Intraoperative radiotherapy (IORT)—a resurrected option for treating glioblastoma?
Background and rationale
Glioblastoma multiforme (GBM) represents the most aggressive and most lethal primary brain tumor. Despite multimodal therapies including tumor resection, radiochemotherapy and adjuvant chemotherapy, GBM is still associated with a median survival of roughly 14 months (1). Local recurrence is the major cause for clinical deterioration (and deaths) and is frequently observed within 2-3 cm from the initial lesion (2-4). Although recurrent GBM is basically not uniformly treated as there are no commonly established guidelines, patients frequently require second-line chemotherapies (e.g., with bevacizumab and/or irinotecan), re-irradiation or, if applicable, re-surgery. However, the survival time with an acceptable quality of life after almost any salvage therapy does not exceed 3-4 months (5-8).
It is known that the extent of resection is one of the most important prognostic factors in patients with GBM as patients with radiographic complete tumour resection have a significantly higher survival rate than patients with incompletely resected tumors (9,10). Early multivariate analyses revealed that at least 98% of the tumor have to be removed to provide survival benefits, which for several reasons (including technical limitations or involvement of eloquent areas) is possible in only roughly 20% of all patients with GBM (11). Thus, to achieve survival benefits in the largest cohort of patients (specifically those with operable, but incompletely resectable tumors), there is an urgent need for efficient therapy add-ons.
The main goal of such add-ons should be to achieve additional tumor cell depletion or at least a growth arrest of non-depleted cells in the period between surgery and adjuvant therapies. It is well known that the mean doubling time of a residual mass is roughly three weeks (12) and consequently, the waiting time for EBRT correlates with overall survival (13). Thus, any delay in starting adjuvant therapies results in significant re-growth of the tumor, making subsequent therapies more or less incapable of lowering the residual tumor burden below the “threshold” under which tumor control could be achieved by the immune system (Figure 1).
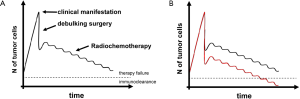
Three approaches to achieve cellular depletion (or growth arrest) after debulking surgery shall be mentioned in this regard: local chemotherapy, local dose-escalated external beam radiotherapy (EBRT) and intraoperative radiotherapy (IORT).
The first strategy is the application of local chemotherapy, which can be achieved with implantable wafers that slowly (within 3-6 weeks) decay and thereby release an alkylating agent (carmustine/BCNU) into the cavity (14). This elegant strategy allows high local levels of a cytostatic drug without the need for the substance to cross the blood-brain barrier and with (almost) no systemic effects (15). Wafers carried a “wave” of excitement when dramatically improved survival rates were reported from a large randomized study that included patients that underwent re-operation for recurrent glioma (16). However, as they only marginally increased survival in the primary setting and elevated complication rates (including infections, wound healing abnormalities, CSF leak) were reported, their actual role is to date controversially discussed (17,18).
The second strategy is to apply higher local radiation doses with EBRT, which has also been proven effective in several studies. One of earliest landmark studies was conducted by the Brain Tumor Study Group (BTSG) in 1979 (19). Within this study, more than 600 patients with high-grade gliomas were allocated to different doses of EBRT up to 60 Gy. It turned out that patients receiving less than 45 Gy had the worst median survival (4.5 months) and escalation of the dose lead to a significant increase in survival (7 months in the cohort receiving 50 Gy vs. 10.5 months in the cohort receiving 60 Gy, P=0.004). Similar effects were seen in the MRC trial in 1991, where 144 patients received 45 Gy and 299 patients 60 Gy. Here, dose escalation also lead to a statistically significant increase in median survival (9 vs. 12 months; P=0.007) (20). The question now is whether or not doses above the commonly applied 60 Gy will still increase survival. This question was, at least in part, since it was a non-randomized setting, answered by the RTOG 9803 trial. In this trial, patients treated with 66 Gy showed the worst (11.6 months) and those that were receiving 84 Gy showed the best survival rates (19.3 months) (21). Of note, ‘high-dose’ EBRT did not result in elevated rates of toxicities. Thus, in GBM there is an undeniable benefit from dose escalation up to 60 Gy and a very likely benefit from doses over 60 Gy (for example 84 Gy as applied in the RTOG trial).
The third approach is to spare healthy tissue during dose escalation by the use of loco-regional (or interstitial) radiotherapy methods including brachytherapy (BT) and IORT. While BT does not necessarily require to be applied during the debulking operation, “true” IORT takes place before cranioplasty directly after gross (or subtotal) tumor resection. IORT appears to be a simple and feasible principle but technical and geometrical obstacles have putatively lowered its potential in most published studies. Here, we will review past and present IORT strategies in the treatment of GBM and also give an overview on novel treatment options that may increase IORT efficiency. We will also provide an outlook on a trial which we have initiated aiming to find a maximum tolerable dose for a randomized phase III study.
The past
The idea of delivering a single shot of high-dose radiation to an intracerebral tumor cavity after (total or subtotal) resection is, by far, not new. In the early 1980s, in a time far before the era of neuronavigation, temozolomide, intensity-modulated radiotherapy, MRI-based diagnostics or PET-based treatment planning, a pioneering group of scientists including Mitsuyuki Abe (Kyoto), Tadayoshi Matsuda (Tokyo) and Alfred Goldson (Washington) was the first to report the application of electron beam-based IORT (IOERT) on patients with supratentorial gliomas (22-25), which was followed by the pilot studies of the group of Masao Matsutani (26,27). Since then, several additional report on small-sized, non-randomized institutional studies on IOERT were published, many of which revealed encouraging results (28-37) (Table 1).
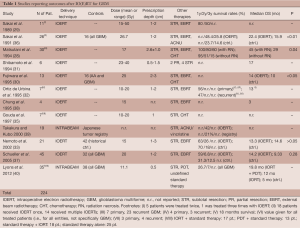
Full table
The most promising data was seen in the early Japanese trials: In their first paper, Sakai et al. presented results of a study on 11 patients with GBM that received 15-50 Gy IOERT prescribed to 1-2 cm beneath the tumor cavity (referred to as “depth”), followed by a median dose of 50 Gy of EBRT (29). They reported 1- and 2-year survival rates of 80% and 50% without increased rates of adverse effects—even not at the highest doses (six patients received IOERT multiple times). The second paper was published two years later and included 26 GBM-patients, whereas all patients also received adjuvant radiochemotherapy (with ACNU) and were followed for at least 12 months (36). In this study, it also turned out that patients showed to benefit from IOERT, which provided a significantly prolonged overall survival compared to a control group (22.4 vs. 15.9 months in the control group, P<0.01). Of note, 14 of these patients received IOERT multiple times (after recurrence) and, although follow-ups were based on low-sensitivity CT imaging, the group did not observe any serious complications (such as brain necrosis).
Similar mesmerizing results were reported by Matsutani and colleagues, who treated 30 GBM patients with IOERT (median dose 18.3 Gy, 20 MeV) and EBRT (mean dose 58.5 Gy). They reported a median overall survival of roughly 30 months and a 2-year survival rate of 61% (28). Interestingly, the median survival of patients with extensive postoperative (radio) necrosis was reported to be even significantly longer compared to patients with no necrosis. Fujiwara et al. conducted a study that included 20 patients (10 primary GBM, 1 recurrent GBM, 7 anaplastic and 2 low-grade astrocytomas), which were treated with IOERT at doses of 20 or 25 Gy (prescribed to a depth of 2-3 cm, energies: 6-10 MeV), followed by 50 Gy EBRT and chemotherapy (ACNU/cisplatin) (30). The median survival turned out to be significantly better with IOERT (14 vs. 10 months of a historical in-house control). There was no significant difference between the group receiving 20 Gy and those receiving 25 Gy; instead, the rate of adverse effects was increasing: 5 of 6 adverse events seen in the trial occurred in the group receiving 25 Gy (1 radionecrosis at 20 Gy and 1 convulsion, 1 abscess, 3 severe brain edemas at 25 Gy) (30).
The group of Felipe Calvo and his colleagues from Pamplona published the first European data on IOERT for malignant brain tumors. They applied IOERT (10-20 Gy, 6-20 MeV) in a broad variety of tumors, including several cases of primary and recurrent high grade gliomas. Especially the outcome in the recurrent setting was remarkable: nearly half (47%) of the patients treated lived 18 months or longer, the median time to progression and the median overall survival were 11 and 13 months, which was considered to be highly superior to any other salvage therapy available at this time (32,41,42).
The encouraging data from Japan and Spain could not be reproduced in the following years, neither by another Japanese (33) nor a German IOERT study conducted by the group of Norman Willich (37). Nemoto and colleagues included 21 patients with GBM and applied IOERT with doses of 12-15 Gy followed by EBRT with a median dose of 60 Gy. They found no significant difference in the survival rate between IOERT-treated patients and a historical control (33). The German study reported on outcomes of 45 patients with GBM that were treated with IOERT at doses of 15-25 Gy followed by 60 Gy of EBRT. Although both, the median and the 1-year survival rate were slightly in favor for IOERT there was no statistical difference detectable that would prove the efficiency of the procedure (37).
Of note, both study teams faced either logistical (the Japanese group transferred patients from the OR to the LINAC rooms) or technical (the positioning of the electron cones was not made on the basis of radiation planning) challenges which they suspected to contribute to non-superiority of combined IOERT/EBRT over EBRT only. Consequently, the German group established methods for postoperative dose reconstruction and recognized that a significant amount of included patients exhibited areas of inadequate target volume coverage (TVC). The predominant reasons were wrongly selected electron energies (mostly too low), inappropriate cone sizes (mostly undersized) and angle errors (Figure 2). As expected, they found that patients with adequate TVC showed a significantly improved median survival and 2-year survival rate as compared to patients with inadequate TVC (15.2 vs. 9.3 months and 9.3% vs. 0%, P=0.02) (37,43).
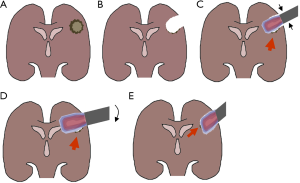
The present
Due to the multiple technical challenges mentioned and the failure to considerably improve survival rates, neurosurgeons were reluctant to use intraoperative radiation devices in the context of GBM thereafter. Together with the advent of radiosurgery for brain metastases, the putative lack of benefit in glioma therapy has not only prompted researchers but also companies that manufacture such devices to move their focus towards other indications. Both, electron- and kV-based IORT devices had a true resurgence in the treatment of breast cancer, where both IOERT and IORT are on the way to become an equivalent treatment option to EBRT in selected early stages (44-50).
In the TARGIT trials, IORT was applied using the INTRABEAM® system (Carl Zeiss Meditec AG, Germany), a device that was initially developed for interstitial radiosurgery of brain tumors before stereotactic (external) radiosurgery (or stereotactic ablative radiotherapy) entered the stage. In the setting of interstitial radiotherapy for brain metastasis (applied doses were 10-20 Gy in 2 mm depth), INTRABEAM achieved local control in up to 80% of cases with delayed necrosis appearing in less than 5% (51-53). These interstitial irradiations were performed with a needle-shaped applicator that was mounted on a stereotactic frame and inserted into the center of a mass without prior resection. In the breast cancer setting, IORT is delivered by the use of spherical plastic applicators, which are fitted into the tumor cavities in the conserved breast. Consequently, in contrast to the previously used forward-beaming electron cones, spherical irradiation sources are specifically attractive in brain tumor IORT, where post-resection cavities are normally of complex shape (Figure 3).
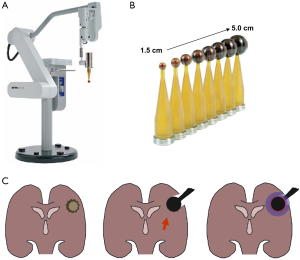
Logically, even at a time before the actual launch of the TARGIT trial, IORT using spherical applicators has been assessed in adult primary brain tumors: Takakura and Kubo intraoperatively irradiated 55 high-grade gliomas with the applicators and saw 2-year survival rates of 89% and 42% for patients with anaplastic astrocytoma or GBM, which was at that time superior to the control rates from the Japanese tumor registry (77% and 21%) (39).
The putatively most widely recognized paper reporting on outcomes after IORT for brain tumors with the INTRABEAM applicators was published by John Kalapurakal and his colleagues from Chicago’s Northwestern Memorial Hospital. The group treated 14 children (4-21 years of age) with recurrent primary brain tumors (mostly ependymomas, n=13) with 10 Gy in 2 and 5 mm depth or 12 Gy in 2 mm depth) using spherical applicators (54). With a median follow-up of six months (range, 5-40 months) the group had three cases of radiation necrosis and all were occurring in previously unirradiated patients that were treated at the higher dose level (10 Gy in 5 mm depth). Although none of these patients died and all remained asymptomatic after treatment (2 were treated conservatively, 1 required surgery), the group decided to stop the dose escalation and limit the dose to 10 Gy in 2 mm depth. As no other late toxicity appeared and local control was achieved in 8 out of 14 patients (57%) with the best response observed in previously unirradiated tumors (local control was achieved in 5 of 6 patients; 83%), IORTs with INTRABEAM applicators can be judged as feasible and safe procedures even in children, where brain tissue is most sensitive to irradiation (55,56).
The most recent data that reported on the use of spherical applicators in patients with GBM was contributed by a group of the University of Dundee, which assessed whether IORT could improve the effects of photodynamic therapy (PDT) (40). PDT makes use of photosensitizers that selectively enrich in GBM cells, allowing irradiating the tumors (or the remaining tumor tissue) with lasers, thereby exciting the sensitizer in a way that singlet oxygen is produced causing subsequent cellular damage. The group treated 73 patients with standard therapy (ST), which was unfortunately not further elucidated, ST and PDT, ST and IORT (mean dose 11.1 Gy prescribed to 0.5 cm depth) or ST, IORT and PDT. Although they saw no statistically significant benefit of IORT alone, the combined application of IORT and PDT almost doubled the median survival compared to PDT alone (79 vs. 39.7 months).
Together with the University College London, our group was one of the first centers worldwide to assess the efficiency of IORT using the INTRABEAM system in early stage breast cancer. We have gained experience for more than a decade with the technique and were able to translate the straight-forward setting into treatment schedules of other tumors, including bone metastases, cervical cancer or recurrent oropharyngeal cancer (45,50,57-64). We also offered IORT using the INTRABEAM system to three patients with operable recurrent GBM, hoping to prolong the period till further disease progression (Table 2). All patients had local recurrence after primary resection and received prior adjuvant radiochemotherapy. Before IORT was conducted, maximal doses to pre-irradiated and now potentially involved risk structures were calculated. We then implemented IORT in a straight forward fashion into the routine surgical procedures. In brief, following conventional frameless neuronavigation-guided microsurgical tumor resection, the machine was prepared after the surgeon defined the macroscopic tumor to be fully (or adequately) removed. Following final documentation of the cavity borders using the neuronavigation software, the cavity geometry and potential risk structures were again identified and the most suitable applicator size was chosen by the team of surgeons and radiation oncologists. The expected doses to the risk organs were then calculated and the applicators were fitted into the resection cavity. Any skin or bone that could receive direct or scattered irradiation was shielded using sterile cut-to-size rubbered-lead laminas. Finally, irradiation was delivered and thereafter surgery was finalized as done in the routine setting.
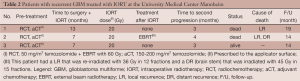
Full table
As all three patients showed rapid local progression after IORT, we assume that—at least in the recurrent setting—the applied doses are highly insufficient to sterilize neither the tumor cavity nor the cellular invasion front from persisting/remaining cells.
The future
There is no doubt that the primary challenge in treating GBM is to achieve improved local control which will hopefully translate into improved survival. However, although up to now far more than 200 patients with high grade-gliomas have received IORT, there still is no randomized data and due to the divergent outcomes reported, IORT cannot be recommended for GBM outside of clinical studies although to our understanding, there may be a benefit which is greater than the risk. To now advance from this uncertain state towards a randomized clinical study, three questions will have to be addressed upfront:
Shall we use IORT for primary or recurrent GBM?
Considering the highly invasive nature of almost all GBMs, the primary goal of IORT, namely to deplete remaining cells at the cavity border is, if at all, exclusively achievable in the primary setting. GBM are highly invasive and rapidly migrate along pre-existing structures such as blood vessels or white matter tracts (65,66). Many patients show multicentric spread in autopsy series that were undetected in brain imaging (67). Furthermore, recurrent gliomas are rich in radio- and chemotherapy resistant (stem and progenitor) cells, which would consequently require much higher IORT doses and larger volumes to achieve growth arrest (68-72). Since nearly all recurrent patients have typically received (I) prior EBRT with doses of ~60 Gy; (II) adjuvant chemotherapy (mostly even at the time progressive disease is noted); and (III) could have underwent re-EBRT or stereotactical radiosurgery (SRS) to progressive areas, there will be no significant margin for dose escalations in most of these cases.
Which patients will benefit from IORT?
Along the line of thought in the response to question 1, it goes without saying that the putatively most suitable population should have tumors that are “treatable” with IORT, which excludes functionally inoperable (co-morbid) patients or patients with multifocal, infratentorial or deep seated tumors. A phase III study on IORT may therefore be critically seen as “picking from the best”, as this population—RPA class I/II patients with circumscribed tumors (and limited perifocal edema)—already has the prerequisite to survive long-term. We believe that this should particularly justify a more aggressive local therapy as the reduction of tumor cell numbers below the “immunogenic threshold” may be eventually achievable only in these cases (see above).
A cohort that may eventually also benefit from IORT is elderly patients, which in the light of an aging population and an increasing incidence of GBM with age, will be a significant portion of patients. Age is consistently shown as a prognostic factor in GBM and it is still controversially discussed whether patients older than 70 years (which were excluded in the Stupp-study) should undergo “classically” fractionated EBRT (60 Gy in 30 fractions), hypofractionated EBRT (45 Gy in 15 fractions) or chemotherapy (with temozolomide) alone after biopsy or surgery (73-76). However, given that the median overall survival in these patients is only about 30-40 weeks, one has to carefully assess whether the application of a purely palliative chemotherapy with all associated risks (thrombocytopenia, lymphocytopenia, neutropenia) or a 3-6-week radiotherapy course (which may resemble up to 20% of the remaining life time) is justified. It may well be that in a carefully selected population of patients (e.g., patients >70 with at least partially resectable tumors and no MGMT promoter methylation), IORT is a non-inferior option in terms of overall survival and a superior option in terms of quality of life compared to EBRT or chemotherapy alone.
What IORT dose should be applied in a future study?
Although fist estimations of dose escalation could be obtained in the Japanese trials and the US trial (with the latter study data limited to children), maximum tolerated doses (MTD) of IORT in GBM are purely speculative as the extent of necrosis in these studies varied from none even after multiple high-dose IOERTs (36) to 25% in previously unirradiated children (54). We believe that one has to separately judge the impact of necroses in the cerebrum (e.g., the frontal, parietal, temporal or occipital lobes) and those of the brain stem and the optical nerves/chiasm. The extent of brain necrosis in the cerebrum may well correlate with improved survival (28) and it should, in our opinion not generally be feared. One should rather consider that IORT can on the long run “enlarge” the tumor cavity and therefore it should be (pre-operatively) taken into account that eloquent areas in close proximity may be affected. Regarding brain stem and optical nerves, tight dose constraints should apply as toxicity rates of 5% (brain stem) and 10% (optical nerves/chiasm) are already expected at single doses of 12 Gy (optical nerve/chiasm) and 12.5 Gy (brain stem) (77). Since we have recently demonstrated an increased (1.2-1.5 times higher) relative biologic effectiveness (RBE) for kV-irradiation (78) the doses to both risk structures will have to be strictly limited to 10 Gy if a low-kV source such as INTRABEAM is used.
To specifically address the latter of these questions we have setup the INTRAGO trial (“Intraoperative Radiotherapy in Glioblastoma”). Within the study, we will apply IORT using the INTRABEAM system in patients with primary supratentorial GBM (intraoperatively confirmed by frozen sections), followed by standard therapy consisting of combined radiochemotherapy and adjuvant chemotherapy. The IORT starting dose was chosen to be 20 Gy prescribed to the applicator surface which will then be escalated up to 40 Gy in a “classical” 3+3 manner. One may argue that, due to the characteristic dose-depth profile of the 50 kV beam, these doses may not sufficiently sterilize deep infiltrated tumor tissue. Depending on the applicator size, the doses at 0.5, 1, 2 and 3 cm depth will be approximately 50%, 30%, 10% and 5% of the surface dose. Consequently, even when assuming the maximum INTRAGO surface radiation dose of 40 Gy (corresponding to a dose of 60 Gy applied with a 6 MV beam), only roughly 2-3 Gy (corresponding to 3-4.5 Gy with a 6 MV beam) are expected in 3 cm depth. The question now is if this technique will compare favorably to electron-based IOERT. We believe that kv-based IORT will increase the local control in the high-dose region (that is the tissue close to the applicator), which compensates the reduction of local control at greater distances (e.g., >0.5 cm) (79). Dose escalation will be terminated if one or more of the following dose-limiting toxicities (DLT) are noted: (I) impaired wound healing or wound infections requiring surgical intervention within two weeks after IORT; (II) cerebral haemorrhage within two weeks after IORT or (III) symptomatic brain necrosis requiring surgical intervention. DLTs will be assessed on the combined analysis of imaging studies (MRI), clinical presentation (physical examination, KPS, current medication) and a neurological assessment using the Late Effects in Normal Tissues Subjective, Objective, Management and Analytic (LENT-SOMA) scales. INTRAGO not only represents the first phase I/II dose escalation study on IORT for GBM, but also the first IORT study in the temozolomide era. The experience gathered with this trial shall then provide the ground for a thorough evaluation of low kV IORT in a subsequent randomized phase III trial.
Conclusions
Despite improved multimodal therapies almost all GBM recur locally suggesting an urgent need for more aggressive first line local treatment strategies such as IORT. Previous (IOERT) studies have shown inconsistent results, mostly due to technical limitations associated with the use of electron tubes. Since mainly target volume coverage appears to have the greatest influence on survival, geometry-adapted IORT with spherically irradiating sources delivering isotropic low-energy (kV) X-rays bear the expectation to consistently improve efficiency of IORT. To assess the safety and the DLT of this procedure, we initiated a phase I/II dose escalating study (INTRAGO, Intraoperative Radiotherapy in Glioblastoma), which shall provide a basis for a future phase III study.
Acknowledgments
Funding: Carl Zeiss Meditec AG supports radiobiological research at the University Medical Center Mannheim. All authors have approved the final version.
Footnote
Provenance and Peer Review: This article was commissioned by the editorial office, Translational Cancer Research for the series “Intraoperative Radiotherapy”. The article has undergone external peer review.
Conflicts of Interest: All authors have completed the ICMJE uniform disclosure form (available at http://dx.doi.org/10.3978/j.issn.2218-676X.2014.01.03). The series “Intraoperative Radiotherapy” was commissioned by the editorial office without any funding or sponsorship. FW served as the unpaid Guest Editor of the series and serves as an unpaid editorial board member of Translational Cancer Research. ES served as the unpaid Guest Editor of the series. The authors have no other conflicts of interest to declare.
Ethical Statement: The authors are accountable for all aspects of the work in ensuring that questions related to the accuracy or integrity of any part of the work are appropriately investigated and resolved.
Open Access Statement: This is an Open Access article distributed in accordance with the Creative Commons Attribution-NonCommercial-NoDerivs 4.0 International License (CC BY-NC-ND 4.0), which permits the non-commercial replication and distribution of the article with the strict proviso that no changes or edits are made and the original work is properly cited (including links to both the formal publication through the relevant DOI and the license). See: https://creativecommons.org/licenses/by-nc-nd/4.0/.
References
- Stupp R, Mason WP, van den Bent MJ, et al. Radiotherapy plus concomitant and adjuvant temozolomide for glioblastoma. N Engl J Med 2005;352:987-96. [PubMed]
- Wallner KE, Galicich JH, Krol G, et al. Patterns of failure following treatment for glioblastoma multiforme and anaplastic astrocytoma. Int J Radiat Oncol Biol Phys 1989;16:1405-9. [PubMed]
- Gaspar LE, Fisher BJ, Macdonald DR, et al. Supratentorial malignant glioma: patterns of recurrence and implications for external beam local treatment. Int J Radiat Oncol Biol Phys 1992;24:55-7. [PubMed]
- Choucair AK, Levin VA, Gutin PH, et al. Development of multiple lesions during radiation therapy and chemotherapy in patients with gliomas. J Neurosurg 1986;65:654-8. [PubMed]
- Gilbert MR. Recurrent glioblastoma: a fresh look at current therapies and emerging novel approaches. Semin Oncol 2011;38:S21-33. [PubMed]
- Harsh GR 4th, Levin VA, Gutin PH, et al. Reoperation for recurrent glioblastoma and anaplastic astrocytoma. Neurosurgery 1987;21:615-21. [PubMed]
- Park JK, Hodges T, Arko L, et al. Scale to predict survival after surgery for recurrent glioblastoma multiforme. J Clin Oncol 2010;28:3838-43. [PubMed]
- Carson KA, Grossman SA, Fisher JD, et al. Prognostic factors for survival in adult patients with recurrent glioma enrolled onto the new approaches to brain tumor therapy CNS consortium phase I and II clinical trials. J Clin Oncol 2007;25:2601-6. [PubMed]
- Pichlmeier U, Bink A, Schackert G, et al. Resection and survival in glioblastoma multiforme: an RTOG recursive partitioning analysis of ALA study patients. Neuro Oncol 2008;10:1025-34. [PubMed]
- Stummer W, Novotny A, Stepp H, et al. Fluorescence-guided resection of glioblastoma multiforme by using 5-aminolevulinic acid-induced porphyrins: a prospective study in 52 consecutive patients. J Neurosurg 2000;93:1003-13. [PubMed]
- Lacroix M, Abi-Said D, Fourney DR, et al. A multivariate analysis of 416 patients with glioblastoma multiforme: prognosis, extent of resection, and survival. J Neurosurg 2001;95:190-8. [PubMed]
- Burnet NG, Jena R, Jefferies SJ, et al. Mathematical modelling of survival of glioblastoma patients suggests a role for radiotherapy dose escalation and predicts poorer outcome after delay to start treatment. Clin Oncol (R Coll Radiol) 2006;18:93-103. [PubMed]
- Do V, Gebski V, Barton MB. The effect of waiting for radiotherapy for grade III/IV gliomas. Radiother Oncol 2000;57:131-6. [PubMed]
- Sampath P, Brem H. Implantable Slow-Release Chemotherapeutic Polymers for the Treatment of Malignant Brain Tumors. Cancer Control 1998;5:130-7. [PubMed]
- Fleming AB, Saltzman WM. Pharmacokinetics of the carmustine implant. Clin Pharmacokinet 2002;41:403-19. [PubMed]
- Brem H, Piantadosi S, Burger PC, et al. Placebo-controlled trial of safety and efficacy of intraoperative controlled delivery by biodegradable polymers of chemotherapy for recurrent gliomas. The Polymer-brain Tumor Treatment Group. Lancet 1995;345:1008-12. [PubMed]
- Bregy A, Shah AH, Diaz MV, et al. The role of Gliadel wafers in the treatment of high-grade gliomas. Expert Rev Anticancer Ther 2013;13:1453-61. [PubMed]
- Gutenberg A, Lumenta CB, Braunsdorf WE, et al. The combination of carmustine wafers and temozolomide for the treatment of malignant gliomas. A comprehensive review of the rationale and clinical experience. J Neurooncol 2013;113:163-74. [PubMed]
- Walker MD, Strike TA, Sheline GE. An analysis of dose-effect relationship in the radiotherapy of malignant gliomas. Int J Radiat Oncol Biol Phys 1979;5:1725-31. [PubMed]
- Bleehen NM, Stenning SP. A Medical Research Council trial of two radiotherapy doses in the treatment of grades 3 and 4 astrocytoma. The Medical Research Council Brain Tumour Working Party. Br J Cancer 1991;64:769-74. [PubMed]
- Tsien C, Moughan J, Michalski JM, et al. Phase I three-dimensional conformal radiation dose escalation study in newly diagnosed glioblastoma: Radiation Therapy Oncology Group Trial 98-03. Int J Radiat Oncol Biol Phys 2009;73:699-708. [PubMed]
- Matsuda T. Intraoperative radiotherapy and confirmation of radiotherapy with special emphasis on the treatment of pancreatic cancer and glioblastoma (Abstr). 4th Asian-Oceanian Congress of Radiology 1983:452-3.
- Goldson AL, Streeter OE Jr, Ashayeri E, et al. Intraoperative radiotherapy for intracranial malignancies. A pilot study. Cancer 1984;54:2807-13. [PubMed]
- Goldson AL, Moorthy C, Ashayeri E, et al. Feasability of intraoperative electron beam irradiation in the management of intracranial malignancies. (Abstr). Radiological Society of North America, 1982.
- Abe M, Fukuda M, Yamano K, et al. Intra-operative irradiation in abdominal and cerebral tumours. Acta Radiol Ther Phys Biol 1971;10:408-16. [PubMed]
- Matsutani M, Matsuda T, Nagashima T, et al. Surgical treatment and radiation therapy for glioblastoma multiforme, with special reference to intraoperative radiotherapy. Gan No Rinsho 1984;30:325-33. [PubMed]
- Matsutani M. Intraoperative radiation therapy for malignant brain tumours. In: Dobelbower RRJea. eds. Intraoperative radiation therapy. CRC: Boca Raton, 1989;137-58.
- Matsutani M, Nakamura O, Nagashima T, et al. Intra-operative radiation therapy for malignant brain tumors: rationale, method, and treatment results of cerebral glioblastomas. Acta Neurochir (Wien) 1994;131:80-90. [PubMed]
- Sakai N, Yamada H, Andoh T, et al. Intraoperative radiation therapy for malignant glioma. Neurol Med Chir (Tokyo) 1989;29:312-8. [PubMed]
- Fujiwara T, Honma Y, Ogawa T, et al. Intraoperative radiotherapy for gliomas. J Neurooncol 1995;23:81-6. [PubMed]
- Shibamoto Y, Yamashita J, Takahashi M, et al. Intraoperative radiation therapy for brain tumors with emphasis on retreatment for recurrence following full-dose external beam irradiation. Am J Clin Oncol 1994;17:396-9. [PubMed]
- Ortiz de Urbina D, Santos M, Garcia-Berrocal I, et al. Intraoperative radiation therapy in malignant glioma: early clinical results. Neurol Res 1995;17:289-94. [PubMed]
- Nemoto K, Ogawa Y, Matsushita H, et al. Intraoperative radiation therapy (IORT) for previously untreated malignant gliomas. BMC Cancer 2002;2:1. [PubMed]
- Wagner W, Schuller P, Willich N, et al. Intraoperative radiotherapy (IORT) in malignant brain tumors. Strahlenther Onkol 1995;171:154-64. [PubMed]
- Gouda J, Brown J, Carter D, et al. Malignant brain tumors treated with IORT. Front Radiat Ther Oncol 1997;31:87-91. [PubMed]
- Sakai N, Yamada H, Andoh T, et al. Intraoperative radiation therapy for malignant glioma. Neurol Med Chir (Tokyo) 1991;31:702-7. [PubMed]
- Schueller P, Micke O, Palkovic S, et al. 12 years’ experience with intraoperative radiotherapy (IORT) of malignant gliomas. Strahlenther Onkol 2005;181:500-6. [PubMed]
- Chung YG, Kim CY, Lee HK, et al. Preliminary experiences with intraoperative radiation therapy (IORT) for the treatment of brain tumors. J Korean Med Sci 1995;10:449-52. [PubMed]
- Takakura K, Kubo O. Treatment of malignant brain tumors. Gan To Kagaku Ryoho 2000;27:449-53. [PubMed]
- Lyons M, Phang I, Eljamel S. The effects of PDT in primary malignant brain tumours could be improved by intraoperative radiotherapy. Photodiagnosis Photodyn Ther 2012;9:40-5. [PubMed]
- Sierrasesumaga L, Calvo Manuel FA, Martin Montaner I, et al. Intraoperative radiotherapy in the multidisciplinary treatment of malignant tumors in children. Preliminary results. An Esp Pediatr 1989;31:269-73. [PubMed]
- Calvo FA, Sierrasesumaga L, Martin I, et al. Intraoperative radiotherapy in the multidisciplinary treatment of pediatric tumors. A preliminary report on initial results. Acta Oncol 1989;28:257-60. [PubMed]
- Schueller P, Palkovic S, Moustakis C, et al. Clinical results and isodose planning of neuronavigation-guided intraoperative radiotherapy (IORT) in 77 brain tumor patients: adequate target volume coverage improve results. Rev Cancer (Madrid) 22(extra) 2008:1-58.
- Keshtgar MR, Vaidya JS, Tobias JS, et al. Targeted intraoperative radiotherapy for breast cancer in patients in whom external beam radiation is not possible. Int J Radiat Oncol Biol Phys 2011;80:31-8. [PubMed]
- Vaidya JS, Baum M, Tobias JS, et al. Targeted intraoperative radiotherapy (TARGIT) yields very low recurrence rates when given as a boost. Int J Radiat Oncol Biol Phys 2006;66:1335-8. [PubMed]
- Vaidya JS, Joseph DJ, Tobias JS, et al. Targeted intraoperative radiotherapy versus whole breast radiotherapy for breast cancer (TARGIT-A trial): an international, prospective, randomised, non-inferiority phase 3 trial. Lancet 2010;376:91-102. [PubMed]
- Wenz F, Welzel G, Blank E, et al. Intraoperative radiotherapy as a boost during breast-conserving surgery using low-kilovoltage X-rays: the first 5 years of experience with a novel approach. Int J Radiat Oncol Biol Phys 2010;77:1309-14. [PubMed]
- Leonardi MC, Maisonneuve P, Mastropasqua MG, et al. Accelerated partial breast irradiation with intraoperative electrons: using GEC-ESTRO recommendations as guidance for patient selection. Radiother Oncol 2013;106:21-7. [PubMed]
- Leonardi MC, Maisonneuve P, Mastropasqua MG, et al. How do the ASTRO consensus statement guidelines for the application of accelerated partial breast irradiation fit intraoperative radiotherapy? A retrospective analysis of patients treated at the European Institute of Oncology. Int J Radiat Oncol Biol Phys 2012;83:806-13. [PubMed]
- Vaidya JS, Wenz F, Bulsara M, et al. Risk-adapted targeted intraoperative radiotherapy versus whole-breast radiotherapy for breast cancer: 5-year results for local control and overall survival from the TARGIT-A randomised trial. Lancet 2014;383:603-13. [PubMed]
- Curry WT Jr, Cosgrove GR, Hochberg FH, et al. Stereotactic interstitial radiosurgery for cerebral metastases. J Neurosurg 2005;103:630-5. [PubMed]
- Di Lorenzo N, Cavedon C, Paier F, et al. Interstitial radiosurgery with the photon radiosurgery system in the minimally-invasive treatment of selected deep-seated brain tumors. J Chemother 2004;16:70-4. [PubMed]
- Gallina P, Francescon P, Cavedon C, et al. Stereotactic interstitial radiosurgery with a miniature X-ray device in the minimally invasive treatment of selected tumors in the thalamus and the basal Ganglia. Stereotact Funct Neurosurg 2002;79:202-13. [PubMed]
- Kalapurakal JA, Goldman S, Stellpflug W, et al. Phase I study of intraoperative radiotherapy with photon radiosurgery system in children with recurrent brain tumors: preliminary report of first dose level (10 Gy). Int J Radiat Oncol Biol Phys 2006;65:800-8. [PubMed]
- Mulhern RK, Palmer SL. Neurocognitive late effects in pediatric cancer. Curr Probl Cancer 2003;27:177-97. [PubMed]
- Moore BD 3rd. Neurocognitive outcomes in survivors of childhood cancer. J Pediatr Psychol 2005;30:51-63. [PubMed]
- Blank E, Kraus-Tiefenbacher U, Welzel G, et al. Single-center long-term follow-up after intraoperative radiotherapy as a boost during breast-conserving surgery using low-kilovoltage x-rays. Ann Surg Oncol 2010;17:352-8. [PubMed]
- Kraus-Tiefenbacher U, Bauer L, Scheda A, et al. Intraoperative radiotherapy (IORT) is an option for patients with localized breast recurrences after previous external-beam radiotherapy. BMC Cancer 2007;7:178. [PubMed]
- Reis T, Schneider F, Welzel G, et al. Intraoperative radiotherapy during kyphoplasty for vertebral metastases (Kypho-IORT): first clinical results. Tumori 2012;98:434-40. [PubMed]
- Schmidt R, Wenz F, Reis T, et al. Kyphoplasty and intra-operative radiotheray, combination of kyphoplasty and intra-operative radiation for spinal metastases: technical feasibility of a novel approach. Int Orthop 2012;36:1255-60. [PubMed]
- Schneider F, Greineck F, Clausen S, et al. Development of a novel method for intraoperative radiotherapy during kyphoplasty for spinal metastases (Kypho-IORT). Int J Radiat Oncol Biol Phys 2011;81:1114-9. [PubMed]
- Wenz F, Schneider F, Neumaier C, et al. Kypho-IORT--a novel approach of intraoperative radiotherapy during kyphoplasty for vertebral metastases. Radiat Oncol 2010;5:11. [PubMed]
- Neumaier C, Elena S, Grit W, et al. TARGIT-E(lderly)--prospective phase II study of intraoperative radiotherapy (IORT) in elderly patients with small breast cancer. BMC Cancer 2012;12:171. [PubMed]
- Abo-Madyan Y, Goessler U, Vent J, et al. Intraoperative radiotherapy (IORT) for recurrent head and neck squamous cell carcinoma (SCC) using a mobile 50 kilovoltage (KV) mini-accelerator. Strahlenther Onkol 2013;189:99-100.
- Scherer J. The forms of growth in gliomas and their practical significance. Brain 1940;63:1-35.
- Holland EC. Glioblastoma multiforme: the terminator. Proc Natl Acad Sci U S A 2000;97:6242-4. [PubMed]
- Batzdorf U, Malamud N. The Problem of Multicentric Gliomas. J Neurosurg 1963;20:122-36. [PubMed]
- Bao S, Wu Q, McLendon RE, et al. Glioma stem cells promote radioresistance by preferential activation of the DNA damage response. Nature 2006;444:756-60. [PubMed]
- Campos B, Wan F, Farhadi M, et al. Differentiation therapy exerts antitumor effects on stem-like glioma cells. Clin Cancer Res 2010;16:2715-28. [PubMed]
- Gao X, McDonald JT, Hlatky L, et al. Acute and fractionated irradiation differentially modulate glioma stem cell division kinetics. Cancer Res 2013;73:1481-90. [PubMed]
- Golding SE, Rosenberg E, Adams BR, et al. Dynamic inhibition of ATM kinase provides a strategy for glioblastoma multiforme radiosensitization and growth control. Cell Cycle 2012;11:1167-73. [PubMed]
- Ropolo M, Daga A, Griffero F, et al. Comparative analysis of DNA repair in stem and nonstem glioma cell cultures. Mol Cancer Res 2009;7:383-92. [PubMed]
- Keime-Guibert F, Chinot O, Taillandier L, et al. Radiotherapy for glioblastoma in the elderly. N Engl J Med 2007;356:1527-35. [PubMed]
- Wick W, Platten M, Meisner C, et al. Temozolomide chemotherapy alone versus radiotherapy alone for malignant astrocytoma in the elderly: the NOA-08 randomised, phase 3 trial. Lancet Oncol 2012;13:707-15. [PubMed]
- Roa W, Brasher PM, Bauman G, et al. Abbreviated course of radiation therapy in older patients with glioblastoma multiforme: a prospective randomized clinical trial. J Clin Oncol 2004;22:1583-8. [PubMed]
- Malmström A, Grønberg BH, Marosi C, et al. Temozolomide versus standard 6-week radiotherapy versus hypofractionated radiotherapy in patients older than 60 years with glioblastoma: the Nordic randomised, phase 3 trial. Lancet Oncol 2012;13:916-26. [PubMed]
- Marks LB, Yorke ED, Jackson A, et al. Use of normal tissue complication probability models in the clinic. Int J Radiat Oncol Biol Phys 2010;76:S10-9. [PubMed]
- Liu Q, Schneider F, Ma L, et al. Relative Biologic Effectiveness (RBE) of 50 kV X-rays measured in a phantom for intraoperative tumor-bed irradiation. Int J Radiat Oncol Biol Phys 2013;85:1127-33. [PubMed]
- Herskind C, Griebel J, Kraus-Tiefenbacher U, et al. Sphere of equivalence--a novel target volume concept for intraoperative radiotherapy using low-energy X rays. Int J Radiat Oncol Biol Phys 2008;72:1575-81. [PubMed]