The relative biological effectiveness of proton and carbon ion beams in photon-sensitive and resistant nasopharyngeal cancer cells
Introduction
Nasopharyngeal cancer (NPC) is one of the most commonly diagnosed head and neck malignancies in Southern China (1). Radiation therapy is the only curative treatment for NPC (2), and intensity-modulated radiation therapy (IMRT) is currently the standard technology. Despite a significant improvement in local control and survival after the prevailing use of IMRT, approximately 10–15% patients will fail locally after definitive radiotherapy (3-6). Presently, re-irradiation by a second course of IMRT remains the principle modality for patients with locally recurrent NPC (LR-NPC). However, the outcome after such aggressive treatment is dismal (7-9). Recently, Kong et al. indicated that the use of salvage IMRT for LR-NPC after a previous course of IMRT with curative intent may represent a distinct clinical condition with more suboptimal outcomes (6). In addition, locally recurrent disease may harbor radio-resistant cancer cells induced by the initial irradiation (10). Clearly, a novel therapeutic modality for LR-NPC in the IMRT era are needed (2).
Charged particle therapy, such as proton beam and carbon ion beam radiotherapy (PRT and CIRT) are cutting edge technologies in radiation oncology (11-13). They deposit energy in a significantly more selective fashion than X-rays (14), allowing for more precise tumor volume coverage, thereby, improved local control and lower damage and complications to the organs at risk (OAR) (15). Furthermore, the potential advantages of CIRT include a higher relative biological effectiveness (RBE) and reduced oxygen enhancement ratio (OER) (14,16); an increased rate of direct DNA double strand breaks (17), an improved efficacy against radio-resistant tumors (18-25); a low cell-cycle dependence; a strong immunological responses (26), angiogenesis inhibition (27), and anti-metastatic potential (28). The use of CIRT for previously irradiated sites with high dose X-ray has been reported for adenoid cystic carcinoma (29), chordoma, chondrosarcoma (30), and squamous cell carcinoma of the head and neck (HNSCC) (31), with favorable dosimetry, encouraging local tumor control, and acceptable toxicity. A number of studies have reported superior dose distributions using particle therapy for primary or recurrent NPC with acceptable clinical outcomes (32-34). Furthermore, the results reported by Feehan et al. showed that heavy charged particles (Helium and Neon) appear to have a superior effect, with no fatal complications, when compared to photon therapy in locally advanced, recurrent NPC (33). As such, CIRT may offer an ideal alternative to conventional X-ray irradiation. Trials which evaluate CIRT, with or without concurrent chemotherapy, for the salvage treatment of LR-NPC are ongoing at the Shanghai Proton and Heavy Ion Center (SPHIC) (35,36).
Despite the active, ongoing research within the clinical setting, the RBEs for photon-sensitive and resistant nasopharyngeal carcinoma (prNPC) have not yet been determined for charged particle therapy. Therefore, we investigated the RBEs for proton and carbon ion beams in this study by establishing prNPC cell line (CNE-2R) from its parental cell line (CNE-2) by a rigorous irradiation protocol. Results suggest that carbon ions have superior potential to kill photon-resistant cancer cells (CNE-2R) compared to the photon-sensitive cancer cells (CNE-2). Our results may have implications in improving the radiotherapy of photon-resistant NPC in patients who failed to benefit from the primary radiotherapy for NPC.
Methods
Cell culture and establishment of X-ray resistant cells
A NPC cell line CNE-2, and its radio-resistant variant CNE-2-IR (kindly provided by the Center for Molecular Medicine, Xiangya Hospital, Central South University, Changsha, China) were cultured in RPMI-1640 supplemented with 10% fetal bovine serum (FBS) in a 37 °C incubator with humidified atmosphere and 5% CO2. The CNE2-IR cell line was established as previously described (37). Briefly, exponentially growing CNE-2 cells were seeded at a density of 1×105 cells per T25 flask and subjected to a series of gradually increasing doses of X-ray irradiation exposure. The CNE-2 cells were cultured and passaged twice following the initial irradiation and were then exposed to an additional dose of X-ray irradiation. The total X-ray dose was 60 Gy (2 fractions of 2, 4, 6, 8 and 10 Gy X-ray irradiation), and the entire selection procedure was completed over 5 months.
We then established a highly resistant population by irradiating the radio-resistant variant CNE-2-IR cells with additional 40 Gy (4 fractions of 10 Gy X-ray irradiation) over an additional 4 months and designated it as CNE-2R (Figure 1A). To verify the radio-resistant phenotypes of CNE-2R, both CNE-2R and CNE-2 were irradiated using X-rays with a range of radiation doses (2–10 Gy) and assessed for their clonogenic survival by macrocolony assay. The surviving colonies of the CNE-2R cells, which showed increased radio-resistance compared with the level of its control CNE-2 (Table 1), were significantly more than CNE-2 cells (Figure 1B) at 0–10 Gy.
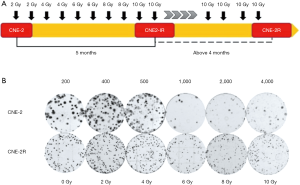
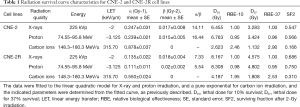
Full table
Before we used the prNPC cell line (CNE-2R) to model extensively irradiated NPC cells by photon therapy and determine their RBE for carbon ion beams, we conducted cell line STR genotyping of 20 gene loci to confirm authenticity and genomic differences. Their gene markers and alleles are shown (Figures S1,S2, Table S1), which are similar to the earlier report (38). These results confirmed the extremely X-ray resistant nature of the extensively photon-irradiated cell (CNE-2R).
Cell line short tandem repeat (STR) genotyping
CNE-2 and its extensively photon-irradiated model, CNE-2R, were collected for genomic DNA (gDNA) extraction, which was followed by STR genotyping. The entire process was performed by Shanghai Biowing Applied Biotechnology Co. LTD, Shanghai, China.
Proton and carbon ion irradiation (CIRT)
Proton irradiation (PRT) and CIRT planning was performed using the Syngo® treatment planning system (Siemens, Erlangen, Germany) including biologic plan optimization. To build a spread-out Bragg peak (SOBP), several beam spots with distinct initial energies were superimposed. PRT and CIRT were performed using the IONTRIS® intensity-modulated raster scan system with a homogeneous SOBP with energy of 74.55–95.8 MeV and 148.3–180.3 MeV/u respectively on the target obtained from the heavy-ion synchrotron accelerator (Siemens AG) at the SPHIC, Shanghai, China. The average linear energy transfer (LET) was approximately 3.125 keV/µm for PRT and 315.7 keV/µm for CIRT. A horizontal beam line was used for PRT and CIRT. Tumor cells plated at a density of 40×103/cm2 (1×106/T25 tissue culture flask) were irradiated to a range of PRT (0, 2, 4, 6, 8 and 10 Gy) and CIRT doses (0, 2, 4, 6 and 8 Gy) at the middle of SOBP beams. The irradiation depth was controlled by changing the thickness of water-equivalent material placed in front of the cell culture flasks. The flasks were mounted on a remote-controlled sample changer standing vertically to face a horizontal beam line. The cells were irradiated as attached to the flask wall and the entire flask was encompassed by an irradiation field (Figure 2). While irradiated, the flasks were filled up with culture media and fixed in a fabricated mold with 37 °C water.
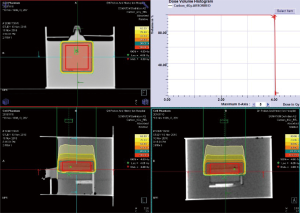
X-ray irradiation
A 225 kVp X-ray beam (PXi precision X-RAD 225, dose rate =3.198 Gy/min, 225 kV, 13.3 mA, 40 cm SSD) was used as the reference photon beam (39). Briefly, cells were plated in T25 tissue culture flasks and were irradiated to 0, 2, 4, 6, 8, and 10 Gy, respectively.
Clonogenic survival assay
Twelve to 16 hours after irradiation, the cells were trypsinized, harvested, counted and plated in 6-well plates for a colony-forming assay and cultured for 7–14 days in RPMI-1640 supplemented with 10% FBS. Only colonies of ≥50 cells were scored, and the data were fitted to the linear quadratic (LQ) equation {Eq. [1]} for X-ray and PRT (40). For high LET (315.7 keV/µm) carbon ion-exposed samples, the fit with the LQ model of the curves resulted in negative β-values for carbon ions in CNE-2R (−0.016±0.013) and CNE-2 (−0.050±0.021) cells and the curves were then fitted with a purely exponential {Eq. [2]} for both cell lines. In this case β can be regarded as 0 (41,42). Based on the data from PIDE, it has been suggested that β rises first and then decreases with rising LET (41). For intermediate and high LET values many data show a vanishing β term (41). As β values indicate repairable portion of the cells irradiated, the extremely low β values (close or equal to zero) with high LET is not surprising, as carbon ion beams largely induce DNA double strand break:
where, SD = the surviving fraction (SF) of cells exposed to radiation dose (D), S0 = the SF of un-irradiated cells, α (Gy−1) = the single-hit inactivation coefficient and β (Gy−2) = the maximal double-hit inactivation coefficient (no repair).
D10 (lethal dose for 10% survival), D37 (lethal dose for 37% survival), SF2 (SF after 2 Gy irradiation) were determined from the fitted curve. RBE 10 and RBE 37 values were calculated as the ratio of the D10 and D37 of PRT and CIRT to those of 225 kV X-rays. Moreover, we also calculated the Dq (quasi-threshold dose and capacity for sub-lethal damage repair) and D0 (cellular radiosensitivity and represent the radiation dose that led to 37% cell survival) of the NPC cells by fitting the data to the multi-target, single-hit model, SD/S0=1−[1− exp (−k × D)]N. The relationship between Dq, D0, k and N is given as follows:
Where, k (slope) and n (the extrapolation number) were determined from the fitted curve.
Statistical analysis
Statistical analysis was performed using the GraphPad Prism version 7.00 for Windows (GraphPad Software, San Diego California, USA, www.graphpad.com). The statistical testing is detailed in the legends of the figures.
Results
RBE calculation and determination of α- and β-parameters
Based on survival curves, the parameters for cellular radio-sensitivity, including D10, D37, α, and β values, were calculated by a fitting using the LQ model (Table 1, Figure 3). Table 1 demonstrates the α-values, β-values, α/β ratios (the dose at which the two terms contribute equally to the total effect) of the CNE-2 (0.247±0.031, 0.017±0.008, and 14.11, respectively) and CNE-2R cells (0.135±0.022 and 0.018±0.004 and 7.33, respectively) following X-ray irradiation. Given that the α/β ratio is traditionally considered as a measure of radio-sensitivity (43) and is used to classify the tissue type for clinical applications (41), this classified CNE-2R cells as particularly radio-resistant and CNE-2 cells as highly radiosensitive. Since the LQ model would not always give a comprehensive insight in to the dose response (44,45), we also fitted the data to multi-target single hit model and compared the different parameters like Dq and D0 in the sensitive and resistant cells. As expected the values of “n” for carbon are lower for both CNE2 and CNE-2R cells compared to X-rays and proton (Table 2). However, the values of “n” are less than 1 for carbon and correspondingly, the Dq values are negative. Although the negative values have no meaning, phenomenological implication is that they are more sensitive to carbon than X-rays and proton irradiation.
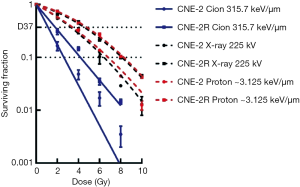
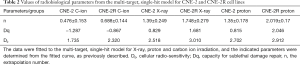
Full table
The proton cell survival curves demonstrated similar profiles to those of X-rays for CNE-2 and CNE-2R cell lines. The RBE at the D10 was 0.95 for CNE-2 cells as compared with 0.98 for CNE-2R cells, respectively. Paganetti’s review (40) highlighted that there is a trend of an increase in RBE as (α/β)x (i.e., the ratio between the linear and the quadratic term of the LQ model for the reference photon radiation) decreases. Our results indicated that the difference in RBE values for CNE-2 cells with higher (α/β)x and that of CNE-2R cells with lower (α/β)x is not appreciable.
The α-values were 3 to 4 folds higher for CIRT for both CNE-2 (0.878±0.037) and CNE-2R (0.550±0.024) as compared to those for X-rays (0.247 and 0.135 respectively for CNE-2 and CNE-2R) reflected by a steeper decline of the initial slope of the survival curves for 315.7 keV/µm LET beams (Figure 3, Table 1
Discussion
Re-irradiation with IMRT, produced worse outcome, in-terms of OS and local control in NPC patients who failed locally after IMRT for their primary NPC as compared to those who failed conventional or 3D conformal radiotherapy (6). It is therefore reasonable to postulate that recurrent NPC after high-dose radiotherapy could be more resistant to re-irradiation using photons, since dose coverage and targeting limitations have been largely eliminated with IMRT. Early clinical experience at the SPHIC indicated that the patients salvaged with intensity-modulated CIRT for LR-NPC produced an 1-year OS of >98% (36,47). However, despite of the apparently more effective outcome of CIRT for LR-NPC, the RBEs of CIRT as compared to photon irradiation has never been investigated nor quantified.
Owing to good clinical outcomes of PRT for patients with NPC (48), in the present study, we have compared the effectiveness of both carbon beam and proton versus X-ray (photon) beam for the CNE-2 nasopharyngeal cancer cell and its photon-resistant counterpart CNE-2-R cells. For a proton beam (energy, 74.55–95.8 MeV; LET, ~3.125 keV/µm), which is another type of particle radiation with low LET, the cell killing effects did not differ substantially from X-rays, providing the RBE values of 0.95 and 0.98 for CNE-2 and CNE-2-R cell lines using D10 doses relative to that of X-rays, which are comparable with the earlier report on human chordoma cells (49) where RBE values for proton (energy, 70 MeV; LET, 1 keV/µm) was 0.89. Therefore, the previously observed clinical benefit of PRT may be due more to the improved ability to spare previously irradiated normal tissues rather than the enhanced cell-kill in the tumor. In general, a constant RBE of either 1.0 or 1.1 is estimated for clinical applications, but the RBE of protons has been reported to vary with many factors, such as particle type, energy, dose per fraction, number of fractions, and cell or tissue type,dose, LET and (α/β)x (40,50,51). We have observed that the RBE of carbon ion beams at the D10 and D37 was 2.46 and 2.90 for CNE-2 cells compared with 1.95 and 2.53 for CNE-2-R cells. This suggested that carbon ions had higher biological effectiveness as compared to X-ray irradiation and PRT, in killing both sensitive and resistant NPC cells. However, CNE-2R cells were comparatively less sensitive than the CNE-2 cells for either photon, proton or carbon beam. Collectively, these data support the use of CIRT for recurrent NPC, for both its dosimetric advantages over X-rays and cell-killing advantages over X-rays and PRT.
RBE is a complex measure which depends on both physical parameters (e.g., particle type and energy, dose, dose rate, dose delivery time and LET) as well as biological parameters [e.g., cell/tissue type (52), cell cycle phase, oxygen level, and endpoint (14,16)]. CIRT is more effective in the killing of both sensitive and resistant cell lines in all studies, as all RBEs reported are >1 for any cell lines studies, regardless of radio-sensitivities. Our findings are highly consistent with those reported by Maalouf et al. for high-LET CIRT. Although photon irradiation is effective in killing photon-sensitive cells, the RBE values of 3–4 for high-LET CIRT indicate a significantly more effective killing. Although our data and those reported by Maalouf indicate that high-LET is also more effective than X-ray for photon-resistant cells, the relative effectiveness is not as evident as that for sensitive cells. This observation suggests that photon-induced resistance may cause resistance to CIRT as well. Interestingly, the RBE value in CNE-2R cells in the present study was lower than it in CNE-2 cells following 315.7 keV/µm carbon ion irradiations compared with X-rays. This finding is consistent with the Maalouf’s observation that the RBE value in SQ20B cells, a radioresistant p53 mutated head and neck squamous cell carcinoma (HNSCC) cell lines, was less than observed in radiosensitive SCC61 cells following 184 keV/µm carbon ion irradiations when compared with X rays (18).
The RBE at D10 for low-LET carbon beam (33.6 keV/µm) has been reported to be 1.5 and 4.2 for high LET (184 keV/µm) carbon beam in SCC61 cells as compared with 2.1 and 2.8 for SQ20B cells, respectively (18). At lower LET (33.6 keV/µm), although CIRT is more effective in cell killing for both sensitive and resistant cell lines (as RBE =1.5 and 2.1 respectively), the RBE for sensitive cell lines are substantially lower than that of high-LET (RBE =4.2). In addition, the RBE of high-LET carbon beam was 2.8, as compared to 2.1 of the lower-LET carbon beam. This finding is consistent with those reported by other studies using lower-LET (46–80 keV/µm) carbon beams, and indicates that low-LET CIRT has less effectiveness than high-LET. The results of several previously published studies suggested that the RBEs of CIRT (LET =33.6–80 keV/µm) in photon-resistant cancer cells were relatively higher than those in photon-sensitive cancer cells (18-25). This appears to be caused by the relative photon resistance of the treated cell line.
Although the difference is not large, interestingly the Dq for proton (2.046) was even higher than for X-ray (1.681) in CNE-2R cells, suggesting that the sparing of tumor cells would be higher for proton than X-rays for the resistant cells. However, neither D10 (8.167 vs. 8.308) nor D37 (4.575 vs. 4.802) are significantly different between X-rays and proton for these cells. This suggests that proton may not be a good choice in treating recurrent and/or resistant tumors.
Taken together, results of the present and earlier studies suggest that lower LET (33.6–80 keV/µm) carbon ions had superior potential to kill photon-resistant cancer cells compared with photon-sensitive cancer cells. But due to the higher LET (184 or 315.7 keV/µm), the RBEs of photon-radiosensitive cancer cells are more efficiently increased as the LET increases, whereas the RBE of photon-radioresistant cancer cells is much less dependent on LET. Many in vitro studies over the years have shown a bell-shaped dependence of RBE for cell killing on LET, wherein RBE increases with LET to a maximum at about 30–150 keV/µm, then decreases at higher LET (16,46) and the range of LET most effective in cell-killing effect is 100–150 keV/µm (16,52). Further studies are required to address the question of how RBEs varies with LET (especially more than 150 keV/µm) in both radiosensitive and relatively radio-resistant cell lines to better illustrate and quantify this relationship to optimize therapeutic design using a choice of the nature and LET values of irradiation systems.
Conclusions
Carbon ion beam radiation demonstrated a clear advantage in cell killing over photon and proton beams for NPC cells. This is exhibited by a higher cell-killing effect with higher LET doses on both native CNE-2 cells and photon-resistant CNE-2R cells. The observed higher RBE values for both photon-sensitive and resistant NPC cells reveal a potential of CIRT in the management of both primary and locally recurrent NPC.
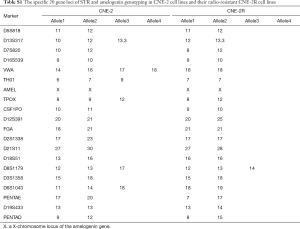
Full table
Acknowledgments
We thank BS Dwarakanath (Division of Research and Development, Shanghai Proton and Heavy Ion Center, Shanghai 201321, China) for helpful suggestions and editing the manuscript.
Funding: This work was financially supported by: The National Key Research and Development Program of China (2017YFC0108603); Natural Science Foundation of Shanghai (Project No. 15ZR1438600); Natural Science Foundation of Shanghai (Project No. 14ZR1407100); Natural Science Foundation of China (Project No. NSFC 21501029); Shanghai Hospital Development Center Joint Breakthrough Project for New Frontier Technologies (Project No. SHDC 12015118).
Footnote
Conflicts of Interest: All authors have completed the ICMJE uniform disclosure form (available at http://dx.doi.org/10.21037/tcr.2018.01.25). The authors have no conflicts of interest to declare.
Ethical Statement: The authors are accountable for all aspects of the work in ensuring that questions related to the accuracy or integrity of any part of the work are appropriately investigated and resolved. The study was conducted in accordance with the Declaration of Helsinki (as revised in 2013). Institutional ethical approval and informed consent were waived.
Open Access Statement: This is an Open Access article distributed in accordance with the Creative Commons Attribution-NonCommercial-NoDerivs 4.0 International License (CC BY-NC-ND 4.0), which permits the non-commercial replication and distribution of the article with the strict proviso that no changes or edits are made and the original work is properly cited (including links to both the formal publication through the relevant DOI and the license). See: https://creativecommons.org/licenses/by-nc-nd/4.0/.
References
- Kong L, Lu JJ. Reirradiation of locally recurrent nasopharyngeal cancer: history, advances, and promises for the future. Chin Clin Oncol 2016;5:26. [Crossref] [PubMed]
- Chua ML, Wee JT, Hui EP, et al. Nasopharyngeal carcinoma. Lancet 2016;387:1012-24. [Crossref] [PubMed]
- Leung TW, Tung SY, Sze WK, et al. Salvage brachytherapy for patients with locally persistent nasopharyngeal carcinoma. Int J Radiat Oncol Biol Phys 2000;47:405-12. [Crossref] [PubMed]
- Chua DT, Wei WI, Sham JS, et al. Stereotactic radiosurgery versus gold grain implantation in salvaging local failures of nasopharyngeal carcinoma. Int J Radiat Oncol Biol Phys 2007;69:469-74. [Crossref] [PubMed]
- Wei WI, Chan JY, Ng RW, et al. Surgical salvage of persistent or recurrent nasopharyngeal carcinoma with maxillary swing approach - Critical appraisal after 2 decades. Head Neck 2011;33:969-75. [Crossref] [PubMed]
- Kong L, Wang L, Shen C, et al. Salvage Intensity-Modulated Radiation Therapy (IMRT) for Locally Recurrent Nasopharyngeal Cancer after Definitive IMRT: A Novel Scenario of the Modern Era. Sci Rep 2016;6:32883. [Crossref] [PubMed]
- Han F, Zhao C, Huang SM, et al. Long-term outcomes and prognostic factors of re-irradiation for locally recurrent nasopharyngeal carcinoma using intensity-modulated radiotherapy. Clin Oncol (R Coll Radiol) 2012;24:569-76. [Crossref] [PubMed]
- Hua YJ, Han F, Lu LX, et al. Long-term treatment outcome of recurrent nasopharyngeal carcinoma treated with salvage intensity modulated radiotherapy. Eur J Cancer 2012;48:3422-8. [Crossref] [PubMed]
- Qiu S, Lin S, Tham IW, et al. Intensity-modulated radiation therapy in the salvage of locally recurrent nasopharyngeal carcinoma. Int J Radiat Oncol Biol Phys 2012;83:676-83. [Crossref] [PubMed]
- Weichselbaum RR, Beckett MA, Schwartz JL, et al. Radioresistant tumor cells are present in head and neck carcinomas that recur after radiotherapy. Int J Radiat Oncol Biol Phys 1988;15:575-9. [Crossref] [PubMed]
- Schulte RW, Wroe AJ. New developments in treatment planning and verification of particle beam therapy. Transl Cancer Res 2012;1:184-95. [PubMed]
- Kamada T, Tsujii H, Blakely EA, et al. Carbon ion radiotherapy in Japan: an assessment of 20 years of clinical experience. Lancet Oncol 2015;16:e93-e100. [Crossref] [PubMed]
- Holliday EB, Frank SJ. Proton therapy for nasopharyngeal carcinoma. Chin Clin Oncol 2016;5:25. [Crossref] [PubMed]
- Loeffler JS, Durante M. Charged particle therapy--optimization, challenges and future directions. Nat Rev Clin Oncol 2013;10:411-24. [Crossref] [PubMed]
- Halperin EC. Particle therapy and treatment of cancer. Lancet Oncol 2006;7:676-85. [Crossref] [PubMed]
- Held KD, Kawamura H, Kaminuma T, et al. Effects of Charged Particles on Human Tumor Cells. Front Oncol 2016;6:23. [Crossref] [PubMed]
- Huang YW, Pan CY, Hsiao YY, et al. Monte Carlo simulations of the relative biological effectiveness for DNA double strand breaks from 300 MeV u(-1) carbon-ion beams. Phys Med Biol 2015;60:5995-6012. [Crossref] [PubMed]
- Maalouf M, Alphonse G, Colliaux A, et al. Different mechanisms of cell death in radiosensitive and radioresistant p53 mutated head and neck squamous cell carcinoma cell lines exposed to carbon ions and x-rays. Int J Radiat Oncol Biol Phys 2009;74:200-9. [Crossref] [PubMed]
- Cui X, Oonishi K, Tsujii H, et al. Effects of carbon ion beam on putative colon cancer stem cells and its comparison with X-rays. Cancer Res 2011;71:3676-87. [Crossref] [PubMed]
- Oonishi K, Cui X, Hirakawa H, et al. Different effects of carbon ion beams and X-rays on clonogenic survival and DNA repair in human pancreatic cancer stem-like cells. Radiother Oncol 2012;105:258-65. [Crossref] [PubMed]
- Hirayama R, Uzawa A, Takase N, et al. Evaluation of SCCVII tumor cell survival in clamped and non-clamped solid tumors exposed to carbon-ion beams in comparison to X-rays. Mutat Res 2013;756:146-51. [Crossref] [PubMed]
- Amornwichet N, Oike T, Shibata A, et al. Carbon-ion beam irradiation kills X-ray-resistant p53-null cancer cells by inducing mitotic catastrophe. PLoS One 2014;9:e115121 [Crossref] [PubMed]
- Takahashi M, Hirakawa H, Yajima H, et al. Carbon ion beam is more effective to induce cell death in sphere-type A172 human glioblastoma cells compared with X-rays. Int J Radiat Biol 2014;90:1125-32. [Crossref] [PubMed]
- Amornwichet N, Oike T, Shibata A, et al. The EGFR mutation status affects the relative biological effectiveness of carbon-ion beams in non-small cell lung carcinoma cells. Sci Rep 2015;5:11305. [Crossref] [PubMed]
- Sai S, Vares G, Kim EH, et al. Carbon ion beam combined with cisplatin effectively disrupts triple negative breast cancer stem-like cells in vitro. Mol Cancer 2015;14:166. [Crossref] [PubMed]
- Durante M, Reppingen N, Held KD. Immunologically augmented cancer treatment using modern radiotherapy. Trends Mol Med 2013;19:565-82. [Crossref] [PubMed]
- Takahashi Y, Teshima T, Kawaguchi N, et al. Heavy ion irradiation inhibits in vitro angiogenesis even at sublethal dose. Cancer Res 2003;63:4253-7. [PubMed]
- Ogata T, Teshima T, Kagawa K, et al. Particle irradiation suppresses metastatic potential of cancer cells. Cancer Res 2005;65:113-20. [PubMed]
- Jensen AD, Poulakis M, Nikoghosyan AV, et al. Re-irradiation of adenoid cystic carcinoma: analysis and evaluation of outcome in 52 consecutive patients treated with raster-scanned carbon ion therapy. Radiother Oncol 2015;114:182-8. [Crossref] [PubMed]
- Combs SE, Kalbe A, Nikoghosyan A, et al. Carbon ion radiotherapy performed as re-irradiation using active beam delivery in patients with tumors of the brain, skull base and sacral region. Radiother Oncol 2011;98:63-7. [Crossref] [PubMed]
- Eekers DB, Roelofs E, Jelen U, et al. Benefit of particle therapy in re-irradiation of head and neck patients. Results of a multicentric in silico ROCOCO trial. Radiother Oncol 2016;121:387-94. [Crossref] [PubMed]
- Brown AP, Urie MM, Chisin R, et al. Proton therapy for carcinoma of the nasopharynx: a study in comparative treatment planning. Int J Radiat Oncol Biol Phys 1989;16:1607-14. [Crossref] [PubMed]
- Feehan PE, Castro JR, Phillips TL, et al. Recurrent locally advanced nasopharyngeal carcinoma treated with heavy charged particle irradiation. Int J Radiat Oncol Biol Phys 1992;23:881-4. [Crossref] [PubMed]
- Lin R, Slater JD, Yonemoto LT, et al. Nasopharyngeal carcinoma: repeat treatment with conformal proton therapy--dose-volume histogram analysis. Radiology 1999;213:489-94. [Crossref] [PubMed]
- Kong L, Gao J, Hu J, et al. Phase I/II trial evaluating concurrent carbon-ion radiotherapy plus chemotherapy for salvage treatment of locally recurrent nasopharyngeal carcinoma. Chin J Cancer 2016;35:101. [Crossref] [PubMed]
- Hu J, Bao C, Gao J, et al. Salvage Treatment Using Carbon-Ion Radiation in Patients with Locoregionally Recurrent Nasopharyngeal Carcinoma: The Initial Results. Cancer 2018; [Epub ahead of print].
- Li G, Liu Y, Su Z, et al. MicroRNA-324-3p regulates nasopharyngeal carcinoma radioresistance by directly targeting WNT2B. Eur J Cancer 2013;49:2596-607. [Crossref] [PubMed]
- Zhao L, Tang M, Hu Z, et al. miR-504 mediated down-regulation of nuclear respiratory factor 1 leads to radio-resistance in nasopharyngeal carcinoma. Oncotarget 2015;6:15995-6018. [PubMed]
- Kavanagh JN, Currell FJ, Timson DJ, et al. Antiproton induced DNA damage: proton like in flight, carbon-ion like near rest. Sci Rep 2013;3:1770. [Crossref] [PubMed]
- Paganetti H. Relative biological effectiveness (RBE) values for proton beam therapy. Variations as a function of biological endpoint, dose, and linear energy transfer. Phys Med Biol 2014;59:R419-72. [Crossref] [PubMed]
- Friedrich T, Scholz U, Elsasser T, et al. Systematic analysis of RBE and related quantities using a database of cell survival experiments with ion beam irradiation. J Radiat Res 2013;54:494-514. [Crossref] [PubMed]
- Tinganelli W, Ma NY, Von Neubeck C, et al. Influence of acute hypoxia and radiation quality on cell survival. J Radiat Res 2013;54:i23-30. [Crossref] [PubMed]
- Franken NA, Oei AL, Kok HP, et al. Cell survival and radiosensitisation: modulation of the linear and quadratic parameters of the LQ model Int J Oncol 2013;42:1501-15. (Review). [Crossref] [PubMed]
- Unkel S, Belka C, Lauber K. On the analysis of clonogenic survival data: Statistical alternatives to the linear-quadratic model. Radiat Oncol 2016;11:11. [Crossref] [PubMed]
- Zhang Y, Hu K, Beumer JH, et al. RAD-ADAPT: Software for modelling clonogenic assay data in radiation biology. DNA Repair (Amst) 2017;52:24-30. [Crossref] [PubMed]
- Hall EJ, Giaccia AJ. Radiobiology for the radiologist. Philadelphia: Lippincott Williams & Wilkins, 2012.
- Kong L, Hu J, Guan X, et al. Phase I/II Trial Evaluating Carbon Ion Radiotherapy for Salvaging Treatment of Locally Recurrent Nasopharyngeal Carcinoma. J Cancer 2016;7:774-83. [Crossref] [PubMed]
- Lewis GD, Holliday EB, Kocak-Uzel E, et al. Intensity-modulated proton therapy for nasopharyngeal carcinoma: Decreased radiation dose to normal structures and encouraging clinical outcomes. Head Neck 2016;38:E1886-95. [Crossref] [PubMed]
- Fujisawa H, Genik PC, Kitamura H, et al. Comparison of human chordoma cell-kill for 290 MeV/n carbon ions versus 70 MeV protons in vitro. Radiat Oncol 2013;8:91. [Crossref] [PubMed]
- Chhabra A, Langen K, Mehta MP. An overview of modern proton therapy. Chin Clin Oncol 2016;5:48. [Crossref] [PubMed]
- Giovannini G, Bohlen T, Cabal G, et al. Variable RBE in proton therapy: comparison of different model predictions and their influence on clinical-like scenarios. Radiat Oncol 2016;11:68. [Crossref] [PubMed]
- Suzuki M, Kase Y, Yamaguchi H, et al. Relative biological effectiveness for cell-killing effect on various human cell lines irradiated with heavy-ion medical accelerator in Chiba (HIMAC) carbon-ion beams. Int J Radiat Oncol Biol Phys 2000;48:241-50. [Crossref] [PubMed]