P53 introduction enhances chemotherapy-induced apoptosis in Hela cells
Introduction
Cervical cancer (cervical carcinoma, CC), one of the most common gynecological malignancies, remains the second highest cause of cancer-associated morbidity and mortality in women, especially in developing countries such as Asia and Africa (1,2). Currently, neo-adjuvant platinum-based chemotherapy is the most favorable treatment regime for locally advanced cervical cancer. Despite the potency of chemotherapeutic drugs, CC treatment is rarely curative. Conventional treatments for CC lead to a poor prognosis due to a gradual increase in resistance to chemotherapy (3). There is now compelling evidence demonstrating that the emergence of continuous resistance to chemotherapy-induced apoptosis increases the risk of recurrence and contributes to CC progression or metastasis (4-6). Unfortunately, the biological or pathological elements leading to those transitions are not fully understood. Therefore, identifying novel molecular targets for overcoming resistance and investigating effective regimes to induce apoptosis in CC has become a primary goal, indispensable for improving and/or developing targeted therapies. Therefore, effective second-line options are urgently needed.
Clinical studies have reported that the human papilloma virus (HPV) is responsible for the occurrence of CC (7,8). The HPV gene product E6 specifically interferes with the cellular tumor suppressor protein p53, which results in its inactivation, increasing the probability of cancer formation and serving as a feasible trigger for cell immortalization and transformation (9). Approximately 75% of malignant cervical lesions are associated with p53 dysfunction induced by HPV16 (a high-risk HPV type) infection (10). Therefore, the status of p53 is crucial for CC origination, development, and therapy. Restoration of p53 activity possesses strong therapeutic potential. As an example, Yoon et al. demonstrated that TSC-22-mediated inhibition of p53 proteasomal degradation successfully inhibited cell viability and induced apoptosis in the HPV-positive CC cell lines Hela and Caski (11). Lee et al. further showed that vorinostat-induced acetylation of p53 enhanced doxorubicin-induced cytotoxicity in Hela, Caski, and SiHa cells (12). However, another report demonstrated that cisplatin-induced apoptosis was independent of p53 in HPV-positive Hela and SiHa cells (13). Consequently, the involvement of p53 in CC therapy, especially in chemotherapy, remains controversial and needs to be clarified.
In this study, recombinant adenovirus p53 [rAdp53, Gendicine (14,15)] was used in combination with loba to investigate its synergistic anticancer efficacy. We observed that rAdp53 significantly sensitized the loba-induced decrease in cell viability and increase in apoptosis in Hela cells. rAdp53 infection up-regulated the expression of pro-apoptotic proteins Bax and Bak. Taken together, combined treatment with rAdp53 and loba effectively inhibited tumor growth via apoptosis in vivo.
Methods
Cell culture and reagents
Hela cervical cancer cells were purchased from ATCC (Manassas, VA, USA) and cultivated in DMEM containing high glucose and 10% fetal bovine serum (HyClone, Victoria, Australia) at 37 °C with 5% CO2. Anti-PARP polyclonal antibody was purchased from ImmunoWay Biotechnology Company (Newark, DE, USA). Anti-Caspase 8, anti-Caspase 3, anti-Bax, and anti-Bak primary antibodies were obtained from Cell Signaling Technology (Beverly, MA, USA). Anti-GAPDH monoclonal antibody and Hoechst 33258 were obtained from Sigma (St. Louis, MO, USA). Goat anti-rabbit/mouse horseradish peroxidase (HRP)-conjugated secondary antibody was purchased from Santa Cruz Biotechnology (Santa Cruz, CA, USA). Annexin V-PI Apoptosis Detection Kit was obtained from BD Biosciences.
Cell proliferation assays
Cell proliferation (viability) assays were performed as previously described by [3-(4,5-dimethylthiazol-2-yl)-5-(3-carboxymethoxyphenyl)-2-(4-sulfophenyl)-2H-tetrazolium], MTS® (16). Briefly, cells were treated with or without desired conditions. Cell viability was determined using the CellTiter®96 AQueous One Solution Cell proliferation assay kit according to the supplier’s protocol (Promega, WI, USA).
Western blotting
Western blotting was performed to measure protein contents as described previously, with minor modification (17). The cells or tumor tissues were treated and collected, and they were lysed with Nonidet P-40 (1% v/v) lysis buffer containing a protease inhibitor cocktail. After centrifugation at 12,000 rpm for 10 min at 4 °C, the supernatant was collected to prepare cell lysates. The total protein concentration was determined using the Bradford assay. Equivalent amounts of total proteins (20 µg) were loaded, separated by 12% SDS-PAGE gels, and transferred to PVDF membranes. The membranes were blocked with 5% non-fat milk for 30 min, followed by probing with the desired primary antibodies for 2 h at an ambient temperature. After washing three times for 10 min each with 1× PBST, the PDVF membranes were incubated with the appropriate HPR-conjugated secondary antibody. Immunoreactive bands were demonstrated using ECL reagents (Pierce, Rockford, IL, USA). The molecular weight of the protein of interest was assessed using prestained protein markers (Bio-Rad, Hercules, CA, USA). To quantify the Western blot results, the image processing and analysis in Java software (Image J) was used to assess the relative band intensity (RBI) (vs. control).
Hoechst staining
Apoptosis was assessed by nuclei morphological changes based on Hoechst 33258 dye staining. Briefly, 5×104 cells were seeded into 6-well plates. After treatment with various conditions, Hoechst 33258 (10 mg/mL) was added into the culture medium, followed by further incubation for 15–30 min in an incubator. Apoptotic nuclei were analyzed using an inverted fluorescence microscope (Nikon, Japan). To count the number of apoptotic cells and calculate the apoptotic ratio, six to eight randomly selected areas under the objective lens were counted for the number of apoptotic cells with condensed nuclei or fragmented DNA.
Flow cytometry
Apoptotic cells were characterized with phosphatidylserine (PS) exposure on the outer cell membrane. Apoptosis was examined by flow cytometry based on Annexin V-PI double staining as described previously (18). Briefly, after cells were treated with desired conditions, cells were harvested and stained with fluorescent probes (Annexin V-FITC, green; PI, red). Health cells showed FITC and PI double-negative staining, early apoptotic cells showed FITC-positive and PI-negative staining, and later apoptotic cells were FITC and PI double-positive. Cell sorting was performed using a Flow cytometry (BD Calibur, USA) and analyzed with CellQuest software (BD).
Tumor suppression in vivo
Twenty 6–8-week-old female BALB/c-nu/nu mice were locally bred and used for implantation. They were housed under specific pathogen-free (SPF) conditions with ad libitum access to food and water supply. The mice with 22–25 g net weight were divided into four groups. Further, 1×106 control Hela cells or rAdp53-infected Hela cells in PBS or saline were injected subcutaneously into the right flank of all test mice for xenograft tumors (n=5 mice/group). When the tumor volume reached around 100 mm3 (the tumor volume was determined as v =1/2ab2 with a digital caliper), mice were treated with desired conditions. After treatment, mice were anesthetized, and the tumor was excised from treated or untreated mice and photographed. After the tumor masses were weighed, tissues from different treatment groups were processed for Western blotting to analyze apoptosis and p53 expression.
Statistical analysis
The experiments were repeated at least three times for MTS, flow cytometry, and Western blotting assays. Consistent data were collected, and representative results were shown. Data were expressed as the mean ± standard error (SE). Significance comparisons among different groups were calculated using one-way ANOVA and the Student’s t-test. P<0.05 was considered to be statistically significant.
Results
rAdp53 enhances loba-induced apoptotic morphological changes in Hela cells
To investigate the effects of rAdp53, loba, or rAdp53 and loba on cancer cell proliferation, Hela cells were treated with rAdp53, loba, or rAdp53 and loba for 48 h. Cell death was measured through morphology, either under a phase-contrast inverted microscope or fluorescence microscope based on Hoechst 333258 staining. In comparison with controls, rAdp53, or loba single treatment, we found that only the combination of rAdp53 and loba induced significant apoptosis (Figure 1). These data suggest that loba induces more apoptosis in the presence of p53 over-expression.
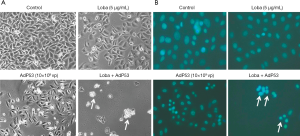
rAdP53 enhances loba-induced cell growth inhibition in Hela cells
To examine whether rAdp53 could enhance the decrease in loba-induced cell viability in CC cells, Hela cells were treated with rAdp53, loba, or rAdp53 plus loba for 48 h. Relative cell viability was assessed through MTS assays. Our results showed that rAdp53 pretreatment markedly inhibited cell viability in Hela cells (Figure 2). These data suggest that p53 is able to act synergistically with loba to induce inhibition of cell growth.
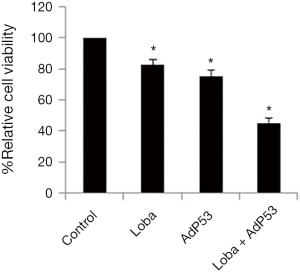
Quantitative analysis of apoptosis induced by loba and rAdp53
To further evaluate the apoptosis induced by the combined rAdp53 and loba treatment, cells were treated with rAdp53, loba, or rAdp53 plus loba. Annexin V-PI double staining was performed for flow cytometry. Our data showed that the combined rAdp53 and loba treatment induced markedly more apoptosis than either single treatment in Hela cells (Figure 3).
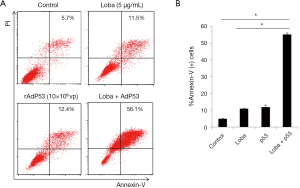
Effects of rAdp53 and loba combined treatment on apoptotic protein expression in Hela cells
To further assess apoptosis induced by the combined rAdp53 and loba treatment, intracellular apoptotic molecular biochemical events were examined. Hela cells were untreated or treated with rAdp53, loba, or rAdp53 plus loba for 48 h. Western blotting results demonstrated that loba incubation triggered significant much higher cysteine-aspartic protease (Caspase) and Poly (ADP-ribose) polymerase (PARP) cleavage activation in rAdp53-infected cells (Figure 4). To investigate the mechanism of rAdp53-enhanced loba-induced apoptosis in CC cells, Hela cells were treated with different concentrations of p53 virus particles (vp) for 48 h. Our data indicated that the pro-apoptotic proteins Bcl-2-associated X protein (Bax) and Bcl-2 homologous antagonist killer (Bak) were significantly up-regulated by rAdp53 infection (Figure 5). These data suggest that p53 may enhance loba-induced apoptosis in Hela cells through up-regulation of pro-apoptotic proteins.
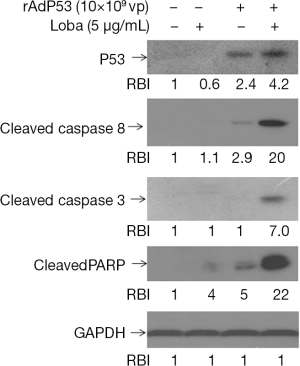
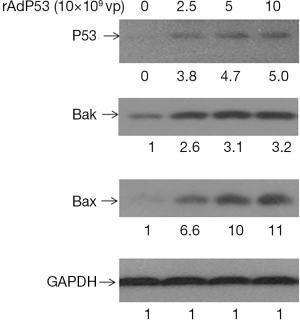
Loba and rAdp53 combination induces tumor growth inhibition
To verify the in vitro findings based on cellular tests, tumor inhibition efficacies in terms of tumor volumes and masses in xenograft mice were assessed. Analysis of CC xenograft tumors from the control buffer, rAdp53, loba, or loba plus rAdp53-treated mice revealed that the combined administration of loba and rAdp53 effectively inhibited CC tumor growth in vivo (Figure 6A). Excised tumor weight analysis indicated that there was a marked difference in tumor mass with at least a 4-fold decrease in the combination-treated mice when compared with untreated control or single-treated mice (Figure 6B). Moreover, tumor tissues from different treatment groups were processed for Western blotting to examine p53 and cleaved Caspase 3 protein levels. Our results indicated that only the combination treatment significantly induced Caspase 3 activation in the xenograft CC tumors (Figure 6B). These data suggest that loba and rAdp53 indeed synergistically exert anti-cancer therapeutic effects in vivo.
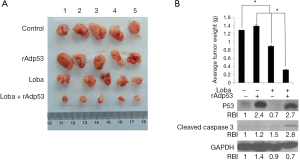
Discussion
Besides breast cancer, CC is the most common cause of cancer-related death in women worldwide. Radical surgery management of early-stage cervical carcinoma, including radical hysterectomy (RH), laparoscopic radical hysterectomy (LRH), or laparoscopic nerve-sparing radical hysterectomy (LNRH), has been developed and is routinely used clinically (19-21). However, for advanced stage cervical carcinoma, metastasis and recurrence are the main impediments that prevent cures of this malignancy. The standard of care for advanced cervical cancer has been RH followed by systemic chemotherapy: neoadjuvant chemotherapy (NAC) (22,23). The benefits and risks of NAC to treat locally advanced cervical cancer remain to be determined. Lobaplatin, as the third-generation platinum and the first-line chemotherapeutic strategy for a wide range of preclinical tumor models, exhibits efficacies to neutralize resistance of some types of malignancies to cisplatin or carboplatin (24). Lobaplatin has shown forceful anti-neoplasm ability and has been used in NAC to treat solid tumors, including breast cancer, liver cancer, and lung cancer (25-27). However, there are few studies on lobaplatin’s neoadjuvant chemotherapeutic effects on cervical carcinoma. In the present study, we demonstrated that rAdp53 and loba synergistically exert anti-cancer effects in CC. rAdp53 enhanced loba-induced apoptosis and cell growth inhibition. rAdp53 introduction up-regulates expression of the Bcl-2 family proapoptotic proteins Bax and Bak. The results imply that rAdp53 may collaborate with loba to inhibit xenograft cervical tumor growth in vivo.
We initially tested the apoptotic effects of either agent alone by treating Hela cells with different concentrations of loba (0, 1, 2.5, 5.0, 10, and 20 µg/mL) or rAdp53 (0, 2.5, 5.0, and 10×109 vp) (data not shown). Our data indicated that Hela cells were relatively resistant to low concentrations of loba (≤5 µg/mL). These findings are consistent with some previous studies that cancer cells are not sensitive to low doses of loba (≤10 µg/mL) (28). However, they are in contrast with other reports that found cancer cells are sensitive to low doses of loba (≤5 µg/mL) (29). To investigate the mechanism underlying this apoptotic resistance, we infected cells with adenovirus harboring the p53 gene that produces rAdp53. We found that low concentrations of rAdp53 (≤109 vp) were relatively safe for Hela cells. Interestingly, our data revealed that pretreatment of Hela cells with rAdp53 markedly increased their sensitivity to loba-induced apoptosis. These data suggest that p53 plays a key role in mediating loba-induced apoptosis in CC cells. It is well known that the high-risk HPV oncoprotein E6 can interfere with tumor suppressor p53 function by promoting its ubiquitylation and proteasomal degradation or by inducing a p53 mutation. Here, we provide solid data that reveals the p53 restoration in CC cells sensitizes cells to loba-induced cytotoxicity, suggesting that rAdp53 could be considered as a co-treatment for patients with CC that are treated clinically with loba. These findings are also in agreement with previous reports that loba can exert anti-cancer effects on liver cancer via a p53-mediated apoptotic axis (30). It has been reported that p53 can activate the Bcl-2 family protein Bad to mediate doxorubicin-induced cytotoxicity in cervical cancer (31). To further clarify the mechanism underlying p53-sensitized apoptosis induced by loba, our Western blotting data indicated that rAdp53 promoted expression of two other Bcl-2 family proapoptotic proteins, Bax and Bak, in CC cells. Since Bax and Bak can be activated through oligomerization and relocate to the mitochondria, these findings suggest that rAdp53 and loba might trigger an intrinsic apoptotic signaling pathway. Our data support the notion that the chemotherapy drug cisplatin-induced apoptosis in non-small-cell lung cancer (NSCLC) cells is dependent on p53-mediated Bax/Bak activation (32). Secondly, using a SCID mouse model burdened with CC derived from Hela cells, we observed that treatment with rAdp53 and loba combined significantly inhibited tumor growth in vivo, suggesting that over-expression of p53 can indeed play a vital role in CC chemotherapy. The Western blotting results further demonstrated that p53 introduction sensitized loba-induced Caspase 3 cleaved activation. Therefore, the tumor growth inhibitory effects induced by these two agents are based on stimulation of apoptosis in vivo.
Conclusions
The study showed that rAdp53 enhanced the ability of loba to induce apoptosis via upregulation of the proapoptotic proteins Bax and Bak. It further demonstrated that this synergistic anticancer effect via apoptosis functions in vivo. Nevertheless, we have acknowledged that more cell lines and primary cells from patients are necessary, which is one of the limitations of this study. This study is only one part of our ongoing novel therapeutic regimes development projects for cervical cancer. We will conduct additional clinical studies to improve the evaluation of this combination in the future. Further investigation is needed to elucidate the toxicity and/or safety of this drug combination for other organs.
Acknowledgments
Funding: This work was supported by the National Science and Technology Major Projects for Major New Drugs Innovation and Development of China (2012ZX09303015 to Q Guo) and the National Natural Science Foundation of China (31371425 to X Zhao and 81771947 to Z Lu).
Footnote
Conflicts of Interest: All authors have completed the ICMJE uniform disclosure form (available at http://dx.doi.org/10.21037/tcr.2018.08.25). The authors have no conflicts of interest to declare.
Ethical Statement: The authors are accountable for all aspects of the work in ensuring that questions related to the accuracy or integrity of any part of the work are appropriately investigated and resolved. This study was performed according to protocols approved by the Committee for Animal Experiments Guidelines on Animal Welfare of the China Medical University Shengjing Hospital (Shenyang, Liaoning, China).
Open Access Statement: This is an Open Access article distributed in accordance with the Creative Commons Attribution-NonCommercial-NoDerivs 4.0 International License (CC BY-NC-ND 4.0), which permits the non-commercial replication and distribution of the article with the strict proviso that no changes or edits are made and the original work is properly cited (including links to both the formal publication through the relevant DOI and the license). See: https://creativecommons.org/licenses/by-nc-nd/4.0/.
References
- Nogueira-Rodrigues A, de Melo AC, Garces AH, et al. Patterns of Care and Outcome of Elderly Women Diagnosed With Cervical Cancer in the Developing World. Int J Gynecol Cancer 2016;26:1246-51. [Crossref] [PubMed]
- Martinez-Nava GA, Fernandez-Nino JA, Madrid-Marina V, et al. Cervical Cancer Genetic Susceptibility: A Systematic Review and Meta-Analyses of Recent Evidence. PLoS One 2016;11:e0157344 [Crossref] [PubMed]
- Boussios S, Seraj E, Zarkavelis G, et al. Management of patients with recurrent/advanced cervical cancer beyond first line platinum regimens: Where do we stand? A literature review. Crit Rev Oncol Hematol 2016;108:164-74. [Crossref] [PubMed]
- Sadalla JC, Andrade JM, Genta ML, et al. Cervical cancer: what's new? Rev Assoc Med Bras (1992) 2015;61:536-42. [PubMed]
- Cai L, Wang Z, Liu D. Interference with endogenous EZH2 reverses the chemotherapy drug resistance in cervical cancer cells partly by up-regulating Dicer expression. Tumour Biol 2016;37:6359-69. [Crossref] [PubMed]
- Ferrandina G, Lauriola L, Distefano MG, et al. Increased cyclooxygenase-2 expression is associated with chemotherapy resistance and poor survival in cervical cancer patients. J Clin Oncol 2002;20:973-81. [Crossref] [PubMed]
- Street D, Delgado G. The role of p53 and HPV in cervical cancer. Gynecol Oncol 1995;58:287-8. [Crossref] [PubMed]
- Ngan HY, Stanley M, Liu SS, et al. HPV and p53 in cervical cancer. Genitourin Med 1994;70:167-70. [PubMed]
- Akeel RA. Identification of HPV Integration and Genomic Patterns Delineating the Clinical Landscape of Cervical Cancer. Asian Pac J Cancer Prev 2015;16:8041-5. [Crossref] [PubMed]
- Wu G, Liu D, Jiang K, et al. PinX1, a novel target gene of p53, is suppressed by HPV16 E6 in cervical cancer cells. Biochim Biophys Acta 2014;1839:88-96. [Crossref] [PubMed]
- Yoon CH, Rho SB, Kim ST, et al. Crucial role of TSC-22 in preventing the proteasomal degradation of p53 in cervical cancer. PLoS One 2012;7:e42006 [Crossref] [PubMed]
- Lee SJ, Hwang SO, Noh EJ, et al. Transactivation of bad by vorinostat-induced acetylated p53 enhances doxorubicin-induced cytotoxicity in cervical cancer cells. Exp Mol Med 2014;46:e76 [Crossref] [PubMed]
- Michnov O, Solomayer E, Fehm T, et al. Knock down of p53 or its ubiquitin ligase E6AP does not affect the sensitivity of human papillomavirus-positive cervical cancer cells to cisplatin. Am J Cancer Res 2012;2:309-21. [PubMed]
- Peng Z. Current status of gendicine in China: recombinant human Ad-p53 agent for treatment of cancers. Hum Gene Ther 2005;16:1016-27. [Crossref] [PubMed]
- Wilson JM. Gendicine: the first commercial gene therapy product. Hum Gene Ther 2005;16:1014-5. [Crossref] [PubMed]
- Zheng D, Cho YY, Lau AT, et al. Cyclin-dependent kinase 3-mediated activating transcription factor 1 phosphorylation enhances cell transformation. Cancer Res 2008;68:7650-60. [Crossref] [PubMed]
- Zhao X, Liu Y, Du L, et al. Threonine 32 (Thr32) of FoxO3 is critical for TGF-beta-induced apoptosis via Bim in hepatocarcinoma cells. Protein Cell 2015;6:127-38. [Crossref] [PubMed]
- Zheng Y, Shi Y, Tian C, et al. Essential role of the voltage-dependent anion channel (VDAC) in mitochondrial permeability transition pore opening and cytochrome c release induced by arsenic trioxide. Oncogene 2004;23:1239-47. [Crossref] [PubMed]
- Liu S, Gu X, Zhu L, et al. Effects of propofol and sevoflurane on perioperative immune response in patients undergoing laparoscopic radical hysterectomy for cervical cancer. Medicine (Baltimore) 2016;95:e5479 [Crossref] [PubMed]
- Hanprasertpong J, Jiamset I, Geater A, et al. Clinical Aspects and Prognostic Factors for Survival in Patients with Recurrent Cervical Cancer after Radical Hysterectomy. Oncol Res Treat 2016;39:704-11. [Crossref] [PubMed]
- Kyo S, Kato T, Nakayama K. Current concepts and practical techniques of nerve-sparing laparoscopic radical hysterectomy. Eur J Obstet Gynecol Reprod Biol 2016;207:80-8. [Crossref] [PubMed]
- Yang Y, Qin T, Zhang W, et al. Laparoscopic nerve-sparing radical hysterectomy for bulky cervical cancer (>/=6 cm) after neoadjuvant chemotherapy: A multicenter prospective cohort study. Int J Surg 2016;34:35-40. [Crossref] [PubMed]
- Takatori E, Shoji T, Takada A, et al. A retrospective study of neoadjuvant chemotherapy plus radical hysterectomy versus radical hysterectomy alone in patients with stage II cervical squamous cell carcinoma presenting as a bulky mass. Onco Targets Ther 2016;9:5651-7. [Crossref] [PubMed]
- Li WP, Liu H, Chen L, et al. A clinical Comparison of Lobaplatin or Cisplatin with Mitomycine and Vincristine in Treating Patients with Cervical Squamous Carcinoma. Asian Pac J Cancer Prev 2015;16:4629-31. [Crossref] [PubMed]
- Wang JQ, Wang T, Shi F, et al. A Randomized Controlled Trial Comparing Clinical Outcomes and Toxicity of Lobaplatin- Versus Cisplatin-Based Concurrent Chemotherapy Plus Radiotherapy and High-Dose-Rate Brachytherapy for FIGO Stage II and III Cervical Cancer. Asian Pac J Cancer Prev 2015;16:5957-61. [Crossref] [PubMed]
- Li X, Ran L, Fang W, et al. Lobaplatin arrests cell cycle progression, induces apoptosis and alters the proteome in human cervical cancer cell Line CaSki. Biomed Pharmacother 2014;68:291-7. [Crossref] [PubMed]
- Kirpensteijn J, Teske E, Kik M, et al. Lobaplatin as an adjuvant chemotherapy to surgery in canine appendicular osteosarcoma: a phase II evaluation. Anticancer Res 2002;22:2765-70. [PubMed]
- Wu Q, Qin SK, Teng FM, et al. Lobaplatin arrests cell cycle progression in human hepatocellular carcinoma cells. J Hematol Oncol 2010;3:43. [Crossref] [PubMed]
- Yin CY, Lin XL, Tian L, et al. Lobaplatin inhibits growth of gastric cancer cells by inducing apoptosis. World J Gastroenterol 2014;20:17426-33. [Crossref] [PubMed]
- Wang Y, Zheng WL, Ma WL. Lobaplatin inhibits the proliferation of hepatollular carcinoma through p53 apoptosis axis. Hepat Mon 2012;12:e6024 [Crossref] [PubMed]
- Lee SJ, Hwang SO, Noh EJ, et al. Transactivation of bad by vorinostat-induced acetylated p53 enhances doxorubicin-induced cytotoxicity in cervical cancer cells. Exp Mol Med 2014;46:e76 [Crossref] [PubMed]
- Matsumoto M, Nakajima W, Seike M, et al. Cisplatin-induced apoptosis in non-small-cell lung cancer cells is dependent on Bax- and Bak-induction pathway and synergistically activated by BH3-mimetic ABT-263 in p53 wild-type and mutant cells. Biochem Biophys Res Commun 2016;473:490-6. [Crossref] [PubMed]