Dihydropyrimidine dehydrogenase overexpression correlates with potential resistance to 5-fluorouracil-based treatment in head and neck squamous cell carcinoma
Introduction
Over the past several decades, the 5-year survival for head and neck squamous cell carcinoma (HNSCC) patients has not improved despite advances in treatment (1). One of the reasons for this is treatment failure due to resistance to chemotherapy and/or radiotherapy (2). 5-Fluorouracil (5-FU) has been widely used for HNSCC patients either in chemotherapy alone or in combination with radiotherapy, as several studies have shown that 5-FU-based chemotherapy or chemoradiotherapy improved survival in HNSCC patients (3,4). However, patients with advanced or recurrent HNSCC frequently showed chemotherapeutic resistance to 5-FU, resulting in poor outcomes (5,6). Thus, identifying biomarkers of chemotherapeutic resistance to 5-FU is crucial to improve survival among HNSCC patients. To date, a variety of candidates have been reported as predictive markers for 5-FU response (7,8), including the tumor expression level of dihydropyrimidine dehydrogenase (DPD), an initial and rate-limiting enzyme for degrading 5-FU, in colorectal (9,10), gastric (11-14), and breast cancer (15). However, the relationship between DPD expression levels and chemotherapeutic resistance to 5-FU remains unclear in HNSCC. We therefore hypothesized that DPD overexpression increases 5-FU resistance in HNSCC cells and that overexpression of DPD in cancer cells predicts shorter overall survival of HNSCC patients who are treated with 5-FU-based chemotherapy in this study. The purpose of the present study is to evaluate the significance of DPD expression on 5-FU sensitivity and survival of HNSCC patients who receive 5-FU based treatment.
Methods
Patients
This study was conducted between October 2009 and December 2012 at Department of Otorhinolaryngology, Head and Neck Surgery, Yokohama City University, School of Medicine, Yokohama, Japan. The protocol was reviewed and approved by the institutional review boards of our institution (Number of ethical approval; B120112024). Of the 22 HNSCC patients who underwent concurrent chemoradiotherapy as a prior therapy, three cases who did not receive 5-FU-based chemotherapy were excluded. Finally, 19 patients were enrolled in this study. Radiotherapy was delivered 5 days a week using a single daily fraction of 1.8 or 2.0 Gray (Gy) for all patients. The median radiation dose was 70.2 Gy (range, 60.0–70.2 Gy).
Evaluation of mRNA expression in biopsy specimens
Tumor specimens were obtained prior to treatment initiation. Sections (10 µm in thickness) of the areas with the highest tumor concentration were obtained from formalin-fixed paraffin-embedded (FFPE) tumor specimens, and then mounted on uncoated glass slides. Representative sections were stained with haematoxylin and eosin by the standard method. The regions of interest were selectively isolated by laser capture microdissection (P.A.L.M. Microsystem, Leica, Wetzlar, Germany), according to the standard procedure (16). RNA isolation and cDNA synthesis were conducted as described previously (14). Quantification of DPD and an internal reference gene (β-actin) was performed using a fluorescence-based real-time detection method (ABI PRISM 7900 Sequence detection System, TaqMan®, Perkin-Elmer (PE) Applied Biosystem, Foster City, CA) as described previously (17). Patients were divided into the high- and low-level DPD mRNA expression groups according to a cut-off value defined using the receiver operating characteristic (ROC) curve.
Cell lines
The human HNSCC cell line, HSC-3, was purchased from American Type Culture Collections (Rockville, MD). The cells were cultured in RPMI-1640 medium containing 10% fetal bovine serum (FBS), 100 µg/mL of streptomycin, and 100 units of penicillin at 37 °C with 5% CO2. To establish a 5-FU-resistant cell line, HSC-3 cells were treated continuously with step-wise concentrations of 5-FU every 2 weeks up to 40 µmol/L, based on a previous report (13). The resultant 5-FU-resistant cell line is hereafter referred to as HSC-3R cells.
Clonogenic survival assay
To determine the sensitivity of HSC-3 cells and HSC-3R cells to 5-FU, clonogenic survival assay was performed as described previously (18). After plating in 6-well plates in triplicate at equal density and subsequent incubation for 24 hours, the cells were exposed to various concentrations (0–400 µmol/L) of 5-FU (Kyowa Hakko Kirin Co., Tokyo, Japan). After 48 hours, medium contained 5-FU was removed and replaced fresh medium without 5-FU. After 11 to 14 days of incubation, the cells were fixed and stained with 0.5% crystal violet solubilized in absolute ethanol, and colonies with more than 50 cells were counted under a dissection microscope. Plating efficacy was defined as the percentage of seeded cells in a given cell line that grew into colonies under a specific culture condition. The concentrations of 5-FU necessary to inhibit growth by 50% (50% maximal effective concentration: IC50) and 15% (15% maximal effective concentration: IC15) were calculated. Three independent experiments were carried out for each treatment.
Water soluble tetrazolium salts-8; 2-(2-methoxy-4-nitrophenyl)-3-(4-nitrophenyl)-5-(2,4-disulfophenyl)-2H-tetrazolium, monosodium salt (WST-8) assay
To examine the effect of 5-Chloro-2,4-dihydroxypyridine (CDHP; Wako Pure Chemical Industries, Osaka, Japan), which acts as a 5-FU modulator to competitively inhibit DPD in vitro, on 5-FU sensitivity, 1×103 of HSC-3 cells or 2×103 of HSC-3R cells were seeded on 96-well microplates and further incubated for 24 h. Both cell lines were exposed to 5-FU, fixed at the respective IC15, in combination with various concentrations of CDHP (0.1–100 µmol/L) for another 72 hours as described previously (19). The cytotoxic effect of the drugs was evaluated by Cell Counting Kit-8 (CCK-8, Dojindo Laboratories, Kumamoto, Japan) according to the instructions. Three independent experiments were carried out for each treatment.
Quantitative RT-PCR
RNA extraction from both cell lines and cDNA synthesis were conducted as described previously (20,21). Real-time PCR was performed on an Applied Biosystems Step One Plus real-time PCR system (Applied Biosystems, CA, USA) using TaqMan® gene expression assays with the following ID numbers (DPD, Hs00559279_m1; β-actin, Hs99999903_m1).
Western blotting analysis
Western blotting analysis was performed to detect DPD expression in both cell lines as described previously (22). Antibodies were purchased from the following sources and used at the indicated dilutions: DPD (1:5,000; Cell Signaling Technologies, Danvers, MA) and β-actin (1:1,000; Cell Signaling Technologies).
Statistical analysis
Chi-square tests were used to examine correlations between DPD mRNA expression and patient characteristics. The overall survival (OS) was defined as the time from the date of diagnosis to the date of death from any cause. The cancer-specific survival (CSS) was defined as the time from the date of diagnosis to the date of death from the cancer in the absence of other causes of death. OS and CSS were analyzed using the Kaplan-Meier method with the Wilcoxon log-rank test. The ROC curve was calculated with EZR (Saitama Medical Center, Jichi Medical University) (23) to assess the optimal cut-off value for mRNA expression, defined as the point with the highest sensitivity and specificity for CSS. Statistical analyses were performed using Graph Pad Prism Version 6.05 (Graph Pad Software, La Jolla CA). For all comparisons, differences with P<0.05 were considered statistically significant.
Results
Correlation between DPD expression and clinical outcome
HNSCC patients who had undergone concurrent chemoradiotherapy with 5-FU-based regimens were enrolled, and we evaluated whether DPD expression was correlated with their survival prognosis. Patient characteristics were summarized in Table 1. Of the 19 patients, 16 were male, and the median age of patients was 70 years (range, 44–81 years). Thirteen patients were received 5-FU in combination with cisplatin or carboplatin, 2 patients received S-1 (an oral fluoropyrimidine preparation combining tegafur, oteracil potassium, and CDHP), and 4 patients received Uracil-tegafur (UFT) as a single agent or in combination with carboplatin. There was no patient with toxic death related to radio-chemotherapy in this study. The patient information including used treatment regimens was summarized in Table 2. The cut-off value for DPD mRNA expression calculated from the ROC curve was 8.53 with an area under the curve of 0.646 against CSS. Kaplan-Meier analysis showed statistically significant shorter OS and CSS for patients with DPD expression above the cut-off value as shown in Figure 1 (P=0.0018 and 0.0004, respectively). These results suggested that high-level DPD expression was correlated with shorter OS and CSS in HNSCC patients receiving 5-FU-based chemoradiotherapy.
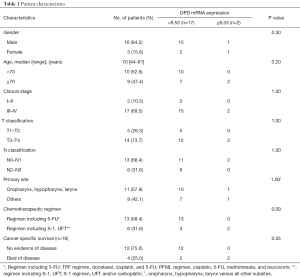
Full table
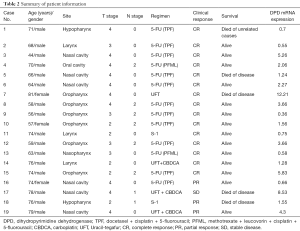
Full table
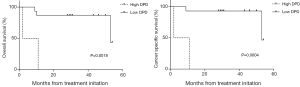
Establishment of 5-FU-resistant HNSCC HSC-3R cells
In order to explore the association between DPD expression and 5-FU resistance, we next developed a 5-FU-resistant HNSCC cell line, HSC-3R. HSC-3R cells could grow in the presence of 5-FU concentrations up to 40 µmol/L, although HSC-3 cells could not be grown under the same conditions (data not shown). As shown in Figure 2, the concentration of 5-FU necessary to inhibit cell proliferation by 50% (IC50) in HSC-3R was 28.2 µmol/L, which was higher than that of the parental HSC-3 cells (2.23 µmol/L). HSC-3R cells, therefore, showed 12.64-fold greater resistance to 5-FU compared with the parental HSC-3 cells.
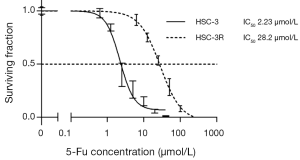
High DPD mRNA/protein expression in HSC-3R cells
We next evaluated DPD expression in HSC-3R cells. DPD mRNA expression levels in HSC-3 and HSC-3R cells were quantified using a real-time RT-PCR technique. As shown in Figure 3A, the DPD mRNA expression level in HSC-3R cells increased significantly in comparison with that in the HSC-3 cells (P=0.0005). DPD protein expression levels in both cells were then evaluated by Western blotting analysis. The DPD protein expression level was also greater in the HSC-3R cells than that in the HSC-3 cells (Figure 3B). These results suggested that the increase in DPD expression in HSC-3R cells might be associated with 5-FU resistance.
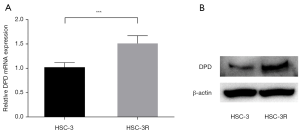
Inhibition of DPD attenuates 5-FU resistance in HSC-3R cells
Furthermore, we examined the effects of CDHP on 5-FU cytotoxicity in the HSC-3 and HSC-3R cells to examine if inhibition of DPD can restore the sensitivity of HNSCC cells to 5-FU. CDHP alone had no effect on cell growth in either cell line (data not shown). Figure 4 shows the sensitivity of both cell lines to 5-FU in combination with CDHP administration. Both the HSC-3 and HSC-3R cells were exposed to 5-FU at their respective IC15 concentrations in combination with CDHP at concentrations from 0.1–100 µmol/L. The IC15 concentration of 5-FU in HSC-3 cells was 2 µmol/L, and that in HSC-3R cells was 20 µmol/L. As shown in Figure 4B, the results of WST-8 analysis revealed that the combination of 5-FU with CDHP had a significant inhibitory effect on 5-FU cytotoxicity in HSC-3R cells in 1–100 µmol/L concentration of CDHP exposure. In contrast, the addition of any concentration of CDHP did not alter the cytotoxicity of 5-FU in HSC-3 cells (Figure 4A). These results suggested that inhibition of DPD using CDHP attenuates 5-FU resistance in HSC-3R cells.
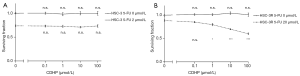
Discussion
In this study, we demonstrated that the high-level DPD expression group showed significantly shorter OS and CSS than the low-level DPD expression group among HNSCC patients who had undergone concurrent chemoradiotherapy with 5-FU-based regimens. Furthermore, we demonstrated that HSC-3R cells, established as 5-FU-resistant HNSCC cells, showed higher DPD overexpression than did the parent cells in terms of both mRNA and protein levels. HSC-3R cells attenuated 5-FU resistance induced by the combined use of a DPD inhibitor, CDHP. These results suggested that a high level of DPD expression was correlated with 5-FU resistance and that DPD expression level might be a predictive biomarker in 5-FU-based chemoradiotherapy for HNSCC patients.
It is well known that 5-FU exerts its antitumor effects through the inhibition of thymidylate synthase and the incorporation of its metabolites into RNA and DNA via a complicated mechanism that involves several enzymes (24). On the other hand, more than 80% of the administered 5-FU is rapidly metabolized to an inactive form by DPD, the rate-limiting enzyme of 5-FU catabolism. Thus, DPD have been assumed to be one of the key enzymes associated with 5-FU resistance. In fact, several studies have reported that DPD overexpression induced 5-FU resistance in various cancers (25-27). In this study, we determined that HSC-3R cells, established by continuous exposure to 5-FU as described previously (28,29), increased DPD expression in a 5-FU dose-dependent manner (data not shown), which is consistent with the results of previous reports (19,30). We also determined that HSC-3R cells decreased 5-FU resistance significantly in combination with CDHP, similar to the finding of the previous studies. In fact, Kikuchi et al. have reported that the 5-FU-resistant esophageal SCC cells established in their study showed high DPD expression and attenuated 5-FU resistance induced by a DPD inhibitor (31). DPD expression could, therefore, be related to 5-FU resistance through the acceleration of 5-FU metabolism. Recent studies have suggested that DPD expression is correlated with not only chemoresistance but also radioresistance. Takagi et al. reported that CDHP had a radiosensitizing effect through the suppression of DNA double-strand repair after disruption by irradiation (32). Sakata et al. also found that DPD depletion enhanced the efficacy of radiotherapy in inhibiting DNA repair (33). High levels of DPD expression could thus contribute to potential radioresistance and lead to shorter overall survival. Further study is necessary to investigate the relationship between DPD expression and radioresistance in HNSCC.
While this study comprised only a small number of HNSCC patients in a single institution, survival analysis showed a significantly shorter OS and CSS in patients with a high level of DPD expression. In addition, our Chi-square analysis based on age, gender, clinical stage, T classification, N classification, primary site, chemotherapeutic regimen and DPD mRNA expression showed that high levels of DPD mRNA expression had a tendency to be associated with worse CSS (P=0.050; Table 1). Other limitations might exist in that miscellaneous chemotherapy regimens were used in this study. Therefore, there is possibility that the impact of DPD expression on the efficacy of the chemotherapy might be different according to the co-administration of a DPD inhibitor. To examine the impact of DPD expression on fluoropyrimidine efficacy for patients treated with tegafur in combination with DPD inhibitors, we have performed an additional subgroup analysis with 6 patients treated with S-1 or UFT. The result also showed that the high-level DPD expression group showed shorter CSS compared to the low-level DPD expression group, however, the difference was not significant (data not shown). Despite these limitations, to the best of our knowledge, this is the first report on the evaluation of DPD expression in HNSCC patients administrated 5-FU-based chemoradiotherapy. Further multi-institutional study studies with a larger cohort and unified regimens should be required to confirm our findings and clarify the value of DPD expression as a predictive biomarker.
Conclusions
In conclusion, we clarified that the high-level DPD expression group of HNSCC patients undergoing concurrent chemoradiotherapy with 5-FU-based regimens showed significantly shorter OS and CSS. The 5-FU-resistant cells established from HNSCC cells showed increased DPD expression and attenuated 5-FU resistance induced by CDHP. Our results suggested that DPD expression could play an important role in 5-FU resistance and that DPD expression level might be a predictive biomarker in 5-FU-based chemoradiotherapy for patients with HNSCC.
Acknowledgments
We thank Mari Mitsuka (Department of Biology and Function in Head and Neck, Yokohama City University Graduate School of Medicine, Yokohama, Japan) and Hideaki Mitsui (Department of Pathology) for their excellent technical assistance.
Funding: This work was supported by Taiho Pharmaceutical Co., Ltd. (Tokyo, Japan).
Footnote
Conflicts of Interest: All authors have completed the ICMJE uniform disclosure form (available at http://dx.doi.org/10.21037/tcr.2018.03.38). The authors have no conflicts of interest to declare.
Ethical Statement: The authors are accountable for all aspects of the work in ensuring that questions related to the accuracy or integrity of any part of the work are appropriately investigated and resolved. The study was conducted in accordance with the Declaration of Helsinki (as revised in 2013). The protocol was reviewed and approved by the institutional review boards of our institution (Number of ethical approval; B120112024). A written, informed consent was obtained for all participants of the study.
Open Access Statement: This is an Open Access article distributed in accordance with the Creative Commons Attribution-NonCommercial-NoDerivs 4.0 International License (CC BY-NC-ND 4.0), which permits the non-commercial replication and distribution of the article with the strict proviso that no changes or edits are made and the original work is properly cited (including links to both the formal publication through the relevant DOI and the license). See: https://creativecommons.org/licenses/by-nc-nd/4.0/.
References
- Goldberg HI, Lockwood SA, Wyatt SW, et al. Trends and differentials in mortality from cancers of the oral cavity and pharynx in the United States, 1973-1987. Cancer 1994;74:565-72. [Crossref] [PubMed]
- Boyer J, Maxwell PJ, Longley DB, et al. 5-Fluorouracil: identification of novel downstream mediators of tumour response. Anticancer Res 2004;24:417-23. [PubMed]
- Adelstein DJ, Saxton JP, Rybicki LA, et al. Multiagent concurrent chemoradiotherapy for locoregionally advanced squamous cell head and neck cancer: mature results from a single institution. J Clin Oncol 2006;24:1064-71. [Crossref] [PubMed]
- Pignon JP, Bourhis J, Domenge C, et al. Chemotherapy added to locoregional treatment for head and neck squamous-cell carcinoma: three meta-analyses of updated individual data. MACH-NC Collaborative Group. Meta-Analysis of Chemotherapy on Head and Neck Cancer. Lancet 2000;355:949-55. [Crossref] [PubMed]
- Colevas AD. Chemotherapy options for patients with metastatic or recurrent squamous cell carcinoma of the head and neck. J Clin Oncol 2006;24:2644-52. [Crossref] [PubMed]
- Gibson MK, Li Y, Murphy B, et al. Randomized phase III evaluation of cisplatin plus fluorouracil versus cisplatin plus paclitaxel in advanced head and neck cancer (E1395): an intergroup trial of the Eastern Cooperative Oncology Group. J Clin Oncol 2005;23:3562-7. [Crossref] [PubMed]
- Johnston PG, Drake JC, Trepel J, et al. Immunological quantitation of thymidylate synthase using the monoclonal antibody TS 106 in 5-fluorouracil-sensitive and -resistant human cancer cell lines. Cancer Res 1992;52:4306-12. [PubMed]
- Copur S, Aiba K, Drake JC, et al. Thymidylate synthase gene amplification in human colon cancer cell lines resistant to 5-fluorouracil. Biochem Pharmacol 1995;49:1419-26. [Crossref] [PubMed]
- McLeod HL, Sludden J, Murray GI, et al. Characterization of dihydropyrimidine dehydrogenase in human colorectal tumours. Br J Cancer 1998;77:461-5. [Crossref] [PubMed]
- Goto T, Shinmura K, Yokomizo K, et al. Expression levels of thymidylate synthase, dihydropyrimidine dehydrogenase, and thymidine phosphorylase in patients with colorectal cancer. Anticancer Res 2012;32:1757-62. [PubMed]
- Ishikawa Y, Kubota T, Otani Y, et al. Dihydropyrimidine dehydrogenase and messenger RNA levels in gastric cancer: possible predictor for sensitivity to 5-fluorouracil. Jpn J Cancer Res 2000;91:105-12. [Crossref] [PubMed]
- Kirihara Y, Yamamoto W, Toge T, et al. Dihydropyrimidine dehydrogenase, multidrug resistance-associated protein, and thymidylate synthase gene expression levels can predict 5-fluorouracil resistance in human gastrointestinal cancer cells. Int J Oncol 1999;14:551-6. [PubMed]
- Chung YM, Park S, Park JK, et al. Establishment and characterization of 5-fluorouracil-resistant gastric cancer cells. Cancer Lett 2000;159:95-101. [Crossref] [PubMed]
- Matsubara J, Nishina T, Yamada Y, et al. Impacts of excision repair cross-complementing gene 1 (ERCC1), dihydropyrimidine dehydrogenase, and epidermal growth factor receptor on the outcomes of patients with advanced gastric cancer. Br J Cancer 2008;98:832-9. [Crossref] [PubMed]
- Horiguchi J, Takei H, Koibuchi Y, et al. Prognostic significance of dihydropyrimidine dehydrogenase expression in breast cancer. Br J Cancer 2002;86:222-5. [Crossref] [PubMed]
- Bonner RF, Emmert-Buck M, Cole K, et al. Laser capture microdissection: molecular analysis of tissue. Science 1997;278:1481,1483.
- Nakajima TE, Yamada Y, Shimoda T, et al. Combination of O6-methylguanine-DNA methyltransferase and thymidylate synthase for the prediction of fluoropyrimidine efficacy. Eur J Cancer 2008;44:400-7. [Crossref] [PubMed]
- Sano D, Matsumoto F, Valdecanas DR, et al. Vandetanib restores head and neck squamous cell carcinoma cells' sensitivity to cisplatin and radiation in vivo and in vitro. Clin Cancer Res 2011;17:1815-27. [Crossref] [PubMed]
- Sasaki E, Tominaga K, Kuwamura H, et al. Synergistic antitumor effect of combined 5-fluorouracil (5-FU) with 5-chloro-2,4-dihydroxypyridine on 5-FU-resistant gastric cancer cells: possible role of a dihydropyrimidine dehydrogenase-independent mechanism. J Gastroenterol 2007;42:816-22. [Crossref] [PubMed]
- Akutsu T, Kitayama T, Watanabe K, et al. Comparison of automated and manual purification of total RNA for mRNA-based identification of body fluids. Forensic Sci Int Genet 2015;14:11-7. [Crossref] [PubMed]
- Tanaka A, Nakagawa H, Tomita C, et al. BRASSINOSTEROID UPREGULATED1, encoding a helix-loop-helix protein, is a novel gene involved in brassinosteroid signaling and controls bending of the lamina joint in rice. Plant Physiol 2009;151:669-80. [Crossref] [PubMed]
- Hyakusoku H, Sano D, Takahashi H, et al. JunB promotes cell invasion, migration and distant metastasis of head and neck squamous cell carcinoma. J Exp Clin Cancer Res 2016;35:6. [Crossref] [PubMed]
- Kanda Y. Investigation of the freely available easy-to-use software 'EZR' for medical statistics. Bone Marrow Transplant 2013;48:452-8. [Crossref] [PubMed]
- Longley DB, Harkin DP, Johnston PG. 5-fluorouracil: mechanisms of action and clinical strategies. Nat Rev Cancer 2003;3:330-8. [Crossref] [PubMed]
- Tanaka K, Saigusa S, Toiyama Y, et al. TS and DPD mRNA levels on formalin-fixed paraffin-embedded specimens as predictors for distant recurrence of rectal cancer treated with preoperative chemoradiotherapy. J Surg Oncol 2012;105:529-34. [Crossref] [PubMed]
- Kobayashi H, Koike T, Nakatsuka A, et al. Dihydropyrimidine dehydrogenase expression predicts survival outcome and chemosensitivity to 5-fluorouracil in patients with oral squamous cell carcinoma. Oral Oncol 2005;41:38-47. [Crossref] [PubMed]
- Terashima M, Fujiwara H, Takagane A, et al. Prediction of sensitivity to fluoropyrimidines by metabolic and target enzyme activities in gastric cancer. Gastric Cancer 2003;6:71-81. [Crossref] [PubMed]
- Kang HC, Kim IJ, Park JH, et al. Identification of genes with differential expression in acquired drug-resistant gastric cancer cells using high-density oligonucleotide microarrays. Clin Cancer Res 2004;10:272-84. [Crossref] [PubMed]
- Yoo BC, Jeon E, Hong SH, et al. Metabotropic glutamate receptor 4-mediated 5-Fluorouracil resistance in a human colon cancer cell line. Clin Cancer Res 2004;10:4176-84. [Crossref] [PubMed]
- Qiu T, Xiang X, Lei W, et al. Establishment and biological characterization of 5-fluorouracil-resistant human colon cancer HT-29/5-FU cell line. Xi Bao Yu Fen Zi Mian Yi Xue Za Zhi 2015;31:328-32. [PubMed]
- Kikuchi O, Ohashi S, Nakai Y, et al. Novel 5-fluorouracil-resistant human esophageal squamous cell carcinoma cells with dihydropyrimidine dehydrogenase overexpression. Am J Cancer Res 2015;5:2431-40. [PubMed]
- Takagi M, Sakata K, Someya M, et al. Gimeracil sensitizes cells to radiation via inhibition of homologous recombination. Radiother Oncol 2010;96:259-66. [Crossref] [PubMed]
- Sakata K, Someya M, Matsumoto Y, et al. Gimeracil, an inhibitor of dihydropyrimidine dehydrogenase, inhibits the early step in homologous recombination. Cancer Sci 2011;102:1712-6. [Crossref] [PubMed]