Squaring the circle: sponging microRNAs in gastric cancer
We have known for some time that the instead of transcribing the roughly 2% of the genome that encodes for proteins, our cells transcribe approximately 75% of our genome (1). That equates to an awful lot of what we now call non-coding RNA (ncRNA). ncRNA itself can be loosely separated into two classes based on size: short ncRNAs (those shorter than 200 bases), and long ncRNAs (those greater than 200 bases) (2,3).
One of the best studied of the short ncRNA species are microRNAs (miRNAs), and they play critical roles in carcinogenesis primarily via their roles in the posttranscriptional regulation of gene expression (4). Because miRNAs are so small, and the region used by them (called the miRNA seed region) to recognise and elicit their effects through base pairing to sites within their target mRNAs is even smaller (nucleotides 2–8), this results in miRNAs having the capacity to target many different target mRNAs. From this realization, a hypothesis known as the competitive endogenous RNA (ceRNA) hypothesis was developed (5). In this hypothesis, mRNAs that share miRNA-response elements (MREs) with other mRNAs compete these miRNAs and this competition for binding to shared miRNAs, results in gene regulatory networks, that both communicate with and co-regulate each other result in important roles in human development and disease (6).
Around this time circular RNAs (circRNAs) were identified as distinct ncRNA entities. For many years we thought of these as artefacts of splicing (perhaps even as a remnant of lariat intermediates) (7). circRNAs are generated from the back-splicing of exons, introns, or both to form exonic or intronic circRNAs (7). A role for circRNAs was linked to the ceRNA hypothesis when it was discovered that certain circRNAs such as CDR1-AS (also known as ciRS-7) are able to act as a molecular sponge for miR-7 (8), and subsequently shown to play important roles in diverse cancers and disease (7,9-11). They achieve this because these circRNAs have numerous binding sites for a specific miRNA and so are completely dedicated to their role of harbouring miRNAs.
In contrast, binding of a miRNA to a ceRNA not only prevents that miRNA from binding to other MREs, but can also suppress translation from the coding portion of the ceRNA. Hence, compared with circRNAs, ceRNAs operate in more complex networks of interacting molecules affecting mRNA translation.
Gastric cancer is a hard to treat intractable cancer with poor prognosis (12,13). Several circRNAs such as CDR1-AS have now been implicated in gastric cancer (14,15). In a recent article Liu et al. described how a circRNA derived from YAP1 plays roles in gastric cancer by a ceRNA network involving hsa-miR-367-5p and p27kip1 (16). In this paper the authors used an in silico-based strategy to select a circRNA they call circYAP1 from CircNet (17). The rationale for deriving this is unclear, the authors in their discussion “combined the reported literature with a circNet analysis to find circYAP1, which is derived from the YAP1 gene locus…”. This is non-intuitive based on the way CircNet operates. In the first instance you either input a gene or a miRNA of interest such as YAP1. In this instance a network of circRNAs and miRNAs is derived. For the sake of this example I have highlighted one such (circYAP1.45) (Figure 1A). Intriguingly, this does not directly associate hsa-miR-367-5p with this circRNA. However, if you click on the relevant circRNA and move to tap for “sponge” in CircNet (Figure 1B), a network for this miRNA is derived, which does indicate that this circRNA can potentially “sponge” this miRNA (Figure 1B). However, according to Liu et al. (17), the circRNA identified by them from CircNet was hsa_circ_0002320. Indeed, if you take the RNA associated with say circYAP1.45 from CircNet (Figure1C), and blast it against circBase (18), then indeed the first circRNA that emerges is hsa_circ_0002320 (Figure 1D). There are however, 9 other circRNAs with identical homology to this region and all derived from YAP1. Nevertheless, the authors designed divergent primers to examine expression of circYAP1 (hsa_circ_0002320) in patients with gastric cancer. In an initial screen this circRNA was found to be downregulated in the cancer tissue than in adjacent normal tissues. The authors then confirm this by designing a FISH based probe to examine the relative levels of this circRNA in an FFPE embedded patient tissue microarray (TMA) with a larger number of patients. When stratified according to tumour stage, the levels of this circRNA were found to be higher in early stage gastric cancer than those with advanced stage disease. This was reflected in patient overall survival (OS) where high expression of circYAP1 was associated with both longer OSs, and also found to be associated with OS response to adjuvant chemotherapy. Having established that this circRNA is altered in gastric cancer, the authors then identified 5 miRNAs using CircInteractome and CircNet were used to predict 5 potential target miRNAs (hsa-miR-1200, hsa-miR-330-5p, hsa-miR-367-5p, hsa-miR-513a-3p and hsa-miR-513c-3p) that could bind with the circYAP1 sequence, the rationale being that the main currently known function of circRNAs are to “sponge” miRNAs. One potential limitation with the current study is that the number of potential miRNA seed-targets for each miRNA has not been provided. The original study by Hansen et al. (8) on the circRNAs ciRS-7 found that it contained n=73 conventional seed-targets for miR-7. In this regard, an examination of the circYAP1 (hsa_circ_0002320) for putative miRNA binding sites using CircInteractome predicts that of the 5 putative miRNAs analysed by Liu et al., miR-1200, miR-513a-3p have 1 putative binding site, while miR-330-5p has 2 putative binding sites, while the number of putative binding sites for miR-367-5p and miR-513c-3p are not found.
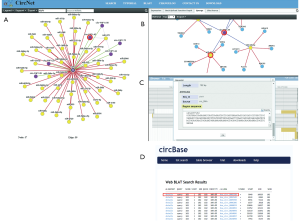
To test whether or not this circRNA could sponge any of the 5 miRNAs identified as potential targets in their initial analysis, the authors overexpressed this circRNA in vitro in two gastric cancer cell lines, and identified one hsa-mir-367-5p, which was elevated in both cell lines following overexpression of this circRNA. RNA in vivo precipitation (RIP) with a circYAP1 specific probe, followed by qPCR analysis confirmed an enrichment of this miRNA. Finally, a dual RNA FISH based approach showed co-localization of both circYAP1 and hsa-miR-367-5p.
Is this sponging? Whilst it is certainly true that the experiments demonstrate that circYAP1 and hsa-mir-367-5p interact, critical experiments demonstrating whether circYAP1 acts as a molecular sponge for this miRNA are lacking. To functionally demonstrate sponging Hansen et al. (8), first developed vectors capable of expressing the circRNA either as circular or linear forms. Then by co-expressing the circRNA or the linear RNA producing vectors with target miRNA vectors they demonstrated that the circRNA is resistant to conventional miRNA destabilization of mRNA and as such not prone to target miRNA dependent regulation. They further confirmed the ability of the miRNA to sponge by then generating two constructs containing either a perfect miRNA target or the entire circRNA sequence in the 3’-untranslated region (UTR) of a luciferase. Co-transfecting these constructs alongside either miRNA overexpression vectors or the individual circRNAs can then test for a reduction of knockdown potential. A substantial reduction in knockdown of luciferase activity indicates potential miRNA sponging. Such controls were not conducted in the manuscript by Liu et al. (16).
Whilst the evidence for miRNA sponging is undefined, the data by Liu et al. (16) clearly demonstrate that circYAP1 acts as a ceRNA.
Overexpression of this circRNA was subsequently found to be associated with cell cycle arrest at the G1 phase of the cell cycle, reduced cellular proliferation, and decreased invasiveness, which could be reversed by co-transfecting cells with hsa-mir-367-5p mimics, while siRNA directed knockdown of the circRNA was associated with increased cellular proliferation, colony formation and migration, again which could be abrogated in this instance by co-transfection with a miR-367-5p inhibitor.
In vivo, overexpression of circYAP1 was associated with decreased tumour volumes in a murine flank model, with an associated decreased Ki-67 expression. However, it must be noted that the numbers of animals in each arm appears to be limited to (n=3), and was restricted only one cell line.
Having identified that circYAP1 can act as a ceRNA for hsa-miR-367-5p, the authors then used TargetScan (19), to predict potential targets of this miRNA. From this analysis, they selected p27kip1 as their top candidate. Again, the rationale for picking this candidate is not intuitive as (I) when CircNet used miRTarBase as the methodology to form the networks demonstrated on CircNet. In this regard, p27kip1 is not in the CircNet network which suggests that the top candidate genes are CDK4, CDH13, ERVMER34-1, YAF2 and ZNF256 and (II) it would not appear to be the top candidate from a TargetScan search using the search term “hsa-mir_367-5p” (the current top candidate from TargetScan is LCMT2, based on either TargetScan Release 7.0/August 2015 or the current Release 7.2/March 2018).
It may be that they chose this mRNA on the basis that it an important regulator of the cell cycle, which is something that would intuitively be worth investigating given the effects seen by the authors of circYAP1 (and its potential sponging of hsa-miR-367-5p) on the cell cycle. When tested Liu et al. (16), clearly demonstrate that this miRNA targets this important cell cycle kinase inhibitor (CKI). To demonstrate that circYAP1 sponging of this miRNA is involved with regulating this CKI; the authors overexpressed circYAP1 and found that that levels of this CKI increased significantly. Furthermore, if they then transfected in a miRNA mimic of hsa-miR-367-5p, this was sufficient to abrogate this effect. In support of this, knockdown of the circRNA also led to decreased expression of p27kip1 (16).
So, what does this mean with respect to gastric cancer? As summarized in Figure 2, the authors present an axis centred on circYAP1 sponging hsa-miR-367-5p as a critical regulator of normal gastric cell homeostasis. By sponging hsa-miR-367-5p, and as such decreasing the free levels of this important miRNA, levels of p27kip1 are regulated to allow for balanced cellular proliferation and growth (Figure 2). In the progression to gastric cancer however, levels of circYAP1 decrease, resulting in increased levels of hsa-miR-367-5p, and a corresponding decrease in the critical CKI p27kip1. As such this leads to inappropriate control of the cell cycle, and concomitant dysregulation of cellular proliferation and invasion (Figure 2).
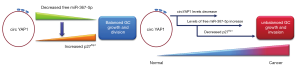
It’s a nice simple axis, but does it in fact “square the circle”? Several questions still remain with regards to this axis. For instance, it is well established that levels of YAP1 mRNA and protein are increased in gastric cancer (20,21). Why then would levels of circYAP1 be decreased in gastric cancer given that it is derived from transcription of the YAP1 locus? This suggests that the higher transcription of YAP1 mRNA could lead to improved splicing of transcripts, with a resultant decrease in back-splicing and a subsequent decrease in circYAP1 levels. Early evidence suggests that circRNAs compete with mRNAs for the existing splicing machinery (22). Does this however mean that there is an in-built “error” rate under normal physiological conditions resulting in back-splicing of YAP1 to create circYAP1 ncRNAs which are essential for regulating normal cellular proliferation and growth by sponging critical miRNAs such as hsa-miR-367-5p? Once cancer has initiated more efficient splicing of upregulated linear mRNAs such as that for YAP1 may take priority for the cellular splicing machinery, resulting in decreased back-splicing and result in loss of circYAP1. It would also have been nice to see if levels of p27kip1 were altered in the tumour developed in mice following overexpression of circYAP1 It must also be noted that this particular miRNA has been shown to also directly repress Rab23 in gastric cancer (23), so the ceRNA network identified by Liu et al. (16), may have multiple as yet unidentified miRNAs/mRNAs/proteins that could also be regulated by circYAP1. The large numbers of additional circRNAs associated with YAP1 have also yet to be fully interrogated. The notion that all circRNAs act as molecular sponges has also been called into question (24), and more recent evidence suggests that circRNAs may also encode peptides (25), suggests that the full complexity of the circYAP1 miRNA sponge/ceRNA network has yet to be fully delineated.
Acknowledgments
Funding: None.
Footnote
Provenance and Peer Review: This article was commissioned and reviewed by the Section Editor Chunlin Ou (Cancer Research Institute of Central South University, Changsha, China).
Conflicts of Interest: The author has completed the ICMJE uniform disclosure form (available at http://dx.doi.org/10.21037/tcr.2019.01.15). The author has no conflicts of interest to declare.
Ethical Statement: The author is accountable for all aspects of the work in ensuring that questions related to the accuracy or integrity of any part of the work are appropriately investigated and resolved.
Open Access Statement: This is an Open Access article distributed in accordance with the Creative Commons Attribution-NonCommercial-NoDerivs 4.0 International License (CC BY-NC-ND 4.0), which permits the non-commercial replication and distribution of the article with the strict proviso that no changes or edits are made and the original work is properly cited (including links to both the formal publication through the relevant DOI and the license). See: https://creativecommons.org/licenses/by-nc-nd/4.0/.
References
- Djebali S, Davis CA, Merkel A, et al. Landscape of transcription in human cells. Nature 2012;489:101-8. [Crossref] [PubMed]
- Thum T, Condorelli G. Long noncoding RNAs and microRNAs in cardiovascular pathophysiology. Circ Res 2015;116:751-62. [Crossref] [PubMed]
- Deveson IW, Hardwick SA, Mercer TR, et al. The Dimensions, Dynamics, and Relevance of the Mammalian Noncoding Transcriptome. Trends Genet 2017;33:464-78. [Crossref] [PubMed]
- Saliminejad K, Khorram Khorshid HR, Soleymani Fard S, et al. An overview of microRNAs: Biology, functions, therapeutics, and analysis methods. J Cell Physiol 2019;234:5451-65. [Crossref] [PubMed]
- Tay Y, Rinn J, Pandolfi PP. The multilayered complexity of ceRNA crosstalk and competition. Nature 2014;505:344-52. [Crossref] [PubMed]
- Karreth FA, Pandolfi PP. ceRNA cross-talk in cancer: when ce-bling rivalries go awry. Cancer Discov 2013;3:1113-21. [Crossref] [PubMed]
- Greene J, Baird AM, Brady L, et al. Circular RNAs: Biogenesis, Function and Role in Human Diseases. Front Mol Biosci 2017;4:38. [Crossref] [PubMed]
- Hansen TB, Jensen TI, Clausen BH, et al. Natural RNA circles function as efficient microRNA sponges. Nature 2013;495:384-8. [Crossref] [PubMed]
- Hansen TB, Kjems J, Damgaard CK. Circular RNA and miR-7 in cancer. Cancer Res 2013;73:5609-12. [Crossref] [PubMed]
- Xu H, Guo S, Li W, et al. The circular RNA Cdr1as, via miR-7 and its targets, regulates insulin transcription and secretion in islet cells. Sci Rep 2015;5:12453. [Crossref] [PubMed]
- Geng HH, Li R, Su YM, et al. The Circular RNA Cdr1as Promotes Myocardial Infarction by Mediating the Regulation of miR-7a on Its Target Genes Expression. PLoS One 2016;11:e0151753. [Crossref] [PubMed]
- Tan AC, Chan DL, Faisal W, et al. New drug developments in metastatic gastric cancer. Therap Adv Gastroenterol 2018;11:1756284818808072. [Crossref] [PubMed]
- Torre LA, Bray F, Siegel RL, et al. Global cancer statistics, 2012. CA Cancer J Clin 2015;65:87-108. [Crossref] [PubMed]
- Pan H, Li T, Jiang Y, et al. Overexpression of Circular RNA ciRS-7 Abrogates the Tumor Suppressive Effect of miR-7 on Gastric Cancer via PTEN/PI3K/AKT Signaling Pathway. J Cell Biochem 2018;119:440-6. [Crossref] [PubMed]
- Shi P, Wan J, Song H, et al. The emerging role of circular RNAs in gastric cancer. Am J Cancer Res 2018;8:1919-32. [PubMed]
- Liu H, Liu Y, Bian Z, et al. Circular RNA YAP1 inhibits the proliferation and invasion of gastric cancer cells by regulating the miR-367-5p/p27 (Kip1) axis. Mol Cancer 2018;17:151. [Crossref] [PubMed]
- Liu YC, Li JR, Sun CH, et al. CircNet: a database of circular RNAs derived from transcriptome sequencing data. Nucleic Acids Res 2016;44:D209-15. [Crossref] [PubMed]
- Glažar P, Papavasileiou P, Rajewsky N. circBase: a database for circular RNAs. RNA 2014;20:1666-70. [Crossref] [PubMed]
- Agarwal V, Bell GW, Nam JW, et al. Predicting effective microRNA target sites in mammalian mRNAs. Elife 2015;4: [Crossref] [PubMed]
- Yu L, Gao C, Feng B, et al. Distinct prognostic values of YAP1 in gastric cancer. Tumour Biol 2017;39:1010428317695926. [Crossref] [PubMed]
- Kang W, Tong JH, Chan AW, et al. Yes-associated protein 1 exhibits oncogenic property in gastric cancer and its nuclear accumulation associates with poor prognosis. Clin Cancer Res 2011;17:2130-9. [Crossref] [PubMed]
- Ashwal-Fluss R, Meyer M, Pamudurti NR, et al. circRNA biogenesis competes with pre-mRNA splicing. Mol Cell 2014;56:55-66. [Crossref] [PubMed]
- Bin Z, Dedong H, Xiangjie F, et al. The microRNA-367 inhibits the invasion and metastasis of gastric cancer by directly repressing Rab23. Genet Test Mol Biomarkers 2015;19:69-74. [Crossref] [PubMed]
- Thomson DW, Dinger ME. Endogenous microRNA sponges: evidence and controversy. Nat Rev Genet 2016;17:272-83. [Crossref] [PubMed]
- Granados-Riveron JT, Aquino-Jarquin G. The complexity of the translation ability of circRNAs. Biochim Biophys Acta 2016;1859:1245-51. [Crossref] [PubMed]