Potential predictive value of JAK2 expression for Pan-cancer response to PD-1 blockade immunotherapy
Introduction
Immunotherapy represents a recent major breakthrough in cancer treatment. In particular, programmed cell death protein-1 (PD-1), programmed cell death ligand 1 (PD-L1), and PD-L2 pathways constitute key immune checkpoints. The PD-1 inhibitors nivolumab and pembrolizumab have induced durable control and shown a survival benefit in immunogenic tumors, such as non-small cell lung carcinoma, melanoma, renal cell carcinoma, and head and neck cancer (1).
Persisting expression of PD-L1 on the surface of tumor cells and partial immune cells can be induced by tumor cell intrinsic and extrinsic signals, which leads to immune escape of the tumor (2,3). An increasing number of clinical trials have shown a high objective response in patients with positive PD-L1 expression in tumor samples (4,5). It was also revealed that the number of tumor infiltrating lymphocytes was significantly associated with objective response toward anti-PD-1/PD-L1 therapy (6). Furthermore, recent advances in immuno- genomics have demonstrated that tumors with a status of high mutational burden, abundant neoantigen, and microsatellite-instability-high (MSI-H) demonstrated active response to anti-PD-1/PD-L1 therapy and longer overall patient survival (7-9). Additionally, oncogenic viruses such as Epstein-Barr virus (EBV) or human papillomavirus (HPV) were also associated with an inflamed tumor microenvironment, which potentially resulted in a favorable clinical outcome in response to anti-PD-1/PD-L1 therapy (10,11). Moreover, the cytolytic activity (“CYT”), which was assessed by measuring granzyme A (GZMA) and perforin 1 (PRF1) expression levels, was associated with inflamed tumors and was considered to be influenced by the infiltration of CD8+ cytolytic T lymphocytes (CTLs) (12,13). Thus, several factors were found to facilitate the antitumor activity of the immune checkpoint inhibitors mentioned above, which raises the question of whether a clear biomarker exists that correlates with these factors.
Notably, current studies have discovered that Janus kinase 2 (JAK2), a classical inflammatory factor, showed a significant correlation with PD-L1, encoded by the CD274 gene (14). Specifically, a cryptic JAK2-CD274 rearrangement was generated by a microdeletion spanning the 3'JAK2-5'CD274 region (15). Furthermore, in head and neck cancer, a significant association was found between PD-L1 expression and phosphorylation of JAK2 as detected by immunohistochemistry (16). Patients with melanoma carrying a JAK2 mutation exhibit an acquired resistance to anti-PD-1/PD-L1 therapy (17); a similar situation has been discovered in a mouse model of breast tumor and melanoma (18). Based on these findings, we speculated that JAK2 expression might represent a potential biomarker for response to PD-1 blockade immunotherapy.
In the current study, we classified a large set of TCGA Pan-Cancer samples into three groups by measuring their JAK2 mRNA expression levels. The object of this TCGA Pan-Cancer analysis was to determine the associations between JAK2 status and the mRNA expression and mutational burden of PD-L1, CD8+CTLs (as measured by CD8A expression), CYT expression, and oncogenic viral infection, which would likely provide strategic information for guiding the treatment of immune checkpoint blockade.
Methods
Experimental design
We studied 9,315 samples from The Cancer Genome Atlas (TCGA) database, involving 31 types of cancers. RNA sequencing (RNA-Seq) data of level 3 reads per kilobase of transcript per million mapped reads (RPKM) were obtained from TCGA Data Portal (https://gdc-portal.nci.nih.gov/) and log2-transformed. Amplification of the locus for PD-L1, MSI status, infection of oncogenic viruses, mutation burden, and neoantigen number were analyzed in this study. The capacity of samples varied from different indices owing to data availability. The MSI status was available for 1,010 samples including samples of colon and rectal adenocarcinoma (COAD) (N=285), uterine carcinosarcoma (UCS) (N=55), esophageal carcinoma (ESCA) (N=88), stomach adenocarcinoma (STAD) (N=414), and uterine corpus endometrioid carcinoma (UCEC) (N=168). The infection status of oncogenic viruses, such as EBV, HPV, and hepatitis B virus (HBV) was available in 6,385 samples. Somatic mutational of 6,257 samples and neoantigens of 3,763 were accessible. Altogether, samples of 31 cancer types (N=9,315) were included in the analysis.
Statistical analyses
According to the log 2-transformed RPKM values of JAK2, all of the TCGA samples were divided into three groups as follows: High-JAK2 (log2JAK2 ≥8.6037, N=3,105), Medium-JAK2 (7.8019≤ log2JAK2 <8.6037, N=3,105), and Low-JAK2 (log2JAK2 <7.8019, N=3,105). Several predicted biomarkers, such as somatic mutations, neoantigens, CD8A expression level, CYT activity, and the mRNA expression of PD-L1, were also log2- transformed. The statistical correlations between variables including the above biomarkers, JAK2, and oncogenic viruses were analyzed. The association between MSI status and JAK2 expression was tested in all samples. Statistical methods including the Mann-Whitney U and correlation analysis were applied in genomic data analysis. Statistical analyses were conducted using GraphPad Prism (version 7.0, LaJolla, CA) Scatter dot plot and box and whisker plots indicate median and 95% confidence intervals (CI), and Chi-square values. All reported P values were two-tailed and for all analyses, P≤0.05 was considered statistically significant, unless otherwise specified.
Results
High JAK2 expression is associated with mRNA expression and gene amplification of PD-L1
To study the relationship between the mRNA expression of JAK2 and PD-L1 expression in thirty-one solid tumors, we investigated the TCGA databases, which include 9,315 tumor samples from thirty-one cancer types. The median log2-transformed mRNA expression values of JAK2 and PD-L1 were 8.2 and 4.8, respectively. The mRNA expression of JAK2and PD-L1 varied according to cancer type (P<0.0001; Figure 1A,B). Among the solid tumors, STAD and diffuse large B-cell lymphoma (DLBC) had the highest JAK2 median values (9.3 and 9.2, respectively; Figure 1A), followed by kidney clear cell carcinoma (KIRC). In contrast, liver hepatocellular carcinoma and uveal melanoma (UVM) had the lowest JAK2 median values (6.9 and 6.8, respectively; Figure 1A). As expected, the solid tumors with high JAK2 expression, such as STAD and DLBC, showed high mRNA expression of PD-L1 (Figure 1B).
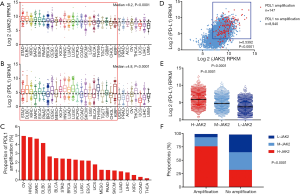
Because amplification of the gene locus for PD-L1 has been reported to serve as a good predictive biomarker of the response to anti-PD-1/PD-L1 therapy (19,20). The frequency of this amplification was analyzed in the various cancer types (Figure 1C). Ovarian serous cystadenocarcinoma (OV), head and neck squamous cell carcinoma (HNSC), sarcoma (SARC), and DLBC showed the highest proportion of amplification (Figure 1C). As in previous reports, the mRNA expression levels of JAK2 and PD-L1 were significantly correlated (r=0.5392, P<0.0001; Figure 1D). Both JAK2 and PD-L1 exhibited high mRNA expression levels under the status of gene locus amplification for PD-L1.
We also found that the mRNA expression of PD-L1 in the H-JAK2 group was higher than that in M-JAK2 or L-JAK2 (P<0.0001; Figure 1E). The H-JAK2 samples constituted a larger proportion in the group exhibiting gene locus amplification of PD-L1 than that in the non-amplified group (76.9% vs. 32.6%, P<0.0001; Figure 1F). The proportion of M-JAK2 or L-JAK2 was prominently lower in the amplification compared to the no-amplification (Figure 1F).
High JAK2 expression is associated with high mutational burden, neoantigen, and MSI-H
We sought to investigate the correlation between high JAK2 expression and mutational burden. In every type of tumor, samples were divided according to their JAK2 expression into three groups: high, medium, and low. The proportion of H-JAK2 was quite high in STAD (76.6%) and DLBC (72.9%), but significantly low in liver hepatocellular carcinoma (LICH, 3.4%) and UVM (2.5%; Figure 2A). The proportion of JAK2 expression differed in all tumors (P=0.0001; Figure 2A).
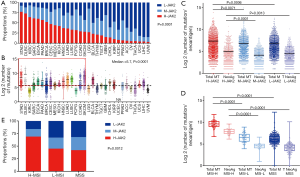
In addition, we compared the mutational burden of every tumor and discovered that tumors with H-JAK2, such as STAD and DLBC, mostly bore high mutational burden (Figure 2B). UVM with L-JAK2 showed low mutational burden. However, among H-JAK2 tumors, kidney chromophobe (KICH) presented low mutation burden, whereas LICH, compared with other L-JAK2 tumors such as OV and thyroid carcinoma (THCA), presented higher mutation burden (Figure 2B). We also discovered a clear association between mutational burden and the number of neoantigens (r=0.9386, P<0.0001; Figure S1). Further analysis linked H-JAK2 to not only the highest mutational burden but also the highest emergence of neoantigens, compared with M-JAK2 or L-JAK2 in those tumors (Figure 2C).
MSI-H status indicates better response to immunotherapy, especially in COAD (21). We therefore examined the relationship between JAK2 expression and MSI status. We found that COAD, ESCA, STAD, UCEC, and UCS showed changed MSI status. MSI-H tumors were loaded with the heaviest mutational burden (P<0.0001; Figure 2D), including STAD and COAD (P<0.0001; Figure S2A,B). Furthermore, the MSI-H group showed the highest proportion of H-JAK2 compared to MSI-L and microsatellite stability (MSS) groups (P=0.0012; Figure 2E), especially in STAD and COAD (P<0.0001; Figure S2C,D).
High JAK2 expression is associated with tumor CYT activity and oncogenic viruses
To determine whether high JAK2 expression is associated with CYT activity and oncogenic viruses, we sought to analyze the alterations of tumor CYT activity and oncogenic virus infection in H-JAK2 patients. Using RNA-Seq data from thousands of TCGA solid tumor biopsies, we first found that GZMA and PRF1 were tightly co-expressed in TCGA samples and exhibited a strong correlation across the TCGA database (r=0.8754, P<0.0001; Figure 3A). Patients with H-JAK2 showed higher expression of PRF1 and GZMA than those with M-JAK2 and L-JAK2 (P<0.0001; Figure 3B,C).
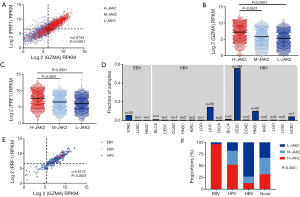
We next investigated the distribution of oncogenic virus infection including HPV, EBV, and HBV. Consistent with previous analysis of TCGA data, STAD exhibited the highest fraction of EBV infection (5.5%). HPV infection was most abundant in cervical cancer (55.6%), but also frequent in head and neck cancer (Figure 3D). HBV was primarily observed in liver samples (14.2%). Consistent with a previous report that demonstrated that oncogenic virus infection increased the CYT activity of a tumor (12), we found that the tumor CYT activity was notably associated with oncogenic viruses and that HPV or EBV positive samples demonstrated a high CYT expression (Figure 3E, Figure S3), whereas HBV positive samples showed a low CYT expression. Further investigation revealed that EBV positive samples featured the highest proportion of H-JAK2, whereas HBV positive samples had the lowest proportion of H-JAK2 (P<0.001; Figure 3F).
High JAK2 expression is associated with tumor infiltrating CD8+CTLs
Central to the efficacy of immune checkpoint blockade is the requirement for immune cells to infiltrate into tumors (6). As tumor-infiltrating CD8+CTLs mediate the antitumor response of immunotherapy, we aimed to discover the association between H-JAK2 and CD8A expression. Notably, we found that there was a significant correlation between CD8A and interferon gamma (IFNG), and between GZMB and the mRNA expression of PD-1 (Figure 4A,B,C). Positive expression for each factor was defined as above-median expression. In addition, the H-JAK2 group showed a large number of patients with tumor infiltrating IFNγ+CD8A+, GZMB+CD8A+, and PD-1+CD8A+ CTLs (Figure 4A,B,C). Patients with M-JAK2 or L-JAK2 showed lower mRNA expression of IFN-γ, GZMB, and PD-1 than H-JAK2, and patients with high CD8A expression encompassed a higher proportion of H-JAK2 than those with medium or low CD8A expression (Figure 4D). The TCGA samples were divided equally into three groups according the RPKM values of CD8A. As expected, the samples with H-CD8A exhibited the highest proportion of H-JAK2 (P<0.0001; Figure 4E).
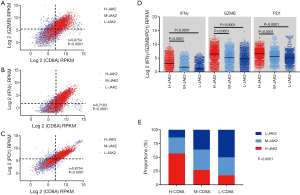
Discussion
Based on TCGA dataset information, we classified thirty-one types of cancer into three groups according on their JAK2 mRNA expression levels as assessed by RNA-Seq. The key finding of the current study consisted of the discovery that the mRNA expressions of PD-L1, mutational burdens, neoantigens, CYT activity, oncogenic viruses, and CD8+CTLs were significantly correlated with high JAK2 expression. Thus, our results potentially indicate that JAK2 might serve as a robust biomarker in Pan-Cancer; however, limited information was provided for guiding immunotherapy and biomarker strategies.
Although several studies have demonstrated that PD-L1 expression on the surface of tumor cells and immune cells was a predictive biomarker of patient response to anti-PD-1/PD-L1 therapies in several cancer types (4,22), not all PD-L1-positive patients respond well to such treatments. In addition, the undefined optimal cutoff of PD-L1, such as 5% or 1%, and its diverse indication in different cancer types as well as adverse patient response to various anti-PD-1/PD-L1 drugs have limited the application of this immune therapy (23). Previous studies have clearly suggested that most human cancers, such as STAD, DLBC, ESCA, and lung adenocarcinoma (LUAD), present variable copy number gains of chromosome 9p24.1, a genomic region that includes the genes for PD-L1, PD-L2 (another ligand of PD-1), and JAK2, which activates the IFNγ/JAK/STAT pathway (15,24-26). The results of our study also confirmed that the mRNA expression levels of JAK2 and PD-L1 were prominently correlated. The amplification of the gene loci for JAK2 and PD-L1 was also highly consistent based on TCGA dataset information. In addition, recent studies have shown that the aberrant status of JAK2 mutation led to a lack of PD-L1 expression upon IFNγ exposure mediated by an inability to signal through the IFNγ receptor pathway (17). Furthermore, JAK2 loss-of-function alterations as noted in TCGA confer adverse outcomes in patients who showed a resistance to anti-PD-1/PD-L1 therapy (17,27).
The mutational burden varies among cancer types and is closely associated with the number of nonsynonymous mutations. Recent results have demonstrated that high mutational burden and nonsynonymous mutations improve the clinical outcome of anti-PD-1 antibody treatment (8,28,29). In our study, the cancer types with high proportion of H-JAK2 such as STAD, DLBC, and ESCA were accompanied with high mutational burden. In contrast, a low proportion of H-JAK2 generally occurred with low mutational burden in OV, THCA, and UVM. Notably, we found a prominent association between mutational burden and neoantigens. Theoretically, it would be feasible to calculate the interaction between a specific mutation and HLA genotype to predict the specific neoantigens (30). Furthermore, the number of neoantigens of the H-JAK2 group was significantly higher than that of M-JAK2 and L-JAK2 over a total of thirty-one kinds of cancers. As shown by a recent study (28) and our report, MSI status was also correlated with mutational burden and neoantigens. In our results, the MSI-H samples displayed a higher proportion of H-JAK2 than MSI-L and MSS. Previous findings discovered that patient MSI status was prominently associated with their response to immunotherapy (9,31,32). For example, patients with COAD and MSI-H benefit more from anti-PD-1/PD-L1 therapy, whereas the patients with MSI-L or MSS fail to respond (21).
In addition, another crucial issue related to treatment response is that some tumors are “inflamed” with effect or T cell infiltration whereas others are not. Growing evidence suggests that inflamed tumors respond more actively than non-inflamed tumors (33). As a key factor of inflammation, JAK2 exhibited a significant association with tumor infiltrating CD8+ CTLs as affirmed in this study. Furthermore, we found that not only PD-L1 but also immune molecules, such as IFNγ+CD8A+, GZMB+CD8A+, and PD-1+CD8A+, were prominently associated with high JAK2 expression in the tumor microenvironment. In addition, viruses giving rise to a subset of inflamed malignancies are also known to activate high affinity antigen-specific CTLs against non-self-viral antigens (34-37). This phenomenon increases the immunogenicity of the tumor by activating the IFNγ pathway and leads to unregulated JAK2 expression. Consistent with this phenomenon, tumors with oncogenic virus infection, such as HPV or EBV, showed high proportions of H-JAK2. Of note, samples with HBV infection exhibited a lower proportion of H-JAK2 than uninfected samples. This indicated that the samples with HBV infection, especially those in hepatocellular carcinoma, were in an immunosuppressive state. Oncogenic virus infection increases the tumor CYT activity as measured by GZMA and PRF1, and was also found to be markedly correlated with H-JAK2.
Our study was limited considering the required clinical validation of JAK2 cutoff values; however, the potential association between H-JAK2 and several predicted biomarkers across most cancer types identified by using TCGA project database information should be highlighted. Our results were fundamentally consistent with previous findings, such as for STAD, DLBC, and LUAD, which showed relatively high proportion of H-JAK2 and better response to anti-PD-1/PD-L1. These findings provide a reference for future preclinical and clinical studies regarding the application of JAK2 expression toward the assessment of immuno-genomic features among cancer types.
In summary, analysis of TCGA samples has revealed that high JAK2 expression was clearly associated with high mRNA expression and mutational burden of PD-L1, CD8+CTLs, CYT expression, and oncogenic viral infection, which are likely good indicators for the response to anti-PD-1/PD-L1 therapy. Our data thus support the combination of H-JAK2 and multiple biomarker assays, and may facilitate the discovery of new anti-PD-1/PD-L1 therapeutic strategies that could screen a cohort of patients who may acquire greater benefit from immunotherapy.
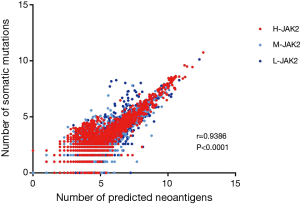
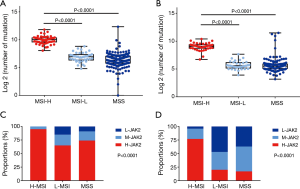
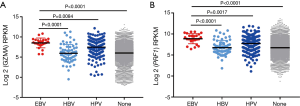
Acknowledgments
The authors appreciate the generosity of Chan-Young Ock and his colleagues at the TCGA Network for sharing the huge amount of data. We would like to thank Editage (
Funding: This work was supported by the National Nature Science Foundation of China (Grant No. 81372283, 81472711, 81401180, 81672756 and 91540111), Guangdong Province Universities and Colleges Pearl River Scholar Funded Scheme (2015), and the Natural Science Foundation of Guangdong Province (Grant No. 2014A030311013).
Footnote
Conflicts of Interest: All authors have completed the ICMJE uniform disclosure form (available at http://dx.doi.org/10.21037/tcr.2018.04.09). The authors have no conflicts of interest to declare.
Ethical Statement: The authors are accountable for all aspects of the work in ensuring that questions related to the accuracy or integrity of any part of the work are appropriately investigated and resolved. The study was conducted in accordance with the Declaration of Helsinki (as revised in 2013). Institutional ethical approval and informed consent were waived.
Open Access Statement: This is an Open Access article distributed in accordance with the Creative Commons Attribution-NonCommercial-NoDerivs 4.0 International License (CC BY-NC-ND 4.0), which permits the non-commercial replication and distribution of the article with the strict proviso that no changes or edits are made and the original work is properly cited (including links to both the formal publication through the relevant DOI and the license). See: https://creativecommons.org/licenses/by-nc-nd/4.0/.
References
- Chow LQ, Haddad R, Gupta S, et al. Antitumor Activity of Pembrolizumab in Biomarker-Unselected Patients With Recurrent and/or Metastatic Head and Neck Squamous Cell Carcinoma: Results From the Phase Ib KEYNOTE-012 Expansion Cohort. J Clin Oncol 2016;34:3838-45. [Crossref] [PubMed]
- Duraiswamy J, Freeman GJ, Coukos G. Therapeutic PD-1 pathway blockade augments with other modalities of immunotherapy T-cell function to prevent immune decline in ovarian cancer. Cancer Res 2013;73:6900-12. [Crossref] [PubMed]
- Akbay EA, Koyama S, Carretero J, et al. Activation of the PD-1 pathway contributes to immune escape in EGFR-driven lung tumors. Cancer Discov 2013;3:1355-63. [Crossref] [PubMed]
- Taube JM, Klein A, Brahmer JR, et al. Association of PD-1, PD-1 ligands, and other features of the tumor immune microenvironment with response to anti-PD-1 therapy. Clin Cancer Res 2014;20:5064-74. [Crossref] [PubMed]
- Addeo R, Caraglia M, Iuliano G. Pembrolizumab: the value of PDL1 biomarker in head and neck cancer. Expert Opin Biol Ther 2016;16:1075-8. [Crossref] [PubMed]
- Tumeh PC, Harview CL, Yearley JH, et al. PD-1 blockade induces responses by inhibiting adaptive immune resistance. Nature 2014;515:568-71. [Crossref] [PubMed]
- Rosenberg JE, Hoffman-Censits J, Powles T, et al. Atezolizumab in patients with locally advanced and metastatic urothelial carcinoma who have progressed following treatment with platinum-based chemotherapy: a single-arm, multicentre, phase 2 trial. Lancet 2016;387:1909-20. [Crossref] [PubMed]
- Rizvi NA, Hellmann MD, Snyder A, et al. Cancer immunology. Mutational landscape determines sensitivity to PD-1 blockade in non-small cell lung cancer. Science 2015;348:124-8. [Crossref] [PubMed]
- Meng X, Huang Z, Teng F, et al. Predictive biomarkers in PD-1/PD-L1 checkpoint blockade immunotherapy. Cancer Treat Rev 2015;41:868-76. [Crossref] [PubMed]
- Nizard M, Sandoval F, Badoual C, et al. Immunotherapy of HPV-associated head and neck cancer: Critical parameters. Oncoimmunology 2013;2:e24534 [Crossref] [PubMed]
- Chen BJ, Chapuy B, Ouyang J, et al. PD-L1 expression is characteristic of a subset of aggressive B-cell lymphomas and virus-associated malignancies. Clin Cancer Res 2013;19:3462-73. [Crossref] [PubMed]
- Rooney MS, Shukla SA, Wu CJ, et al. Molecular and genetic properties of tumors associated with local immune cytolytic activity. Cell 2015;160:48-61. [Crossref] [PubMed]
- Danilova L, Wang H, Sunshine J, et al. Association of PD-1/PD-L axis expression with cytolytic activity, mutational load, and prognosis in melanoma and other solid tumors. Proc Natl Acad Sci U S A 2016;113:E7769-77. [Crossref] [PubMed]
- Van Roosbroeck K, Ferreiro JF, Tousseyn T, et al. Genomic alterations of the JAK2 and PDL loci occur in a broad spectrum of lymphoid malignancies. Genes Chromosomes Cancer 2016;55:428-41. [Crossref] [PubMed]
- Kataoka K, Shiraishi Y, Takeda Y, et al. Aberrant PD-L1 expression through 3'-UTR disruption in multiple cancers. Nature 2016;534:402-6. [Crossref] [PubMed]
- Concha-Benavente F, Srivastava RM, Trivedi S, et al. Identification of the Cell-Intrinsic and -Extrinsic Pathways Downstream of EGFR and IFNgamma That Induce PD-L1 Expression in Head and Neck Cancer. Cancer Res 2016;76:1031-43. [Crossref] [PubMed]
- Shin DS, Zaretsky JM, Escuin-Ordinas H, et al. Primary Resistance to PD-1 Blockade Mediated by JAK1/2 Mutations. Cancer Discov 2017;7:188-201. [Crossref] [PubMed]
- Benci JL, Xu B, Qiu Y, et al. Tumor Interferon Signaling Regulates a Multigenic Resistance Program to Immune Checkpoint Blockade. Cell 2016;167:1540-54 e12.
- Roemer MG, Advani RH, Ligon AH, et al. PD-L1 and PD-L2 Genetic Alterations Define Classical Hodgkin Lymphoma and Predict Outcome. J Clin Oncol 2016;34:2690-7. [Crossref] [PubMed]
- Ikeda S, Goodman AM, Cohen PR, et al. Metastatic basal cell carcinoma with amplification of PD-L1: exceptional response to anti-PD1 therapy. NPJ Genom Med 2016;1:16037. [Crossref] [PubMed]
- Le DT, Uram JN, Wang H, et al. PD-1 Blockade in Tumors with Mismatch-Repair Deficiency. N Engl J Med 2015;372:2509-20. [Crossref] [PubMed]
- Taube JM, Young GD, McMiller TL, et al. Differential Expression of Immune-Regulatory Genes Associated with PD-L1 Display in Melanoma: Implications for PD-1 Pathway Blockade. Clin Cancer Res 2015;21:3969-76. [Crossref] [PubMed]
- Gibney GT, Weiner LM, Atkins MB. Predictive biomarkers for checkpoint inhibitor-based immunotherapy. Lancet Oncol 2016;17:e542-51. [Crossref] [PubMed]
- Xie QK, Zhao YJ, Pan T, et al. Programmed death ligand 1 as an indicator of pre-existing adaptive immune responses in human hepatocellular carcinoma. Oncoimmunology 2016;5:e1181252 [Crossref] [PubMed]
- Lee SJ, Jang BC, Lee SW, et al. Interferon regulatory factor-1 is prerequisite to the constitutive expression and IFN-gamma-induced upregulation of B7-H1 (CD274). FEBS Lett 2006;580:755-62. [Crossref] [PubMed]
- Ikeda S, Okamoto T, Okano S, et al. PD-L1 Is Upregulated by Simultaneous Amplification of the PD-L1 and JAK2 Genes in Non-Small Cell Lung Cancer. J Thorac Oncol 2016;11:62-71. [Crossref] [PubMed]
- Ansell SM, Lesokhin AM, Borrello I, et al. PD-1 Blockade with Nivolumab in Relapsed or Refractory Hodgkin's Lymphoma. N Engl J Med 2015;372:311-9. [Crossref] [PubMed]
- Liontos M, Anastasiou I, Bamias A, et al. DNA damage, tumor mutational load and their impact on immune responses against cancer. Ann Transl Med 2016;4:264. [Crossref] [PubMed]
- Gubin MM, Zhang X, Schuster H, et al. Checkpoint blockade cancer immunotherapy targets tumour-specific mutant antigens. Nature 2014;515:577-81. [Crossref] [PubMed]
- Lu YC, Robbins PF. Cancer immunotherapy targeting neoantigens. Semin Immunol 2016;28:22-7. [Crossref] [PubMed]
- Ock CY, Keam B, Kim S, et al. Pan-Cancer Immunogenomic Perspective on the Tumor Microenvironment Based on PD-L1 and CD8 T-Cell Infiltration. Clin Cancer Res 2016;22:2261-70. [Crossref] [PubMed]
- Gargiulo P, Della Pepa C, Berardi S, et al. Tumor genotype and immune microenvironment in POLE-ultramutated and MSI-hypermutated Endometrial Cancers: New candidates for checkpoint blockade immunotherapy? Cancer Treat Rev 2016;48:61-8. [Crossref] [PubMed]
- Tang H, Wang Y, Chlewicki LK, et al. Facilitating T Cell Infiltration in Tumor Microenvironment Overcomes Resistance to PD-L1 Blockade. Cancer Cell 2016;29:285-96. [Crossref] [PubMed]
- Welters MJ, Kenter GG, Piersma SJ, et al. Induction of tumor-specific CD4+ and CD8+ T-cell immunity in cervical cancer patients by a human papillomavirus type 16 E6 and E7 long peptides vaccine. Clin Cancer Res 2008;14:178-87. [Crossref] [PubMed]
- Fogg MH, Wirth LJ, Posner M, et al. Decreased EBNA-1-specific CD8+ T cells in patients with Epstein-Barr virus-associated nasopharyngeal carcinoma. Proc Natl Acad Sci U S A 2009;106:3318-23. [Crossref] [PubMed]
- Badrinath N, Heo J, Yoo SY. Viruses as nanomedicine for cancer. Int J Nanomedicine 2016;11:4835-47. [Crossref] [PubMed]
- Badoual C, Hans S, Merillon N, et al. PD-1-expressing tumor-infiltrating T cells are a favorable prognostic biomarker in HPV-associated head and neck cancer. Cancer Res 2013;73:128-38. [Crossref] [PubMed]