Prostate-specific membrane antigen in prostate cancer imaging and treatment
Introduction/background
Prostate cancer (PC) is the most common cancer and the third most common cause of cancer deaths in American men (1). Despite its prevalence and the recent advances in its treatment, the ability of standard imaging technologies, such as computed tomography (CT) and technetium-99m (99mTc) bone scintigraphy, to accurately identify PC continues to be a problem in the field (2). This limitation can result in management dilemmas at all stages of the disease, from initial detection (3) and biochemical recurrence (4), to the development of metastases (5). It has also led to issues in clinical trial design for PC in that standard imaging used for the Response Evaluation Criteria in Solid Tumors (RECIST) do not address key features of the disease (6), in part leading to the development of Prostate Cancer Clinical Trials Working Group (PCWG) recommendations for clinical trial design (7,8).
The limitations in standard imaging have led to the study of other imaging modalities such as magnetic resonance imaging (MRI) and positron emission tomography (PET). Novel radiotracers targeting different aspects of PC biology have been evaluated for PET imaging, including fluorodeoxyglucose (18F-FDG), 18F-sodium fluoride (18F-NaF), 11C-acetate, 11C/18F choline (9-12), and 18F-FACBC (fluciclovine) (13), each with their advantages over standard imaging, but with ongoing limitations in terms of sensitivity and specificity (2,14).
Some of the most promising PET tracers are those that target prostate specific membrane antigen (PSMA). PSMA is a 100-kDa type II transmembrane zinc metalloenzyme receptor (15,16) expressed largely in the prostate and to a lesser degree in the duodenum, brain, salivary gland, kidney, colon, and the neovasculature of tumors including lung, colon, breast, and kidney cancers (17,18). While its biologic function in PC is unknown, PSMA expression is nearly 100-fold higher in the prostate than in other tissues and is 10-fold higher in PC than in healthy prostate tissue (2,15). Its expression is further increased in higher-grade and metastatic cancers, and on development of castration resistance (19). Unlike prostate-specific antigen (PSA) and prostatic acid phosphatase that are secreted into the circulation, PSMA is membrane bound (20). In addition, its cytoplasmic domain contains an internalization motif that results in clathrin-mediated endocytosis that increases the uptake and deposition of radiotracers into cells. This feature may allow for a higher signal to noise ratio, making it an attractive target for imaging (19).
Initial targeting of PSMA used monoclonal antibodies, with 111In-capromab pendetide (Prostascint) being the only FDA approved agent for PSMA imaging (21). However, it targets the cytoplasmic domain of the receptor and is thus only taken up by dying cells with disrupted membranes. As it is also excreted into the bowel and bladder, interpretation of scans, especially in the retroperitoneum where PC often metastasizes is limited by a poor positive predictive value (PPV) and specificity (22). Another antibody, J915, targets the extracellular domain of PSMA. Chelated with DOTA as a linker for radiotracers, it can be linked to 89Zr, 64Cu, 90Y, 177Lu, making it an agent that can be used both for imaging and targeted radionuclide therapy (23). However, one significant limitation of antibody-based imaging includes the long half-life of antibodies that results in high background signal and poor penetration into tumors (2).
Newer PSMA tracers are small molecule inhibitors targeting the extracellular portion of the molecule. These agents can be labeled with radioisotopes including 123I, 18F, 111In, and 68Ga. Because of their size, these molecules exhibit rapid extravasation, diffusion into extracellular spaces, and blood clearance allowing for a higher tumor-to-background signal within 1–2 hours after injection. These agents can be grouped into three different classes: those that are urea-based, thiol based, or phosphorous based (24). One of the most studied and widely used compounds is the urea-based Glu-NH-CO-NH-Lys(Ahx)-HBED-CC (68Ga-PSMA-HBED-CC, or 68Ga-PSMA) initially described by Eder et al. (25). Urea based 18F compounds have also demonstrated favorable imaging characteristics. N-[N-[(S)-1,3-dicarboxypropyl] carbamoyl]-4-[18F]fluorobenzyl-L-cysteine (18F-DCFBC), a first generation molecule developed at Johns Hopkins (26), has been followed by a second generation compound, 2-(3-(1-carboxy-5-[(6-[18F]fluoro-pyridine-3-carbonyl)-amino]-pentyl)-ureido)-pentanedioic acid ([18F]DCFPyL), that demonstrates increased sensitivity and specificity for PC (27,28). These targeted imaging molecules have great promise for PC detection and management, and their application in different clinical scenarios will be reviewed here.
Local tumor detection
Not all men diagnosed with localized PC will die of the disease or even develop symptoms (29,30). Determining who requires therapy is critical because treatments, such as surgery or radiation, can result in long-term side effects including urinary incontinence and erectile dysfunction (31). However, unlike other early stage solid tumors that are diagnosed on biopsy of a lesion detected on imaging, PC is diagnosed by random sampling of the entire gland. This can lead to misclassification of a patient’s initial disease stage or grade, both of which are necessary to guide appropriate management. The possibility of not sampling a clinically significant lesion is 10–46% (32), and up to 36% of PCs on biopsy are upgraded or upstaged at the time of surgery (33). As a result, an estimated 23–42% of men with PC are over-treated (34), leading to unnecessary complications and healthcare costs.
Multiparametric MRI (mpMRI) combines conventional T1 and T2 weighted images with functional MRI sequences such as diffusion weighted imaging, dynamic contrast enhancement, and MR spectroscopy, and has an improved ability over conventional imaging to detect localized PC (35-37). Targeting tumors for biopsy using mpMRI can improve the detection of clinically significant disease and reduce the detection of lower risk disease (38-42). However, in a retrospective analysis of mpMRI compared to tumor histology at the time of prostatectomy, the average sensitivity of detecting an index lesion was only 60.2% across six radiologists (43). In a prospective cohort study of 1,003 men undergoing both MRI-targeted and standard random biopsies, targeted biopsies detected 30% more high-risk cancers and 17% fewer low risk cancers (43,44). However, 15% of cases had higher risk pathology found only on standard biopsy, and in the subset of patients with no prior biopsies there was no difference between targeted and standard biopsies. Thus, with a sensitivity of 58–97% and specificity of 23–87% to detect clinically significant PC additional improvements are needed for mpMRI (38,45-47).
PSMA-PET has also been studied to detect localized PC (3,48-58). Rowe et al. prospectively evaluated 13 patients with 18F-DCFBC PET and MRI prior to prostatectomy then correlated 18F-DCFBC PET to histopathology on a per-segment (12 regions) basis. They found that for detection of PC 18F-DCFBC PET had a sensitivity of 17% while that for MRI was 39%, however 18F-DCFBC PET had a specificity of 96% compared to 89% for MRI (3). Interestingly, 18F-DCFBC uptake was associated with Gleason score (ρ=0.64) and 18F-DCFBC uptake was significantly lower in benign prostatic hypertrophy than primary tumors (median SUV 2.2 vs. 3.5, P=0.004). Similarly, Turkbey et al. evaluated 13 patients with both 18F-DCFBC PET/CT and MRI and correlated these findings to histopathology either from MRI-fusion biopsies (n=9) or prostatectomy (n=4) (59). They found that sensitivity to detect the largest and highest Gleason lesion for 18F-DCFBC and MRI were 61.5% and 92%, respectively.
68Ga-PSMA has a higher tumor-to-background signal ratio compared to 18F-DCFBC (55), and studies evaluating this tracer demonstrate higher sensitivities and specificities for detecting disease. Rahbar et al. prospectively evaluated 68Ga-PSMA PET in six patients who underwent prostatectomy for Gleason ≥3+4 tumors (55). They found that 68Ga-PSMA PET had a sensitivity and specificity of 92% for identifying areas of PC. Fendler et al. also prospectively evaluated 68Ga-PSMA PET in 21 patients with biopsy proven PC who underwent prostatectomy for Gleason 7 tumors (50). In this study, the prostate was sectioned based on a six-segment model. The sensitivity and specificity of 68Ga-PMSA PET to detect cancer were 67% and 92%, respectively. False-negative results were noted in 6 of 12 segments with a Gleason score (GS) of 6, 12 of 27 segments with a GS of 7, 4 of 19 segments with a GS of 8, 10 of 41 segments with a GS of 9, and one segment with a GS of 10. Rhee et al. compared mpMRI and 68Ga-PSMA PET in men scheduled to undergo radical prostatectomy (51). They compared the imaging results to whole mount specimens and found that the sensitivity, specificity, PPV, and negative predictive value (NPV) for mpMRI to be 44%, 94%, 81%, 76%, respectively, while the same values for 68Ga-PSMA PET were 49%, 95%, 85%, 88%.
Determining the accuracy of an imaging modality to detect PC requires accurate registration between imaging and histopathology as the distribution of PC within the gland can be heterogeneous and deformations can occur during surgery and the histopathologic work up (45). Zamboglou et al. addressed this problem by conducting a prospective study of 9 men who went 68Ga-PSMA PET/CT scans prior to prostatectomy. They meticulously performed co-registration studies between 68Ga-PSMA scans and histopathology and found a statistically significant correlation of 68Ga-PSMA PET/CT with histopathology in eight subjects and an average ROC AUC of 0.82 (48). A follow up study evaluated 10 men undergoing prostatectomy and compared 68Ga-PMSA PET/CT and mpMRI to co-registered histopathology (60). They found that sensitivity and specificity for PC detected by 68Ga-PMSA PET/CT and mpMRI were 75% and 87%, and 70% and 82%, respectively. They further found that the union of 68Ga-PMSA PET/CT with mpMRI had a sensitivity and specificity of 82% and 67%, respectively, while that for the intersection of PSMA-PET and mpMRI were 55% and 99%, respectively.
Combining 68Ga-PMSA PET and MRI has also been studied to evaluate whether additional multimodal imaging might improve the diagnostic performance. Using an integrated PET/MRI system, Eiber et al. performed simultaneous 68Ga-PMSA PET/MRI on 66 patients with intermediate or high risk PC prior to prostatectomy (52). MRIs were read separately from 68Ga-PMSA PET scans by different radiologists then together by a dual-board certified radiologist. Prostatectomy specimens were divided into sextants and the presence of tumor, size, and Gleason grade were noted for comparison to scans. Sensitivity and specificity for tumor detection were 43% and 95%, respectively for MRI, 64% and 94%, respectively for 68Ga-PMSA PET, and 76% and 97%, respectively for combination 68Ga-PMSA PET/MRI.
One problem with these initial studies to locate primary tumors in PC using 68Ga-PMSA PET scans is that they were all conducted in patients who were candidates for treatment of clinically significant PC. The performance of this imaging modality may eventually need to be tested in a population undergoing screening for PC where not all men will be diagnosed with the disease.
Lymph node metastases
The presence of lymph node metastases at initial diagnosis is thought to represent systemic disease and signifies a poorer prognosis often necessitating a change in clinical management (61-63). Determining whether lymph nodes contain PC is challenging as standard imaging modalities rely on size and shape criteria. Specifically, round nodes larger than 8 mm in diameter or oval nodes with the short axis length greater than 10mm are considered metastatic. However, metastases can often be found in lymph nodes smaller than 8 mm in diameter resulting in a sensitivity of 36–40% using standard imaging methods (64).
PSMA-PET scans have been used to evaluate the presence of nodal metastases with varying success. Budäus et al. performed a retrospective analysis of 30 men with a nomogram-calculated risk of lymph node metastases greater than 20% who had undergone a 68Ga-PMSA PET prior to prostatectomy and extended pelvic lymph node dissection (65). The overall sensitivity, specificity, PPV, and NPV of 68Ga-PMSA PET/CT to detect lymph node metastases were 33.3%, 100%, 100%, and 69.2%, respectively. They found that smaller intranodal tumor deposits were missed. The median size of 68Ga-PMSA PET detected lymph node metastases was 13.6 mm compared to that of those missed measuring 4.3 mm.
The largest study to evaluate the ability of 68Ga-PMSA PET to detect lymph node metastases reviewed scans and pathology of 130 men who were imaged with either a 68Ga-PMSA PET/CT (n=95) or 68Ga-PMSA PET/MRI (n=25) then underwent prostatectomy and standard template lymph node dissection (66). The sensitivity, specificity, and accuracy for standard imaging (MRI or CT) for nodal metastases were 43.9%, 85.4%, and 72.9%, respectively, while those for 68Ga-PMSA PET were 65.9%, 98.9%, and 88.5%.
Another retrospective study of 42 men evaluated with mpMRI and 68Ga-PMSA PET/CT prior to prostatectomy and pelvic lymph node dissection noted a patient based sensitivity, specificity, PPV, and NPV for nodal metastases detected by 68Ga-PMSA PET of 93.33%, 96.30%, 93.33% and 96.30%, respectively (67). MRI had similar results for detecting nodal metastases with a patient-based sensitivity, specificity, PPV and NPV of 93.33%, 96.30%, 87.5% and 96.15%, respectively. These results might be explained by the larger lymph node sizes in this patient population with a mean size of 28.87 mm (range, 16–45 mm).
Biochemical recurrence
Within 10 years after curative therapy for localized PC, as many as 20–40% of patients will develop recurrent disease often in the form of a rising PSA (45). Also known as biochemical recurrence (BCR), this often precedes the appearance of clinical metastases by about 8 years (68). Whether BCR represents a local recurrence in the prostate or prostatectomy bed, or new metastatic disease is unclear as current standard imaging modalities are often unable to detect the presence of disease at these low PSA levels (4,69-71). As a result, management of BCR is controversial with recommendations including observation until the time of metastatic progression, early initiation of androgen deprivation therapy, participation on clinical trial, local ablation, or administration of salvage pelvic irradiation (4,5,72,73). As localization of recurrent disease is limited, salvage radiation plans have delivered varying doses to the prostatic and seminal vesicle bed and periprostatic tissues, and may or may not include the pelvic lymph nodes (72,74). And while this strategy may be effective in curing or delaying the need for systemic therapy, success rates remain poor at 10–40% (69).
Detecting the presence of malignancy in the setting of BCR after prostatectomy has been perhaps the most active area of research for PSMA-PET imaging. Numerous retrospective studies demonstrate the increased ability to detect suspicious lesions using PSMA-PET over standard imaging (12,75-84). Many of these studies evaluated cohorts ranging from 35 to 393 patients and focused on 68Ga-PMSA PET, reporting the detection of suspicious lesions in 74.2–91.4% of their patients, significantly higher numbers when compared to conventional imaging (12,75-78,80,82). Various factors have been studied and found associated with increased PSMA-PET detection, the most reliable being an increased serum PSA level (12,75-77,82). While some have noted increased detection with a faster PSA velocity (12,76) and shorter doubling time (77), others have found no association with PSA doubling time (12,75) or Gleason score (75,82).
Bluemel et al. evaluated 139 patients with BCR who first underwent an 18F-choline PET/CT (76). If that scan showed no evidence of malignancy, a 68Ga-PSMA PET/CT was offered. Of the 41 patients with a negative 18F-choline PET/CT, 32 agreed to the 68Ga-PSMA PET/CT, 14 (43.8%) of whom were found to have uptake on the second scan suggesting that 68Ga-PSMA PET/CT is a more sensitive test compared to 18F-choline PET/CT. Schwenck et al. performed a similar study of 103 patients with BCR and evaluated all with 11C-choline PET/CT followed by a 68Ga-PSMA PET/CT 24 hours later (53). While 55% of the 458 lymph nodes suspicious for metastases were detected with both imaging modalities, 39% were seen only with 68Ga-PSMA and tended to be smaller (6 vs. 11.7 mm). Interestingly, though 68Ga-PSMA appeared to be more sensitive, 6% of the lymph nodes showed only 11C-choline uptake.
Urinary excretion and accumulation of 68Ga-PSMA in the bladder may obscure small pelvic lymph nodes involved with disease. Freitag et al. evaluated the utility of adding mpMRI to 68Ga-PSMA PET/CT or 68Ga-PSMA PET/MRI to detect local recurrences in 119 patients with BCR (78). The team measured the effect of urinary bladder proximity on the local recurrence detection rate and found that eight local recurrences were found on mpMRI and not on 68Ga-PSMA PET scans. These local recurrences were within 1.3cm of the bladder and were not determined to be positive because of the high bladder SUV suggesting that there may be a role to combine PET with mpMRI. Uprimny et al. also addressed this issue evaluating local recurrences in 203 patients with BCR (84). Subjects were imaged within minutes of 68Ga-PSMA injection then again one hour after injection to test the hypothesis that with less bladder accumulation of radiotracer local recurrences might be more easily detected. Indeed they found that there was an increased detection rate for local recurrences within the pelvis of 24.6% and fewer equivocal lesions noted at the early time point compared to 12.8% at one hour after injection.
While the above studies demonstrate compelling data, only a handful included confirmation of disease status by histology or other clinical measures. Afshar-Oromieh et al. evaluated 319 patients with 68Ga-PSMA PET/CT (75). Histologic verification was performed on 42 patients and on a lesion based analysis, sensitivity, specificity, PPV and NPV were 76.6%, 100%, 100%, and 91.4%, respectively. Hijazi et al. retrospectively evaluated the ability of 68Ga-PMSA PET to detect lymph node metastases in 35 patients who underwent pelvic lymph node dissection either for biochemical recurrence (n=23) or for high-risk PC (n=12) (80). They found that for this cohort, 68Ga-PMSA PET had a sensitivity, specificity, PPV, and NPV to detect lymph node metastases of 94%, 99%, 89%, and 99.5%, respectively.
With their increased sensitivity to detect local recurrences, PSMA PET scans may impact therapy. Schiller et al. selected 31 patients who had developed BCR after prostatectomy and were found to have lymph node involvement on 68Ga-PSMA PET/CT or 68Ga-PSMA PET/MRI. Of these patients, 21 had suspicious lesions found only on 68Ga-PSMA PET imaging. Of the 28 nodes seen exclusively on 68Ga-PSMA PET, 15 were outside of the standard lymph drainage radiation field, a concerning clinical implication. As a result of these data, 13 patients received a boost to a subarea of the prostate bed they would otherwise not have received with standard imaging, and 18 patients received radiation to uncommon lymph node sites. Similarly, van Leeuwen et al. reported 70 patients evaluated with 68Ga-PMSA PET for BCR and found that the additional imaging changed salvage radiation therapy plans for 28.6% of patients (85). Eiber et al. evaluated 248 prostatectomy patients who had undergone 68Ga-PMSA PET for BCR (12). Of these patients 35 had selective radiation to PSMA-positive lesions and experienced a PSA decline, clinically indicating disease involvement in those areas. In a retrospective study by Afshar-Oromieh et al. 50 subjects received targeted therapy as local therapy for their PSMA detected lesions (75). Twenty-seven received external beam radiation, and 17 with follow up data all had a decline in their PSA; 19 had surgery and 4 were treated with high intensity focused ultrasound, all with documented declines in their PSA. While these studies are compelling, well-designed prospective clinical trials and long-term follow up are needed to understand the true risks and benefits of PSMA-PET in this setting.
Metastatic disease
The standard treatment for metastatic PC includes the use of systemic therapies (86,87). Oligometastatic disease, often defined as five or fewer metastatic lesions, is thought to be an intermediate step between localized and widespread metastatic disease. There is an increasing interest to treat these limited metastatic lesions with local ablative methods with the hope that doing so may interrupt the natural progression of disease and offer improved outcomes (5,88,89). This strategy is predicated on the ability to accurately identify lesions, both to appropriately classify patients and to target lesions for treatment. Given the improved imaging of PSMA PET over standard CT, MRI, or 99mTm-bone scan, a number of groups have published on the treatment of oligometastatic PC detected with this imaging modality.
A case report by Schiavina et al. described a patient with BCR found to have three lymph nodes involved on 68Ga-PMSA PET (90). An open lymph node dissection was performed and his PSA declined to <0.2 ng/mL and remained stable two months later. Of note, while metastatic disease was confirmed histologically in the three nodes found on imaging, another nine removed were also found to be involved, suggesting a concerning high false negative rate. Longer-term follow up was not provided and would be instructive. Maurer et al. presented a case series of 5 patients who underwent PSMA-radioguided surgery for metastatic PC (91). Lymph node metastases were identified with 68Ga-PMSA PET, and using an 111In labeled PSMA radiotracer and a gamma probe, metastatic lymph nodes were located intraoperatively. Interestingly, smaller tumor deposits in neighboring lymph nodes not seen on 68Ga-PMSA PET were detected with the gamma probe intraoperatively and confirmed histologically. While the team demonstrated the feasibility of this procedure with few complications, no response or follow up data were presented.
Larger case series have noted variable long-term outcomes. A series of 29 patients with BCR and metastases found using 68Ga-PMSA PET/CT was presented by Henkenberens et al. (92). The disease sites included local recurrences (13.8%), isolated lymph node metastases (58.6%), isolated bone metastases (20.7%), and one with a single pelvic node and vertebral body metastasis, all of which were treated with radiotherapy and had PSA declines. There were two recurrences, 12 and 12.7 months later, outside the radiation fields suggesting good disease control. Casamassima et al. detected metastatic recurrences using 11C- or 18F-choline as a radiotracer, their cohort of patients had 3 years of survival follow up (93). They found 25 patients with node only metastases who were treated with radiotherapy. Progression of disease was noted in 10 patients, two developed bone metastases within 60 days and eight had lymph node recurrences outside the irradiated areas. This translated to a 17% disease free survival and 92% overall survival at 3 years. The poor disease free survival may be due to the less sensitive imaging technique used to detect metastatic disease, however later studies also note high recurrence rates even with 68Ga-PMSA PET detection of disease.
Guler et al. evaluated 23 patients with BCR, 13 had castration sensitive and 10 had castration resistant disease, and were found to have 3 or fewer metastatic sites seen on 68Ga-PMSA PET (94). These lesions were treated with radiation and at a median follow up of 7 months, 19 patients (83%) had no recurrence of disease, however the actuarial one-year progression free survival was 51%. Habl et al. evaluated 15 patients who were treated with radiation therapy for 20 lesions found on either 68Ga-PMSA PET/CT or 11C-choline PET/CT (95). With a median follow up after radiation therapy of 22.5 months, the median PSA progression free survival was only 6.9 months and the median distant progression free survival was 7.36 months. Siriwardana et al. performed robot assisted salvage lymphadenectomy on 35 patients who had 58 lesions detected on 68Ga-PMSA PET/CT (96). A total of 32 patients had histopathologically proven metastatic disease with 87 lymph node metastases found at the time of surgery. A treatment response (PSA decline) was seen only in 43% of patients and PSA progression free survival at a median follow up of 12 months was only 23%. Similar to the case report by Schiavina et al. this study found more lymph nodes involved with disease than seen with 68Ga-PMSA PET/CT suggesting a lower sensitivity than previously thought and may explain the low response rates and poor recurrence free survival in this cohort. Again, prospectively designed clinical trials are needed to fully understand the utility of PSMA PET scans in this clinical setting (Table 1).
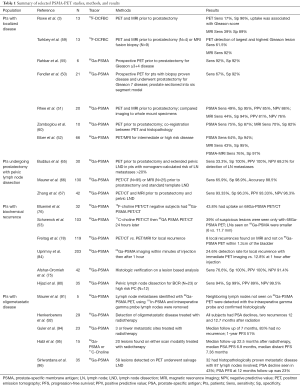
Full table
Future directions
Though 68Ga-PMSA PET demonstrates superior imaging qualities compared to other PET radiotracers (11), it has limitations in sensitivity and specificity. Newer PET tracers targeting PSMA are thus being developed. Particularly promising is a second generation small molecule inhibitor of PSMA, 2-(3-(1-carboxy-5-[(6-[18F]fluoro-pyridine-3-carbonyl)-amino]-pentyl)-ureido)-pentanedioic acid (18F-DCFPyL) (27). It demonstrates a higher tumor to background uptake allowing it to detect significantly more metastatic lesions compared to conventional imaging modalities (139 vs. 45) (97), and has more favorable imaging characteristics compared to 68Ga-PMSA PET/CT (98). In preliminary reports 18F-DCFPyL demonstrated the ability to detect intraprostatic tumors with good correlation to whole mount specimens (99). A larger study of 25 men with high or very high risk PC imaged with 18F-DCFPyL prior to prostatectomy and pelvic lymph node dissection demonstrated a sensitivity of 71.4% and specificity of 88.9% for detecting lymph node metastases (100).
The characteristics that make PSMA a good imaging target, particularly its specificity for PC and that ligands are internalized by clathrin mediated endocytosis, also allow it to be a target for delivery of radiopharmaceuticals, also known as endoradiotherapy. Several agents that target PSMA with bound radionuclide have been developed. Most of these agents use beta particle emitters including 177lutecium (177Lu), 90yittrium (90Y), and 131iodine (131I) (28), however agents using alpha particle emitter 225actinium (225Ac) are also being developed (101).
One of the first endoradiotherapeutic agents to be developed and studied was 177Lu-labeled J951 (102,103). In a phase II study of 47 patients with metastatic castration resistant prostate cancer (mCRPC), 55.3% of whom had received chemotherapy, 59.6% experienced a PSA decline with 36.2% having a ≥30% PSA decline and 10.6% having a ≥50% PSA decline (102). Toxicities included grade 4 thrombocytopenia in 46.8% (29.8% requiring transfusions) and grade 4 neutropenia in 25.5% (one patient with febrile neutropenia). Another early endoradiotherapeutic agent targeting PSMA was (S)-2-(3-((S)-1-Carboxy-5-(3-(4-[131I] iodophenyl)ureido)pentyl)ureido) pentanedioic acid, 131I-MIP-1095, developed by Molecular Insight Pharmaceuticals (104). Tested in 34 men with mCRPC, 94.1% had a PSA decline with 70.6% having a ≥50% PSA decline (105). Of the 16 patients who had pain prior to therapy, 15 experienced a reduction in pain. Twenty-three patients received a second dose, and three patients received a third dose at the time of PSA progression if they had had an initial response, however responses to subsequent doses were less effective. Hematologic toxicities after the first dose included grade 3 thrombocytopenia in 5.9% and grade 3 leukopenia in 2.9%, while non-hematologic toxicities included grade 1 or 2 xerostomia in 88.2% and fatigue in 5.9%. Despite the favorable efficacy and side effect profile of 131I-MIP-1095, excitement has not been as robust as radioiodinated pharmaceuticals have poor in vivo stability due to dehalogenation (23,106).
DOTAGA-(I-y)fk(Sub-KuE), termed PSMA I&T (for Imaging and Therapy), was developed by Weineisen et al. (107). It is a PSMA targeting endoradiotherapeutic agent that can be tagged with 68Ga or 177Lu for imaging or endoradiotherapy, respectively. In a preliminary study imaging one patient with 68Ga-PSMA I&T, uptake was seen in the primary tumor, regional lymph nodes, and bones with a lesion-to-background ratio of 17.6 for lymph node and 35.2 for bone metastases. One patient treated with 177Lu-PSMA I&T had a decline in PSA from 40.2 to 0.7 ng/mL within 3 months of the first dose with a concomitant improvement in pain symptoms (107). In a follow up report of 56 patients with mCRPC treated with 177Lu-PSMA I&T, 80.4% had a decline in PSA with 66.1% having a ≥30% PSA decline and 58.9% having a ≥50% PSA decline (108). The agent was well tolerated with grade 1 or 2 leukopenia in 9 patients but no other significant hematologic toxicities and only mild xerostomia in 2 patients. As a result 40 patients were able to receive more than one cycle of therapy. The median progression free survival was 13.7 months and median overall survival was not reached in the 28 months of follow up.
PSMA DKFZ-617 is another small molecule inhibitor of PSMA that can bind to both 68Ga and 177Lu for imaging and therapy. In an initial study of 10 patients, 70% had a decline in PSA with 5 having a ≥50% PSA decline and only one patient having a grade 3 anemia (109). A follow up report of 22 patients treated with at least two cycles of 177Lu-PSMA DKFZ-617 showed that PSA responses were obtained in 79.1% after the first dose and 68.2% after the second dose with only two cases of grade 3 anemia (110). Alpha emitter 225Ac has also been linked to PSMA DKFZ-617 with the thought that alpha radiation therapy may effectively treat disease that is resistant to beta emitters (101). In their initial report, Kratochwil et al. documented the administration of 225Ac-PSMA DKFZ-617 in two heavily pre-treated patients, one with diffuse marrow infiltration and contraindicated to beta emitter therapy, and another who had disease progression on 177Lu-PSMA DKFZ-617 with peritoneal carcinomatosis and liver infiltration (101). Quite remarkably, both patients had a decline in PSA to undetectable levels and a complete response on imaging with xerostomia as the only clinical side effect. The group later reported follow-up on 14 heavily pre-treated patients with poor risk features including ten patients with visceral metastases (111). Astonishingly nine of the 11 evaluable patients had either a radiographic response or PSA decline with therapy, however severe xerostomia did become a dose limiting toxicity (111).
PSMA imaging and targeting is an active field of research with at least 40 trials currently open and recruiting subjects on ClinicalTrials.gov, including NCT03042468 and NCT03042312, which further evaluate the use of 177Lu-PSMA DKFZ-617, and NCT02552394, which explores additional applications of J591. Others combine this exciting field with the burgeoning field of immunotherapy such as NCT03089203, a phase I study of an intravenously administered dual PSMA-specific/TFGβ chimeric antigen receptor modified autologous T cell in patients with mCRPC, and NCT02262910, evaluating MOR209/ES414 (112), a bispecific antibody targeting PSMA and the T-cell receptor in patients with mCPRC.
Conclusions
The field of PC imaging has changed dramatically with the rapid development of PSMA-targeted imaging. With its increased ability to detect disease, the clearest application of this imaging modality appears to be in patients with biochemical recurrence when conventional imaging is unable to identify disease at very low PSA levels. Other areas where PSMA imaging appears promising is the detection and characterization of primary tumors, local staging of regional lymph nodes, and the identification of oligometastatic disease to allow for targeted treatment. PSMA-targeted treatment with endoradiotherapeutic agents is another emerging field. Results from preliminary reports with dramatic response rates and often tolerable side effects demonstrate the promise of targeting PSMA with radioligands. While results of many of these studies are compelling, they are also limited as they are largely retrospective and descriptive without clear controls, making it difficult to determine whether these agents affect outcomes. The true potential of this field can be better realized with well-designed prospective clinical trials.
Acknowledgments
Funding: None.
Footnote
Provenance and Peer Review: This article was commissioned by the Guest Editors (Israel Deutsch, James McKiernan, Charles Drake) for the series “Prostate Cancer: Current Understanding and Future Directions” published in Translational Cancer Research. The article has undergone external peer review.
Conflicts of Interest: The author has completed the ICMJE uniform disclosure form (available at http://dx.doi.org/10.21037/tcr.2018.07.04). The series “Prostate Cancer: Current Understanding and Future Directions” was commissioned by the editorial office without any funding or sponsorship. The author has no conflicts of interest to declare.
Ethical Statement: The author is accountable for all aspects of the work in ensuring that questions related to the accuracy or integrity of any part of the work are appropriately investigated and resolved.
Open Access Statement: This is an Open Access article distributed in accordance with the Creative Commons Attribution-NonCommercial-NoDerivs 4.0 International License (CC BY-NC-ND 4.0), which permits the non-commercial replication and distribution of the article with the strict proviso that no changes or edits are made and the original work is properly cited (including links to both the formal publication through the relevant DOI and the license). See: https://creativecommons.org/licenses/by-nc-nd/4.0/.
References
- Siegel RL, Miller KD, Jemal A. Cancer Statistics, 2017. CA Cancer J Clin 2017;67:7-30. [Crossref] [PubMed]
- Lindenberg L, Choyke P, Dahut W. Prostate Cancer Imaging with Novel PET Tracers. Curr Urol Rep 2016;17:18. [Crossref] [PubMed]
- Rowe SP, Gage KL, Faraj SF, et al. (1)(8)F-DCFBC PET/CT for PSMA-Based Detection and Characterization of Primary Prostate Cancer. J Nucl Med 2015;56:1003-10. [Crossref] [PubMed]
- Kosuri S, Akhtar NH, Smith M, et al. Review of salvage therapy for biochemically recurrent prostate cancer: the role of imaging and rationale for systemic salvage targeted anti-prostate-specific membrane antigen radioimmunotherapy. Adv Urol 2012;2012:921674 [Crossref] [PubMed]
- Yao HH, Hong M, Corcoran NM, et al. Advances in local and ablative treatment of oligometastasis in prostate cancer. Asia Pac J Clin Oncol 2014;10:308-21. [Crossref] [PubMed]
- Cook GJ, Azad G, Padhani AR. Bone imaging in prostate cancer: the evolving roles of nuclear medicine and radiology. Clin Transl Imaging 2016;4:439-47. [Crossref] [PubMed]
- Scher HI, Morris MJ, Stadler WM, et al. Trial Design and Objectives for Castration-Resistant Prostate Cancer: Updated Recommendations From the Prostate Cancer Clinical Trials Working Group 3. J Clin Oncol 2016;34:1402-18. [Crossref] [PubMed]
- Scher HI, Halabi S, Tannock I, et al. Design and end points of clinical trials for patients with progressive prostate cancer and castrate levels of testosterone: recommendations of the Prostate Cancer Clinical Trials Working Group. J Clin Oncol 2008;26:1148-59. [Crossref] [PubMed]
- Souvatzoglou M, Weirich G, Schwarzenboeck S, et al. The sensitivity of [11C]choline PET/CT to localize prostate cancer depends on the tumor configuration. Clin Cancer Res 2011;17:3751-9. [Crossref] [PubMed]
- Farsad M, Schiavina R, Castellucci P, et al. Detection and localization of prostate cancer: correlation of (11)C-choline PET/CT with histopathologic step-section analysis. J Nucl Med 2005;46:1642-9. [PubMed]
- Afshar-Oromieh A, Zechmann CM, Malcher A, et al. Comparison of PET imaging with a (68)Ga-labelled PSMA ligand and (18)F-choline-based PET/CT for the diagnosis of recurrent prostate cancer. Eur J Nucl Med Mol Imaging 2014;41:11-20. [Crossref] [PubMed]
- Eiber M, Maurer T, Souvatzoglou M, et al. Evaluation of Hybrid (6)(8)Ga-PSMA Ligand PET/CT in 248 Patients with Biochemical Recurrence After Radical Prostatectomy. J Nucl Med 2015;56:668-74. [Crossref] [PubMed]
- Nanni C, Zanoni L, Pultrone C, et al. (18)F-FACBC (anti1-amino-3-(18)F-fluorocyclobutane-1-carboxylic acid) versus (11)C-choline PET/CT in prostate cancer relapse: results of a prospective trial. Eur J Nucl Med Mol Imaging 2016;43:1601-10. [Crossref] [PubMed]
- Jadvar H. Molecular imaging of prostate cancer: PET radiotracers. AJR Am J Roentgenol 2012;199:278-91. [Crossref] [PubMed]
- Meller B, Bremmer F, Sahlmann CO, et al. Alterations in androgen deprivation enhanced prostate-specific membrane antigen (PSMA) expression in prostate cancer cells as a target for diagnostics and therapy. EJNMMI Res 2015;5:66. [Crossref] [PubMed]
- Maurer T, Eiber M, Schwaiger M, et al. Current use of PSMA-PET in prostate cancer management. Nat Rev Urol 2016;13:226-35. [Crossref] [PubMed]
- O'Keefe DS, Su SL, Bacich DJ, et al. Mapping, genomic organization and promoter analysis of the human prostate-specific membrane antigen gene. Biochim Biophys Acta 1998;1443:113-27. [Crossref] [PubMed]
- Liu H, Moy P, Kim S, et al. Monoclonal antibodies to the extracellular domain of prostate-specific membrane antigen also react with tumor vascular endothelium. Cancer Res 1997;57:3629-34. [PubMed]
- Rajasekaran SA, Anilkumar G, Oshima E, et al. A novel cytoplasmic tail MXXXL motif mediates the internalization of prostate-specific membrane antigen. Mol Biol Cell 2003;14:4835-45. [Crossref] [PubMed]
- Elsasser-Beile U, Reischl G, Wiehr S, et al. PET imaging of prostate cancer xenografts with a highly specific antibody against the prostate-specific membrane antigen. J Nucl Med 2009;50:606-11. [Crossref] [PubMed]
- Taneja SS. ProstaScint(R) Scan: Contemporary Use in Clinical Practice. Rev Urol 2004;6:S19-28. [PubMed]
- Kelloff GJ, Choyke P, Coffey DS, et al. Challenges in clinical prostate cancer: role of imaging. AJR Am J Roentgenol 2009;192:1455-70. [Crossref] [PubMed]
- Tagawa ST, Beltran H, Vallabhajosula S, et al. Anti-prostate-specific membrane antigen-based radioimmunotherapy for prostate cancer. Cancer 2010;116:1075-83. [Crossref] [PubMed]
- Lutje S, Heskamp S, Cornelissen AS, et al. PSMA Ligands for Radionuclide Imaging and Therapy of Prostate Cancer: Clinical Status. Theranostics 2015;5:1388-401. [Crossref] [PubMed]
- Eder M, Schafer M, Bauder-Wust U, et al. 68Ga-complex lipophilicity and the targeting property of a urea-based PSMA inhibitor for PET imaging. Bioconjug Chem 2012;23:688-97. [Crossref] [PubMed]
- Mease RC, Dusich CL, Foss CA, et al. N-[N-[(S)-1,3-Dicarboxypropyl]carbamoyl]-4-[18F]fluorobenzyl-L-cysteine, [18F]DCFBC: a new imaging probe for prostate cancer. Clin Cancer Res 2008;14:3036-43. [Crossref] [PubMed]
- Szabo Z, Mena E, Rowe SP, et al. Initial Evaluation of [(18)F]DCFPyL for Prostate-Specific Membrane Antigen (PSMA)-Targeted PET Imaging of Prostate Cancer. Mol Imaging Biol 2015;17:565-74. [Crossref] [PubMed]
- Bouchelouche K, Turkbey B, Choyke PL. PSMA PET and Radionuclide Therapy in Prostate Cancer. Semin Nucl Med 2016;46:522-35. [Crossref] [PubMed]
- Holund B. Latent prostatic cancer in a consecutive autopsy series. Scand J Urol Nephrol 1980;14:29-35. [Crossref] [PubMed]
- Sakr WA, Grignon DJ, Crissman JD, et al. High grade prostatic intraepithelial neoplasia (HGPIN) and prostatic adenocarcinoma between the ages of 20-69: an autopsy study of 249 cases. In Vivo 1994;8:439-43. [PubMed]
- Sanda MG, Dunn RL, Michalski J, et al. Quality of life and satisfaction with outcome among prostate-cancer survivors. N Engl J Med 2008;358:1250-61. [Crossref] [PubMed]
- Shariat SF, Roehrborn CG. Using biopsy to detect prostate cancer. Rev Urol 2008;10:262-80. [PubMed]
- Cohen MS, Hanley RS, Kurteva T, et al. Comparing the Gleason prostate biopsy and Gleason prostatectomy grading system: the Lahey Clinic Medical Center experience and an international meta-analysis. Eur Urol 2008;54:371-81. [Crossref] [PubMed]
- Draisma G, Etzioni R, Tsodikov A, et al. Lead time and overdiagnosis in prostate-specific antigen screening: importance of methods and context. J Natl Cancer Inst 2009;101:374-83. [Crossref] [PubMed]
- Haider MA, van der Kwast TH, Tanguay J, et al. Combined T2-weighted and diffusion-weighted MRI for localization of prostate cancer. AJR Am J Roentgenol 2007;189:323-8. [Crossref] [PubMed]
- Turkbey B, Mani H, Shah V, et al. Multiparametric 3T prostate magnetic resonance imaging to detect cancer: histopathological correlation using prostatectomy specimens processed in customized magnetic resonance imaging based molds. J Urol 2011;186:1818-24. [Crossref] [PubMed]
- Kitajima K, Kaji Y, Fukabori Y, et al. Prostate cancer detection with 3 T MRI: comparison of diffusion-weighted imaging and dynamic contrast-enhanced MRI in combination with T2-weighted imaging. J Magn Reson Imaging 2010;31:625-31. [Crossref] [PubMed]
- Scheenen TW, Rosenkrantz AB, Haider MA, et al. Multiparametric Magnetic Resonance Imaging in Prostate Cancer Management: Current Status and Future Perspectives. Invest Radiol 2015;50:594-600. [Crossref] [PubMed]
- Haffner J, Lemaitre L, Puech P, et al. Role of magnetic resonance imaging before initial biopsy: comparison of magnetic resonance imaging-targeted and systematic biopsy for significant prostate cancer detection. BJU Int 2011;108:E171-8. [Crossref] [PubMed]
- Moore CM, Robertson NL, Arsanious N, et al. Image-guided prostate biopsy using magnetic resonance imaging-derived targets: a systematic review. Eur Urol 2013;63:125-40. [Crossref] [PubMed]
- Watanabe Y, Terai A, Araki T, et al. Detection and localization of prostate cancer with the targeted biopsy strategy based on ADC map: a prospective large-scale cohort study. J Magn Reson Imaging 2012;35:1414-21. [Crossref] [PubMed]
- Siddiqui MM, Rais-Bahrami S, Truong H, et al. Magnetic resonance imaging/ultrasound-fusion biopsy significantly upgrades prostate cancer versus systematic 12-core transrectal ultrasound biopsy. Eur Urol 2013;64:713-9. [Crossref] [PubMed]
- Rosenkrantz AB, Deng FM, Kim S, et al. Prostate cancer: multiparametric MRI for index lesion localization--a multiple-reader study. AJR Am J Roentgenol 2012;199:830-7. [Crossref] [PubMed]
- Siddiqui MM, Rais-Bahrami S, Turkbey B, et al. Comparison of MR/ultrasound fusion-guided biopsy with ultrasound-guided biopsy for the diagnosis of prostate cancer. JAMA 2015;313:390-7. [Crossref] [PubMed]
- Bailey J, Piert M. Performance of 68Ga-PSMA PET/CT for Prostate Cancer Management at Initial Staging and Time of Biochemical Recurrence. Curr Urol Rep 2017;18:84. [Crossref] [PubMed]
- Johnson LM, Turkbey B, Figg WD, et al. Multiparametric MRI in prostate cancer management. Nat Rev Clin Oncol 2014;11:346-53. [Crossref] [PubMed]
- Rosenkrantz AB, Ginocchio LA, Cornfeld D, et al. Interobserver Reproducibility of the PI-RADS Version 2 Lexicon: A Multicenter Study of Six Experienced Prostate Radiologists. Radiology 2016;280:793-804. [Crossref] [PubMed]
- Zamboglou C, Schiller F, Fechter T, et al. (68)Ga-HBED-CC-PSMA PET/CT Versus Histopathology in Primary Localized Prostate Cancer: A Voxel-Wise Comparison. Theranostics 2016;6:1619-28. [Crossref] [PubMed]
- Rauscher I, Maurer T, Fendler WP, et al. (68)Ga-PSMA ligand PET/CT in patients with prostate cancer: How we review and report. Cancer Imaging 2016;16:14. [Crossref] [PubMed]
- Fendler WP, Schmidt DF, Wenter V, et al. 68Ga-PSMA PET/CT Detects the Location and Extent of Primary Prostate Cancer. J Nucl Med 2016;57:1720-5. [Crossref] [PubMed]
- Rhee H, Thomas P, Shepherd B, et al. Prostate Specific Membrane Antigen Positron Emission Tomography May Improve the Diagnostic Accuracy of Multiparametric Magnetic Resonance Imaging in Localized Prostate Cancer. J Urol 2016;196:1261-7. [Crossref] [PubMed]
- Eiber M, Weirich G, Holzapfel K, et al. Simultaneous 68Ga-PSMA HBED-CC PET/MRI Improves the Localization of Primary Prostate Cancer. Eur Urol 2016;70:829-36. [Crossref] [PubMed]
- Schwenck J, Rempp H, Reischl G, et al. Comparison of 68Ga-labelled PSMA-11 and 11C-choline in the detection of prostate cancer metastases by PET/CT. Eur J Nucl Med Mol Imaging 2017;44:92-101. [Crossref] [PubMed]
- Sahlmann CO, Meller B, Bouter C, et al. Biphasic (6)(8)Ga-PSMA-HBED-CC-PET/CT in patients with recurrent and high-risk prostate carcinoma. Eur J Nucl Med Mol Imaging 2016;43:898-905. [Crossref] [PubMed]
- Rahbar K, Weckesser M, Huss S, et al. Correlation of Intraprostatic Tumor Extent with (6)(8)Ga-PSMA Distribution in Patients with Prostate Cancer. J Nucl Med 2016;57:563-7. [Crossref] [PubMed]
- Kabasakal L, Demirci E, Ocak M, et al. Evaluation of PSMA PET/CT imaging using a 68Ga-HBED-CC ligand in patients with prostate cancer and the value of early pelvic imaging. Nucl Med Commun 2015;36:582-7. [Crossref] [PubMed]
- Giesel FL, Sterzing F, Schlemmer HP, et al. Intra-individual comparison of (68)Ga-PSMA-11-PET/CT and multi-parametric MR for imaging of primary prostate cancer. Eur J Nucl Med Mol Imaging 2016;43:1400-6. [Crossref] [PubMed]
- Zamboglou C, Wieser G, Hennies S, et al. MRI versus (6)(8)Ga-PSMA PET/CT for gross tumour volume delineation in radiation treatment planning of primary prostate cancer. Eur J Nucl Med Mol Imaging 2016;43:889-97. [Crossref] [PubMed]
- Turkbey B, Mena E, Lindenberg L, et al. 18F-DCFBC Prostate-Specific Membrane Antigen-Targeted PET/CT Imaging in Localized Prostate Cancer: Correlation With Multiparametric MRI and Histopathology. Clin Nucl Med 2017;42:735-40. [Crossref] [PubMed]
- Zamboglou C, Drendel V, Jilg CA, et al. Comparison of 68Ga-HBED-CC PSMA-PET/CT and multiparametric MRI for gross tumour volume detection in patients with primary prostate cancer based on slice by slice comparison with histopathology. Theranostics 2017;7:228-37. [Crossref] [PubMed]
- Park SY, Oh YT, Jung DC, et al. Prediction of Micrometastasis (< 1 cm) to Pelvic Lymph Nodes in Prostate Cancer: Role of Preoperative MRI. AJR Am J Roentgenol 2015;205:W328-34 [Crossref] [PubMed]
- Messing EM, Manola J, Sarosdy M, et al. Immediate hormonal therapy compared with observation after radical prostatectomy and pelvic lymphadenectomy in men with node-positive prostate cancer. N Engl J Med 1999;341:1781-8. [Crossref] [PubMed]
- Abdollah F, Karnes RJ, Suardi N, et al. Impact of adjuvant radiotherapy on survival of patients with node-positive prostate cancer. J Clin Oncol 2014;32:3939-47. [Crossref] [PubMed]
- Heesakkers RA, Hovels AM, Jager GJ, et al. MRI with a lymph-node-specific contrast agent as an alternative to CT scan and lymph-node dissection in patients with prostate cancer: a prospective multicohort study. Lancet Oncol 2008;9:850-6. [Crossref] [PubMed]
- Budäus L, Leyh-Bannurah SR, Salomon G, et al. Initial Experience of (68)Ga-PSMA PET/CT Imaging in High-risk Prostate Cancer Patients Prior to Radical Prostatectomy. Eur Urol 2016;69:393-6. [Crossref] [PubMed]
- Maurer T, Gschwend JE, Rauscher I, et al. Diagnostic Efficacy of (68)Gallium-PSMA Positron Emission Tomography Compared to Conventional Imaging for Lymph Node Staging of 130 Consecutive Patients with Intermediate to High Risk Prostate Cancer. J Urol 2016;195:1436-43. [Crossref] [PubMed]
- Zhang Q, Zang S, Zhang C, et al. Comparison of 68Ga-PSMA-11 PET-CT with mpMRI for preoperative lymph node staging in patients with intermediate to high-risk prostate cancer. J Transl Med 2017;15:230. [Crossref] [PubMed]
- Antonarakis ES, Feng Z, Trock BJ, et al. The natural history of metastatic progression in men with prostate-specific antigen recurrence after radical prostatectomy: long-term follow-up. BJU Int 2012;109:32-9. [Crossref] [PubMed]
- Stephenson AJ, Scardino PT, Kattan MW, et al. Predicting the outcome of salvage radiation therapy for recurrent prostate cancer after radical prostatectomy. J Clin Oncol 2007;25:2035-41. [Crossref] [PubMed]
- Rouviere O. Imaging techniques for local recurrence of prostate cancer: for whom, why and how? Diagn Interv Imaging 2012;93:279-90. [Crossref] [PubMed]
- Moul JW. Prostate specific antigen only progression of prostate cancer. J Urol 2000;163:1632-42. [Crossref] [PubMed]
- Stephenson AJ, Shariat SF, Zelefsky MJ, et al. Salvage radiotherapy for recurrent prostate cancer after radical prostatectomy. JAMA 2004;291:1325-32. [Crossref] [PubMed]
- Trock BJ, Han M, Freedland SJ, et al. Prostate cancer-specific survival following salvage radiotherapy vs observation in men with biochemical recurrence after radical prostatectomy. JAMA 2008;299:2760-9. [Crossref] [PubMed]
- Stephenson AJ, Slawin KM, Bianco FJ Jr, et al. Perspectives on the natural history of recurrent prostate cancer after radical prostatectomy, based on the response to salvage radiotherapy. BJU Int 2004;94:1210-2. [Crossref] [PubMed]
- Afshar-Oromieh A, Avtzi E, Giesel FL, et al. The diagnostic value of PET/CT imaging with the (68)Ga-labelled PSMA ligand HBED-CC in the diagnosis of recurrent prostate cancer. Eur J Nucl Med Mol Imaging 2015;42:197-209. [Crossref] [PubMed]
- Bluemel C, Krebs M, Polat B, et al. 68Ga-PSMA-PET/CT in Patients With Biochemical Prostate Cancer Recurrence and Negative 18F-Choline-PET/CT. Clin Nucl Med 2016;41:515-21. [Crossref] [PubMed]
- Ceci F, Uprimny C, Nilica B, et al. (68)Ga-PSMA PET/CT for restaging recurrent prostate cancer: which factors are associated with PET/CT detection rate? Eur J Nucl Med Mol Imaging 2015;42:1284-94. [Crossref] [PubMed]
- Freitag MT, Radtke JP, Afshar-Oromieh A, et al. Local recurrence of prostate cancer after radical prostatectomy is at risk to be missed in 68Ga-PSMA-11-PET of PET/CT and PET/MRI: comparison with mpMRI integrated in simultaneous PET/MRI. Eur J Nucl Med Mol Imaging 2017;44:776-87. [Crossref] [PubMed]
- Giesel FL, Fiedler H, Stefanova M, et al. PSMA PET/CT with Glu-urea-Lys-(Ahx)-[(6)(8)Ga(HBED-CC)] versus 3D CT volumetric lymph node assessment in recurrent prostate cancer. Eur J Nucl Med Mol Imaging 2015;42:1794-800. [Crossref] [PubMed]
- Hijazi S, Meller B, Leitsmann C, et al. Pelvic lymph node dissection for nodal oligometastatic prostate cancer detected by 68Ga-PSMA-positron emission tomography/computerized tomography. Prostate 2015;75:1934-40. [Crossref] [PubMed]
- Mena E, Lindenberg ML, Shih JH, et al. Clinical impact of PSMA-based 18F-DCFBC PET/CT imaging in patients with biochemically recurrent prostate cancer after primary local therapy. Eur J Nucl Med Mol Imaging 2018;45:4-11. [Crossref] [PubMed]
- Sanli Y, Kuyumcu S, Sanli O, et al. Relationships between serum PSA levels, Gleason scores and results of 68Ga-PSMAPET/CT in patients with recurrent prostate cancer. Ann Nucl Med 2017;31:709-17. [Crossref] [PubMed]
- Schiller K, Sauter K, Dewes S, et al. Patterns of failure after radical prostatectomy in prostate cancer - implications for radiation therapy planning after 68Ga-PSMA-PET imaging. Eur J Nucl Med Mol Imaging 2017;44:1656-62. [Crossref] [PubMed]
- Uprimny C, Kroiss AS, Fritz J, et al. Early PET imaging with [68]Ga-PSMA-11 increases the detection rate of local recurrence in prostate cancer patients with biochemical recurrence. Eur J Nucl Med Mol Imaging 2017;44:1647-55. [Crossref] [PubMed]
- van Leeuwen PJ, Stricker P, Hruby G, et al. (68) Ga-PSMA has a high detection rate of prostate cancer recurrence outside the prostatic fossa in patients being considered for salvage radiation treatment. BJU Int 2016;117:732-9. [Crossref] [PubMed]
- Fizazi K, Tran N, Fein L, et al. Abiraterone plus Prednisone in Metastatic, Castration-Sensitive Prostate Cancer. N Engl J Med 2017;377:352-60. [Crossref] [PubMed]
- Sweeney CJ, Chen YH, Carducci M, et al. Chemohormonal Therapy in Metastatic Hormone-Sensitive Prostate Cancer. N Engl J Med 2015;373:737-46. [Crossref] [PubMed]
- Rowe SP, Mana-Ay M, Javadi MS, et al. PSMA-Based Detection of Prostate Cancer Bone Lesions With (1)(8)F-DCFPyL PET/CT: A Sensitive Alternative to ((9)(9)m)Tc-MDP Bone Scan and Na(1)(8)F PET/CT? Clin Genitourin Cancer 2016;14:e115-8. [Crossref] [PubMed]
- Hellman S, Weichselbaum RR. Oligometastases. J Clin Oncol 1995;13:8-10. [Crossref] [PubMed]
- Schiavina R, Ceci F, Romagnoli D, et al. (68)Ga-PSMA-PET/CT-Guided Salvage Retroperitoneal Lymph Node Dissection for Disease Relapse After Radical Prostatectomy for Prostate Cancer. Clin Genitourin Cancer 2015;13:e415-7. [Crossref] [PubMed]
- Maurer T, Weirich G, Schottelius M, et al. Prostate-specific membrane antigen-radioguided surgery for metastatic lymph nodes in prostate cancer. Eur Urol 2015;68:530-4. [Crossref] [PubMed]
- Henkenberens C, von Klot CA, Ross TL, et al. (68)Ga-PSMA ligand PET/CT-based radiotherapy in locally recurrent and recurrent oligometastatic prostate cancer: Early efficacy after primary therapy. Strahlenther Onkol 2016;192:431-9. [Crossref] [PubMed]
- Casamassima F, Masi L, Menichelli C, et al. Efficacy of eradicative radiotherapy for limited nodal metastases detected with choline PET scan in prostate cancer patients. Tumori 2011;97:49-55. [Crossref] [PubMed]
- Guler OC, Engels B, Onal C, et al. The feasibility of prostate-specific membrane antigen positron emission tomography(PSMA PET/CT)-guided radiotherapy in oligometastatic prostate cancer patients. Clin Transl Oncol 2018;20:484-90. [Crossref] [PubMed]
- Habl G, Straube C, Schiller K, et al. Oligometastases from prostate cancer: local treatment with stereotactic body radiotherapy (SBRT). BMC Cancer 2017;17:361. [Crossref] [PubMed]
- Siriwardana A, Thompson J, van Leeuwen PJ, et al. Initial multicentre experience of 68 gallium-PSMA PET/CT guided robot-assisted salvage lymphadenectomy: acceptable safety profile but oncological benefit appears limited. BJU Int 2017;120:673-81. [Crossref] [PubMed]
- Rowe SP, Macura KJ, Mena E, et al. PSMA-Based [(18)F]DCFPyL PET/CT Is Superior to Conventional Imaging for Lesion Detection in Patients with Metastatic Prostate Cancer. Mol Imaging Biol 2016;18:411-9. [Crossref] [PubMed]
- Dietlein M, Kobe C, Kuhnert G, et al. Comparison of [(18)F]DCFPyL and [ (68)Ga]Ga-PSMA-HBED-CC for PSMA-PET Imaging in Patients with Relapsed Prostate Cancer. Mol Imaging Biol 2015;17:575-84. [Crossref] [PubMed]
- Bauman G, Martin P, Thiessen JD, et al. [18F]-DCFPyL Positron Emission Tomography/Magnetic Resonance Imaging for Localization of Dominant Intraprostatic Foci: First Experience. Eur Urol Focus 2016. [Epub ahead of print].
- Gorin MA, Rowe SP, Patel HD, et al. Prostate Specific Membrane Antigen Targeted 18F-DCFPyL Positron Emission Tomography/Computerized Tomography for the Preoperative Staging of High Risk Prostate Cancer: Results of a Prospective, Phase II, Single Center Study. J Urol 2018;199:126-32. [Crossref] [PubMed]
- Kratochwil C, Bruchertseifer F, Giesel FL, et al. 225Ac-PSMA-617 for PSMA-Targeted alpha-Radiation Therapy of Metastatic Castration-Resistant Prostate Cancer. J Nucl Med 2016;57:1941-4. [Crossref] [PubMed]
- Tagawa ST, Milowsky MI, Morris M, et al. Phase II study of Lutetium-177-labeled anti-prostate-specific membrane antigen monoclonal antibody J591 for metastatic castration-resistant prostate cancer. Clin Cancer Res 2013;19:5182-91. [Crossref] [PubMed]
- Bander NH, Milowsky MI, Nanus DM, et al. Phase I trial of 177lutetium-labeled J591, a monoclonal antibody to prostate-specific membrane antigen, in patients with androgen-independent prostate cancer. J Clin Oncol 2005;23:4591-601. [Crossref] [PubMed]
- Zechmann CM, Afshar-Oromieh A, Armor T, et al. Radiation dosimetry and first therapy results with a (124)I/ (131)I-labeled small molecule (MIP-1095) targeting PSMA for prostate cancer therapy. Eur J Nucl Med Mol Imaging 2014;41:1280-92. [Crossref] [PubMed]
- Afshar-Oromieh A, Haberkorn U, Zechmann C, et al. Repeated PSMA-targeting radioligand therapy of metastatic prostate cancer with 131I-MIP-1095. Eur J Nucl Med Mol Imaging 2017;44:950-9. [Crossref] [PubMed]
- Cavina L, van der Born D, Klaren PHM, et al. Design of Radioiodinated Pharmaceuticals: Structural Features Affecting Metabolic Stability towards in Vivo Deiodination. European J Org Chem 2017;2017:3387-414. [Crossref] [PubMed]
- Weineisen M, Schottelius M, Simecek J, et al. 68Ga- and 177Lu-Labeled PSMA I&T: Optimization of a PSMA-Targeted Theranostic Concept and First Proof-of-Concept Human Studies. J Nucl Med 2015;56:1169-76. [Crossref] [PubMed]
- Baum RP, Kulkarni HR, Schuchardt C, et al. 177Lu-Labeled Prostate-Specific Membrane Antigen Radioligand Therapy of Metastatic Castration-Resistant Prostate Cancer: Safety and Efficacy. J Nucl Med 2016;57:1006-13. [Crossref] [PubMed]
- Ahmadzadehfar H, Rahbar K, Kurpig S, et al. Early side effects and first results of radioligand therapy with (177)Lu-DKFZ-617 PSMA of castrate-resistant metastatic prostate cancer: a two-centre study. EJNMMI Res 2015;5:114. [Crossref] [PubMed]
- Ahmadzadehfar H, Eppard E, Kurpig S, et al. Therapeutic response and side effects of repeated radioligand therapy with 177Lu-PSMA-DKFZ-617 of castrate-resistant metastatic prostate cancer. Oncotarget 2016;7:12477-88. [Crossref] [PubMed]
- Kratochwil C, Bruchertseifer F, Rathke H, et al. Targeted alpha-Therapy of Metastatic Castration-Resistant Prostate Cancer with 225Ac-PSMA-617: Dosimetry Estimate and Empiric Dose Finding. J Nucl Med 2017;58:1624-31. [Crossref] [PubMed]
- Hernandez-Hoyos G, Sewell T, Bader R, et al. MOR209/ES414, a Novel Bispecific Antibody Targeting PSMA for the Treatment of Metastatic Castration-Resistant Prostate Cancer. Mol Cancer Ther 2016;15:2155-65. [Crossref] [PubMed]