH was used for evaluating the quality and quantity of PTVs according to ICRU83. The dose values covering 98% (D98) and 2% of PTV (D2) were calculated as metrics for minimal and maximal doses respectively. The criteria of dosimetric comparison were as follows:
- Homogeneity index (HI): measurement of how even the dose distributes in PTV1 and PTV2. Formula: HI = (D2% − D98%)/D50%. A higher HI means the actual dose received by target is greater than prescribed dose. It implies poorer homogeneity;
- Conformity index (CI): measurement of how conformed the dose distribution is along target volume of PTV1 and PTV2. Formula: CI = (TVPV × TVPV)/(VPTV × VTV). CI value <1 and a higher CI denoted better dose conformity in PTV (VPTV: volume of PTV, VTV: treatment volume of body receiving 95% of prescribed dose and TVPV: volume of VPTV within VTV) (11);
- OARs: normal tissue doses of IMRT, VMAT and HT plans were calculated. Maximal dose (Dmax), mean dose (Dmean) and receiving dose of 1 cubic centimeter (D1cc) of spinal cord, brainstem, as well as D50 and D33 were recorded and compared for contralateral and ipsilateral parotids. The maximal and mean doses were also determined for various organs (optic nerves & optic chiasm), parallel organs (double lens, double TMJ & double temporal lobes) and posterior neck muscles;
- Normal tissue outside PTV: the strategy for normal tissue was to minimise its involvement. The volumes of normal tissue to receive 5 Gy, 10–60 Gy were recorded for the three plans;
- Treatment time and MUs: treatment time was calculated from the first beam-on to the last beam being turned off, including time for radiation delivery and gantry rotation, but not time for patient setup and image comparison.
Statistical analysis
Paired t-test was used for comparing the dosimetric differences in IMRT versus VMAT, IMRT versus HT and VMAT versus HT. P<0.05 was considered statistically significant. All analyses were performed using SPSS statistical software (Version 19.0) (SPSS, IL, Chicago, USA).
Results
PTV coverage
Dose distribution in all plans fulfilled clinical requirements. Typical dose distributions produced by each of three techniques in one representative case were summarized in Figure 1. CI and HI of PTVs were tabulated in Table 2. The coverage of PTVs of all the three plans was evaluated by comparing the target volumes receiving 95% of the prescribed dose (V95%). In PTV1, V95% in IMRT, VMAT and HT were 97.10%±0.66%, 97.76%±1.34% and 97.43%±0.88% respectively. While in PTV2, V95% were 98.65±1.94%, 98.14±1.00% and 97.85±1.42% in IMRT, VMAT and HT respectively. All plans had similar PTV coverage and all fulfilled the prescription requirements. However, no significant difference existed in target coverage among IMRT, VMAT and HT plans. Both IMRT (PTV1 HI: 0.16±0.03, PTV2 HI: 0.31±0.02) and HT (PTV1 HI: 0.16±0.03, PTV2 HI: 0.30±0.02) plans had a better homogeneity than VMAT (PTV1 HI: 0.20±0.03, PTV2 HI: 0.33±0.03) plan in PTVs. HT (PTV1 CI: 0.80±0.04, PTV2 CI: 0.66±0.03) showed superior dose conformity as compared with IMRT (PTV1 CI: 0.74±0.04, PTV2 CI: 0.62±0.03) and VMAT (PTV1 CI: 0.75±0.03, PTV2 CI: 0.63±0.03) in PTVs. VMAT had a better conformity than IMRT, but it had no statistical significance.
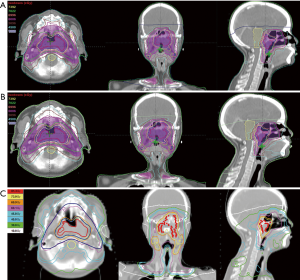
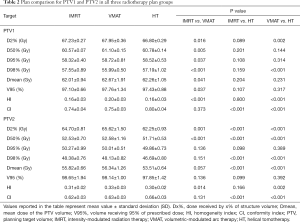
Full table
Dose to OARs
PRV of brain stem
As compared with IMRT, VMAT and HT performed better in sparing of brain stem. Especially, HT obtained significantly lower values. A dose reduction of Dmax by 4.76±5.57 Gy was obtained from HT to IMRT and 1.76±4.59 Gy from HT to VMAT. Dmean decreased by 3.12±3.08 Gy from HT to IMRT and 2.67±3.89 Gy from HT to VMAT. And D1cc decreased by 3.07±3.92 Gy from HT to IMRT and 2.49±3.97 Gy from HT to VMAT.
PRV of spinal cord
The planning objective of Dmax ≤45 Gy for spinal cord was fulfilled by all techniques. Similar to brain stem, the mean values of Dmax, Dmean and D1cc were significantly lower in HT as compared with IMRT and VMAT. A dose reduction of Dmax by 2.99±2.07 Gy was obtained from HT to IMRT and 1.69±2.40 Gy from HT to VMAT while the mean values of Dmean of HT to spinal cord were reduced by >5 Gy as compared with the other two techniques.
Parotid glands
Ipsilateral and contralateral parotids were analyzed separately. As expected, larger sparing was observed for the contralateral parotid glands. HT reduced the involvement of both parotid glands more effectively as compared with IMRT/VMAT. As compared with those of IMRT/VMAT, the mean values of Dmean of HT to ipsilateral and contralateral parotids decreased by more than 10 Gy. Yet dosimetric difference between VMAT and IMRT remained small.
Visual apparatus
Both lens, optic nerves and chiasm were analyzed. As compared with those of IMRT and VMAT, HT had significantly lowered the mean values of Dmax and Dmean to lens. For left lens, a dose reduction of Dmax by 4.49±1.93 Gy was obtained from HT to IMRT and 1.94±1.03 Gy from HT to VMAT. For right lens, a dose reduction of Dmax by 3.70±1.58 Gy was obtained from HT to IMRT and 2.31±1.12 Gy from HT to VMAT, while the doses to optic nerves and chiasm was the largest in HT. VMAT achieved the largest dose reduction in both optic nerves and chiasm. As compared with IMRT/HT, VMAT reduced the Dmax of chiasm by 1.64±3.62 and 7.02±8.65 Gy respectively.
TMJs
Among three plan groups, the maximal doses to TMJs in HT plans were little higher than those of VMAT/IMRT and no significant difference existed in Dmax for right TMJ. However, the mean doses to both TMJs in HT were lower than those of VMAT/IMRT. A dose reduction of Dmean by 5.63±4.13 Gy was obtained from HT to IMRT and 4.01±4.77 Gy from HT to VMAT for left TMJ. A dose reduction of Dmean by 5.45±7.17 Gy was obtained from HT to IMRT and 5.79±6.29 Gy from HT to VMAT for right TMJ.
Temporal lobes
The maximal doses to left temporal lobe in HT were slightly lower than those of VMAT/IMRT. However, the mean doses to both temporal lobes in HT were higher than those of VMAT/IMRT. No significant difference existed in IMRT/VMAT for the mean doses to both temporal lobes.
Posterior neck
The maximal and mean doses of posterior neck in HT were the lowest as compared with IMRT/VMAT (P<0.05). A dose reduction of Dmax by 8.38±1.49 Gy was obtained from HT to IMRT and 8.31±1.89 Gy from HT to VMAT. And a dose reduction of Dmean by 4.07±1.04 Gy was obtained from HT to IMRT and 1.87±2.05 Gy from HT to VMAT. Dosimetric comparison for OARs was shown in Table 3.
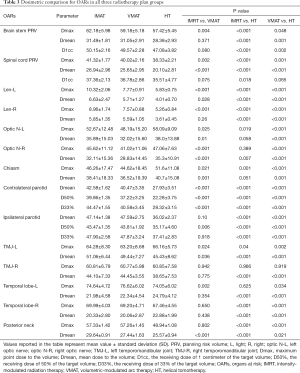
Full table
Normal tissue outside PTV
The relative volumes of different doses to normal tissue of three radiotherapy plans were measured. As seen in Table 4, V5, V10, V20, V30, V50 and V60 in HT plans were all lower than those in IMRT and VMAT plans. However, only V5, V10, V20 and V60 in HT had statistical significance as compared with IMRT and VMAT. VMAT had the highest volume in V5 and V10 as compared with IMRT and HT (P<0.05). Average DVHs for PTV and selected OARs are presented in Figure 2.
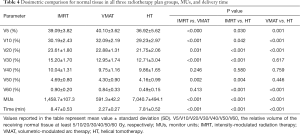
Full table
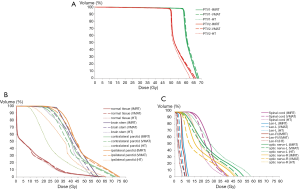
MUs and delivery time
MUs and delivery time of three radiotherapeutic plans were summarized in Table 4. The mean values of MUs were 1,458.7±107.3 in IMRT, 591.3±42.2 in VMAT and 7,040.7±494.1 in HT. The mean delivery time was 8.47±0.53 min in IMRT, 2.27±0.27 min in VMAT and 7.81±0.52 min in HT. As compared with HT and IMRT, VMAT significantly reduced MUs and average treatment time (P<0.05).
Discussion
It was previously reported that HT techniques achieved comparable or better treatment plans for head-and-neck cancer as compared to IMRT and VMAT (12,13). However, there are still few studies of comparing HT, IMRT and VMAT for NPC. Different institutions vary greatly in prescriptions, optimization methods & systems, dosimetric parameters and planning systems. Therefore it is rather difficult to compare planning studies at different treatment centers. Here the authors intended to quantify the potential dosimetric gains of HT versus IMRT/VMAT for late-stage NPC at our department.
In the present study, all target coverages for PTV1 and PTV2 in IMRT, VMAT and HT techniques fulfilled clinical requirements. And HT plans significantly improved target dose homogeneity and conformity. IMRT plans achieved better homogeneity than VMAT plans. Lee et al. (12) analyzed the potential dosimetric gains of HT versus step-and-shoot intensity-modulated radiotherapy (SaS-IMRT) for 20 NPC patients. HT could significantly improve conformity index and homogeneity index of PTV54 as compared with SaS-IMRT. As reported by Lu et al. (14), VMAT, HT and IMRT plans for NPC had comparable PTV coverage with an average of 96%. The homogeneity indices of VMAT and HT were better than that those of IMRT (P<0.05). And HT provided a better conformity index than those of VMAT and IMRT (P<0.05).
In our study, IMRT had better homogeneity indices than VMAT. Similar results have been reported elsewhere. Johnston et al. (15) evaluated VMAT and IMRT for 10 patients with locoregionally advanced oropharynx or nasopharynx carcinoma. The coverage of 95% of PTV70 was between 96% and 100% of prescribed dose for IMRT plans and 100% for all VMAT plans. There was an overall trend of improved dose homogeneity for IMRT plans in both PTV63 and PTV56. Guckenberger et al. (16) suggested that the dosimetric results depended upon target location, contouring of OARs, margin of CTV to PTV and dosimetric endpoint. And the differences between VMAT and s-IMRT were also correlated with using standard s-IMRT treatment as a baseline for comparison.
With regards to dose to OARs, VMAT and HT provided better sparing of OARs as compared with IMRT. HT could significantly reduce the dose to brain stem and spinal cord. Furthermore, sparing of OARs was better achieved in HT and VMAT plans versus IMRT plans for head-and-neck cancer (12,14).
For parotid glands, HT could lower median dose values as compared with VMAT/IMRT and VMAT technique was superior to IMRT (17). In another study (14), the mean dose to parotid glands was comparable between VMAT (26.3 Gy) and HT (27.5 Gy). Yet both were better than IMRT (31.3 Gy, P<0.01). In the present study, VMAT and IMRT showed equivalent sparing effects for parotids and the mean dose for parotids was reduced by >10 Gy in HT as compared with VMAT/IMRT.
Grégoire et al. (18) had confirmed that residual salivary output for head-and-neck cancer was associated with the mean doses to parotids during treatment. Li et al. (19) reported that saliva production was affected significantly by the dose of radiation. However, at a mean dose of <25–30 Gy to parotid glands, recovery became substantial and returned to pretreatment levels at 2 years post-RT. Therefore it was beneficial to lower the mean dose of parotid glands. In the present study, the doses to parotid glands failed to meet the dose constraints due to a partial overlap with PTV and contouring of parotid gland. Furthermore, upper cervical nodal coverage was in higher to intermediate dose volumes. All of the above caused a higher dose to parotid glands.
HT could significantly reduce the dose to lens as compared with VMAT/IMRT. However, for optic nerve and optic chiasm, HT resulted in a higher Dmax and Dmean than VMAT/IMRT. Another study (14) made a dosimetric comparison with NPC of stage I–IV. IMRT reduced the maximal dose to optic nerve by 22.8% (stage I–II) and 21.3% (stage III–IV) and VMAT 24.0% (stage I–II) and 27.8% (stage III–IV) respectively versus HT separately. VMAT was superior to IMRT when comparing the dose to optic nerve for stage III-IV NPC. VMAT could better protect optic nerve. The result was consistent with that of Lee et al. (12). HT versus IMRT offered a higher dose to optic chiasm and this phenomenon tended to be more common in T3/T4 patients since all enrolled patients were within stage III-IV.
Bony structures of our study were TMJs. As compared with IMRT/VMAT, HT plans lowered the mean dose of TLMs and the maximal dose was comparable among three different techniques. It is well-known that a high dose to bone structures causes osteoradionecrosis and radiation-induced trismus is one of the most common late complications for NPC patients after RT. Fang et al. (20) evaluated the health-related quality-of-life for 182 NPC patients after RT. And 30% of survivors had severe trismus. As reported by other studies, when TMJs and masseter muscles were subject to radiation exposure, 50% of patients had difficulties of mouth opening (21). Thus it is imperative to lower the doses to TMJs and mandible.
For IMRT, radiation-induced brain necrosis is uncommon. In the present study, the mean dose of temporal lobes was higher in HT than that in VMAT/IMRT. And the maximal dose to temporal lobes was much higher than those of previous studies (22). Contouring of temporal lobes may be a partial reason. And intracranial invasion is often found adjacent to temporal lobes.
For soft tissues, HT could effectively reduce the maximal dose and mean dose of posterior neck. Reduced dose to posterior neck is essential for minimizing such late toxicities as neck fibrosis. Post-irradiation neck fibrosis is one of the most common complications of RT for NPC patients. It was previously reported that 22% NPC patients had severe neck stiffness after RT (20). Neck fibrosis causes neck discomfort and restricts head-turning.
For normal tissues, HT had the lowest volume at different doses. So HT could lower integral dose to body and reduce the volume at a low dose of radiation. With a highest volume in V5 and V10, VMAT increased the volume at a low dose of radiation. Some studies reached a similar conclusion. Bertelsen et al. (23) reported that high-dose volumes in healthy tissue (>15 Gy) decreased in VMAT versus IMRT for 25 oropharyngeal and hypopharyngeal cancer patients. However, some studies reported that VMAT had a greater volume at a lower range of radiation dose (24). Future clinical studies should be designed to clarify the impact of VMAT on the incidence of radioactive secondary tumors.
In the present study, VMAT showed decreases in MU and treatment time as compared with IMRT/HT. Some studies reported that VMAT could significantly reduce treatment time and MU (25). Shorter treatment time not only greatly improved patient comfort, but also increased the number of patients treated per unit of time. And the efficiency of equipment utilization was also enhanced. Most importantly, shorter treatment time helped the patients maintain a steady posture and minimize setup errors so as to reduce the margin of PTV and lower the irradiation dose to normal adjacent tissue. Some in vitro experiments also suggested that shorter treatment time helped to reduce sublethal damage repair halftime (26). So it could greatly facilitate the biological effects of radiation. Fewer MUs of VMAT might decrease scattered radiation and integral radiation dose to patients, thereby reducing the occurrences of radiation-induced tumors (6).
Conclusions
All techniques may yield plans with an excellent coverage of PTVs for locally advanced NPC. However, HT offers optimal dosimetric outcomes of improving target dose homogeneity and conformity and reducing the dose of OARs. And VMAT has a comparable or better dose distribution as compared with IMRT. However, there is still room for improvement in terms of OAR sparing. Additionally, VMAT significantly reduces treatment time and MUs. Future studies are required to clarify the impact of reduced delivery time on clinical outcomes.
Acknowledgments
Funding: This work was supported by the National Natural Science Foundation of China (81472847) and a grant (2012 No. 1493) from the Development and Reform Commission of Hunan province of China.
Footnote
Conflicts of Interest: All authors have completed the ICMJE uniform disclosure form (available at http://dx.doi.org/10.21037/tcr.2017.09.48). The authors have no conflicts of interest to declare.
Ethical Statement: The authors are accountable for all aspects of the work in ensuring that questions related to the accuracy or integrity of any part of the work are appropriately investigated and resolved. The study was conducted in accordance with the Declaration of Helsinki (as revised in 2013). The study is retrospective and does not involve the treatment of patients, it did not violate ethics. Institutional ethical approval was waived. A written consent form was signed.
Open Access Statement: This is an Open Access article distributed in accordance with the Creative Commons Attribution-NonCommercial-NoDerivs 4.0 International License (CC BY-NC-ND 4.0), which permits the non-commercial replication and distribution of the article with the strict proviso that no changes or edits are made and the original work is properly cited (including links to both the formal publication through the relevant DOI and the license). See: https://creativecommons.org/licenses/by-nc-nd/4.0/.
References
- Sun X, Su S, Chen C, et al. Long-term outcomes of intensity-modulated radiotherapy for 868 patients with nasopharyngeal carcinoma: an analysis of survival and treatment toxicities. Radiother Oncol 2014;110:398-403. [Crossref] [PubMed]
- Zhou Q, He Y, Zhao Y, et al. A study of 358 cases of locally advanced nasopharyngeal carcinoma receiving intensity-modulated radiation therapy: Improving the Seventh Edition of the American Joint Committee on Cancer T-Staging System. Biomed Res Int 2017;2017:1419676.
- Kuang WL, Zhou Q, Shen LF. Outcomes and prognostic factors of conformal radiotherapy versus intensity-modulated radiotherapy for nasopharyngeal carcinoma. Clin Transl Oncol 2012;14:783-90. [Crossref] [PubMed]
- Wang W, Yang H, Mi Y, et al. Rules of parotid gland dose variations and shift during intensity modulated radiation therapy for nasopharyngeal carcinoma. Radiat Oncol 2015;10:3. [Crossref] [PubMed]
- Su J, Chen W, Yang H, et al. Different setup errors assessed by weekly cone-beam computed tomography on different registration in nasopharyngeal carcinoma treated with intensity-modulated radiation therapy. Onco Targets Ther 2015;8:2545-53. [PubMed]
- Mansur DB, Klein EE, Maserang BP. Measured peripheral dose in pediatric radiation therapy: a comparison of intensity-modulated and conformal techniques. Radiother Oncol 2007;82:179-84. [Crossref] [PubMed]
- Teoh M, Clark CH, Wood K, et al. Volumetric modulated arc therapy: a review of current literature and clinical use in practice. Br J Radiol 2011;84:967-96. [Crossref] [PubMed]
- Winkler C, Duma MN, Popp W, et al. Protection of quality and innovation in radiation oncology: the prospective multicenter trial QUIRO of DEGRO: evaluation of time, attendance of medical staff, and resources during radiotherapy with tomotherapy. Strahlenther Onkol 2014;190:950-6. [Crossref] [PubMed]
- Widesott L, Pierelli A, Fiorino C, et al. Intensity-modulated proton therapy versus helical tomotherapy in nasopharynx cancer: planning comparison and NTCP evaluation. Int J Radiat Oncol Biol Phys 2008;72:589-96. [Crossref] [PubMed]
- Broggi S, Perna L, Bonsignore F, et al. Static and rotational intensity modulated techniques for head-neck cancer radiotherapy: a planning comparison. Phys Med 2014;30:973-9. [Crossref] [PubMed]
- Feuvret L, Noël G, Mazeron JJ, et al. Conformity index: a review. Int J Radiat Oncol Biol Phys 2006;64:333-42. [Crossref] [PubMed]
- Lee TF, Fang FM, Chao PJ, et al. Dosimetric comparisons of helical tomotherapy and step-and-shoot intensity-modulated radiotherapy in nasopharyngeal carcinoma. Radiother Oncol 2008;89:89-96. [Crossref] [PubMed]
- Van Gestel D, van Vliet-Vroegindeweij C, Van den Heuvel F, et al. RapidArc, SmartArc and TomoHD compared with classical step and shoot and sliding window intensity modulated radiotherapy in an oropharyngeal cancer treatment plan comparison. Radiat Oncol 2013;8:37. [Crossref] [PubMed]
- Lu SH, Cheng JC, Kuo SH, et al. Volumetric modulated arc therapy for nasopharyngeal carcinoma: a dosimetric comparison with TomoTherapy and step-and-shoot IMRT. Radiother Oncol 2012;104:324-30. [Crossref] [PubMed]
- Johnston M, Clifford S, Bromley R, et al. Volumetric-modulated arc therapy in head and neck radiotherapy: a planning comparison using simultaneous integrated boost for nasopharynx and oropharynx carcinoma. Clin Oncol (R Coll Radiol) 2011;23:503-11. [Crossref] [PubMed]
- Guckenberger M, Richter A, Krieger T, et al. Is a single arc sufficient in volumetric-modulated arc therapy (VMAT) for complex-shaped target volumes? Radiother Oncol 2009;93:259-65. [Crossref] [PubMed]
- Wiezorek T, Brachwitz T, Georg D, et al. Rotational IMRT techniques compared to fixed gantry IMRT and tomotherapy: multi-institutional planning study for head-and-neck cases. Radiat Oncol 2011;6:20. [Crossref] [PubMed]
- Grégoire V, De Neve W, Eisbruch A, et al. Intensity-modulated radiation therapy for head and neck carcinoma. Oncologist 2007;12:555-64. [Crossref] [PubMed]
- Li Y, Taylor JM, Ten Haken RK, et al. The impact of dose on parotid salivary recovery in head and neck cancer patients treated with radiation therapy. Int J Radiat Oncol Biol Phys 2007;67:660-9. [Crossref] [PubMed]
- Fang FM, Chiu HC, Kuo WR, et al. Health-related quality of life for nasopharyngeal carcinoma patients with cancer-free survival after treatment. Int J Radiat Oncol Biol Phys 2002;53:959-68. [Crossref] [PubMed]
- Bhrany AD, Izzard M, Wood AJ, et al. Coronoidectomy for the treatment of trismus in head and neck cancer patients. Laryngoscope 2007;117:1952-6. [Crossref] [PubMed]
- Sun Y, Guo R, Yin WJ, et al. Which T category of nasopharyngeal carcinoma may benefit most from volumetric modulated arc therapy compared with step and shoot intensity modulated radiation therapy. PLoS One 2013;8:e75304 [Crossref] [PubMed]
- Bertelsen A, Hansen CR, Johansen J, et al. Single Arc Volumetric Modulated Arc Therapy of head and neck cancer. Radiother Oncol 2010;95:142-8. [Crossref] [PubMed]
- Vanetti E, Clivio A, Nicolini G, et al. Volumetric modulated arc radiotherapy for carcinomas of the oro-pharynx, hypo-pharynx and larynx: a treatment planning comparison with fixed field IMRT. Radiother Oncol 2009;92:111-7. [Crossref] [PubMed]
- Clemente S, Wu B, Sanguineti G, et al. SmartArc-based volumetric modulated arc therapy for oropharyngeal cancer: a dosimetric comparison with both intensity-modulated radiation therapy and helical tomotherapy. Int J Radiat Oncol Biol Phys 2011;80:1248-55. [Crossref] [PubMed]
- Qi XS, Yang Q, Lee SP, et al. An Estimation of Radiobiological Parameters for Head-and-Neck Cancer Cells and the Clinical Implications. Cancers (Basel) 2012;4:566-80. [Crossref] [PubMed]