Androgen attenuates antitumor effects of gastric cancer cells by bone marrow mesenchymal stem cells via restricting the JNK signaling activation
Introduction
Gastric carcinoma is the second most frequent malignancy in the world (1). Although the overall incidence of gastric carcinoma has been declining in the United States and Western Europe in recent years, it is pretty high in certain Asian countries, South America and parts of Eastern Europe (2). In addition, the mortality of gastric carcinoma varies from regions to regions and is interrelated to gender. Also, the death rate of gastric carcinoma in males is significantly higher than that in females, and China’s mortality of gastric carcinoma notably exceeds developed countries (3). With the development of tumor molecular biology, researchers investigate the underlying mechanism of tumor occurrence and progression at the molecular level, thus providing theoretical basis to the development of more effective clinical therapy methods.
Bone marrow mesenchymal stem cells (BMMSCs), which is a type of non-hematopoietic stem cells found in bone marrow, can be induced to differentiate into a variety of tissue cells and facilitate not only the transfection and expression of external genes in vitro, but also chemotactic migration to tumor tissues under specific environment in vivo and in vitro (4,5). At present, it is shown that BMMSCs can release a large number of cytokines to affect the growth of tumor, while the effect of BMMSCs on the growth of tumor cells remains unclearly defined and controversial (6). Certain studies have shown that bone marrow-derived stem cells participated in tumor angiogenesis, thereby promoting tumor growth. For instance, Wu suggested that injection around the wound and application to the wound bed of green fluorescence protein(+) allogeneic BMMSCs significantly increased angiogenesis and wound healing in mice (7). Zhang found that BMMSCs could promote breast and prostate tumor growth and suggested that BMMSCs could migrate and settle in tumor cells to form tumor blood vessels and regulate the microenvironment of a tumor by increasing the expressions of pro-angiogenic factors (8). Tu suggested that BMMSCs could promote the proliferation of Saos-2 osteosarcoma through IL-6/STAT3 signaling, which, by increasing cell metastasis, invasion and distant metastasis of osteosarcoma, enhanced the survival signals of Saos-2 cells (9). In addition, BMMSCs could induce an epithelial-mesenchymal transition (EMT) process. Giannoni reported that once breast cancer cells contacted with BMMSCs, the expressions of EMT specific markers would increase, causing decreased adhesion and polarization of breast cancer cells (10). BMMSCs could also induce EMT effect in human hepatoma cells, leading to metastasis (11). Interestingly, growing evidences demonstrated that BMMSCs had an antitumor effect. To be more specific, Nakamizo suggested that human bone marrow-derived mesenchymal stem cells (hMSCs) could integrate into human gliomas after intravascular transfer, and such a tropism of hMSCs could significantly improve the survival rate of mice that were implanted with tumor (12). Lu also showed that MSCs could also inhibit the growth of tumor cell lines in mice in a number-dependent manner, even without host immunosuppression (13). Khakoo demonstrated that BMMSCs inhibited tumor growth in the mouse model of Kaposis sarcoma (14). Ohlsson found that BMMSCs inhibited the proliferation of rat colon cancer cell line H1D2 and promoted apoptosis (15).
To the best of our knowledge, sex hormones play an important role in the development of gastric cancer (16). Formerly, Furukawa demonstrated that male hormones facilitated carcinogenesis in the stomachs of rats (16). Yazawa injected BMMSCs labeled with green fluorescent protein into the testicles of 3-week-old developing rats, and found that a part of the injected BMMSCs could differentiate into Leydig cells and express various cell markers of Leydig cells (17,18). Zhang et al. suggested that androgen receptor could promote gastric cancer cell metastasis (19). However, the relations among BMMSCs, androgen and gastric cancer still remain to be defined.
In this study, we aim to investigate the relations among androgen, BMMSCs and gastric cancer and to explore the possible mechanism through mimicking internal microenvironment. Our study provides theoretical and practical basis to the prevention and treatment of gastric carcinoma.
Methods
Cell culture and co-culture SGC-7901 cells with BMMSCs
BMMSCs and gastric cancer SGC-7901 cells were obtained from American Type Culture Collection (ATCC; Manassas, VA, USA). The cells were cultured at 37 °C in 5% CO2 in RMPI-1640 medium (Gibco, USA), which contained 10% fetal bovine serum (Thermo Fisher, USA), 100 U/mL penicillin (Sigma-Aldrich, USA) and 100 µg/mL streptomycin (Invitrogen, USA). In some experiments, BMMSCs and SGC-7901 cells were respectively seeded on 6 and 96 well plates and incubated with 10 nmol/L androgens (Dr. Ehrenstorfer GmbH, Augsburg, Germany) with or without antagonists.
We established an upper and lower double-cell co-cultured system, in which BMMSCs (5×105 cell/mL) were seeded on the upper 0.4 µm transwell insert (Millipore) in RPMI 1640 medium in the absence or presence of 10 nmol/L androgens, while SGC-7901 cells (1×105 cell/mL) were cultured in the lower layer. In some experiments, the co-cultured system containing androgens added with or without antagonist.
Cell counting kit-8 (CCK-8) analysis
Cell viability was tested using CCK-8 (Meilunbio, Dalian, China). Corresponding cells above were all seeded on a 96-well culture plate at a density of 4×103 cells/well. Cell viabilities were tested at 12, 24 and 48 h after incubating the cells in a humidified incubator with 5% CO2 at 37 °C. Next, 10 µL CCK-8 solution was added to each well and held for 4 hours. Finally, the absorbance value was read at 450 nm using Thermo Multiskan FC (Thermo, USA).
Cell apoptosis analysis
Cell apoptosis was detected using Annexin V-FITC/PI Apoptosis Detection Kit (YEASEN, Shanghai, China). After a routine incubation in a 6-well culture plate for 48 h, adherent and suspended cells were harvested, washed twice with Phosphate Buffered Saline (PBS) and then resuspended with Binding Buffer. Subsequently, suspended cells were incubated for 10 min with Annexin V-FITC and propidium iodide (PI, Yeasen Biotechnology Co., Ltd., Shanghai, China) in a dark room for 15 min. Next, cell apoptosis assay was carried out by using FACScan flow cytometry (Becton Dickinson, Immunocytometry System, San Jose, CA).
Quantitative real-time PCR (qRT-PCR)
TRIZOL reagent (Invitrogen, California, USA) was used to extract total RNA collected from cells following the manufacturer’s protocol. The synthesis of cDNA was carried out using Prime Script RT reagent kit (Takara, Dalian, China), according to the instructions. The amplification reactions of TNF-α, Bcl-2, Bax and p53 were carried out using Thermo Power SYBR Green qRT-PCR Reagents Kit, and ABI 7500 thermocycler system (Applied Biosystems, California, USA) was used to analyze the results. Samples were ran by following cycling parameters: at 94 °C for 2 min, at 94 °C for 30 s, 35 cycles at 63 °C for 30 s, at 72 °C for 1 min, extended at 72 °C for 7 min and finally maintained at 4 °C. GAPDH was used as an internal control. The expressive discrepancy was calculated by 2−ΔΔCt method. The corresponding primers were purchased commercially (Invitrogen, USA) and sequences used were listed in Table 1.
Table 1
Gene | Primer | Sequence |
---|---|---|
TNF-α | Forward | 5'-TCTCAAAACTCGAGTGACAAG-3' |
Reverse | 5'-AGTTGGTTGTCTTTGAGATCC-3' | |
Bcl-2 | Forward | 5'-CGGGAGAACAGGGTATGA-3' |
Reverse | 5'-CAGGCTGGAAGGAGAAGAT-3' | |
Bax | Forward | 5'-CCGATTCATCTACCCTGCTG-3' |
Reverse | 5'-TGAGCAATTCCAGAGGCAGT-3' | |
P53 | Forward | 5'-GGAAATCTCACCCCATCCCA-3' |
Reverse | 5'-CAGTAAGCCAAGATCACGCC-3' | |
GAPDH | Forward | 5'-CACCCACTCCTCCACCTTTG-3' |
Reverse | 5'-CCACCACCCTGTTGCTGTAG-3' |
Western blotting assay
Total protein was extracted from the cells using RIPA Lysis Buffer (Beyotime, Shanghai, China). Protein quantification was performed by bicinchoninic acid (BCA) protein assay kit (Beyotime, Shanghai, China). Twelve percent SDS-PAGE (Beyotime, Shanghai; P0012A) was used to isolate the proteins, which were then transferred onto polyvinylidene fluoride (PVDF; Beyotime, Shanghai; FFP28) membranes. ECL (Amersham Pharmacia, Piscataway, NJ, USA) system was used to detect Chemiluminescence and Image J software was used for density analysis. Primary antibodies against TNF-α, Bcl-2, Bax, p-JNK, JNK and p53 were as follows: anti-TNF-α antibody (ab6671, 1:2,000, Abcam, USA), anti-Bcl-2 antibody (ab32124, 1:1,000, Abcam, USA), anti-Bax antibody (ab32503, 1:2,000, Abcam, USA), anti-P-JNK antibody (sc-6452, 1:1,000, Santa Cruz Biotechnology, USA), anti-JNK antibody (ab4821, 1:1000, Abcam, USA), anti-p53 antibody (ab131442, 1:1,000, Abcam, USA), anti-GAPDH antibody (ab9485, 1:2,500, Abcam, USA). HRP-conjugated goat anti-Rabbit IgG (ab6721, 1:2,000, Abcam, USA) were treated as secondary antibodies and antibodies against GAPDH served as an internal standard.
Statistical analysis
Statistical analysis was conducted by SPSS 22.0 software (Chicago, Illinois, USA). Data from 3 independent and repetitive experiments were presented as mean ± standard deviation. Comparisons between 2 sets of data were performed by using Student’s t-test, and the discrepancies in data among multiple groups were assessed by one-way analysis of variance (ANOVA). A P value <0.05 indicated a statistical significance.
Results
Androgen attenuates the effects of BMMSCs on cell proliferation and apoptosis in SGC-7901 cells
Cell viability and cell apoptosis was respectively performed to investigate the effects of BMMSCs on the proliferation and apoptosis of SGC-7901 cells and the roles of androgens. The results showed that BMMSCs decreased significantly SGC-7901 cell viability, and that Androgen promoted the cell proliferation at 24 and 48 h (P<0.05, Figure 1A). In the co-culture system of BMMSCs and SGC-7901 cells, adding androgen could notably increase the viability of SGC-7901 cells (P<0.05, Figure 1A). As Figure 1B shown, BMMSCs remarkably induced cell apoptosis (P<0.01), which was reduced by androgen (P<0.05). Compared to the co-culture system (21.05%), adding androgen (11.60%) could significantly decrease SGC-7901 cell apoptosis (P<0.01, Figure 1B,C). The results suggested that androgen partially reversed anti-tumor effect of BMMSCs on SGC-7901 cells.
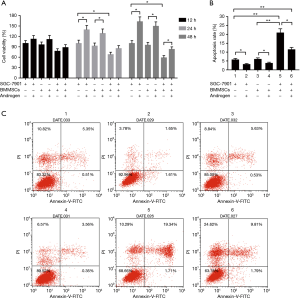
The effects of androgen-stimulated BMMSCs on the expressions of TNF-α, Bcl-2 and Bax in SGC-7901 cells
The tumor necrosis factor-α, which was shown to have an antitumor activity, apoptosis-related genes Bcl-2 and Bax were detected to further investigate the roles of androgen-stimulated BMMSCs on the regulation of SGC-7901 cells. Distinctly, at mRNA (Figure 2A,B,C) and protein (Figure 2D,E,F,G) levels, SGC-7901 cells co-cultured with BMMSCs showed higher expressions of TNF-α and Bax than cultured SGC-7901 cells alone (P<0.01). The results suggested that BMMSCs could increase the expressions of pro-apoptosis-related cytokines. Nevertheless, Bcl-2 expression levels in SGC-7901 cells co-cultured with BMMSCs were significantly reduced in comparison with that in cultured SGC-7901 cells (P<0.01). In addition, SGC-7901 with androgen and BMMSCs with androgen treatment attenuated the mRNA and protein levels of TNF-α and Bax and up-regulated Bcl-2 expression, compared with those without androgen treatment (P<0.05). The co-cultured system of SGC-7901 cells and BMMSCs added with androgen exhibited, to some extent, decreased expressions of TNF-α and Bax and increased expression of Bcl-2, compared with the group of SGC-7901 cells and BMMSCs (Bcl-2, P<0.01, others, P<0.05). The results suggested that androgen partially suppressed the expressions of pro-apoptosis-related cytokines.
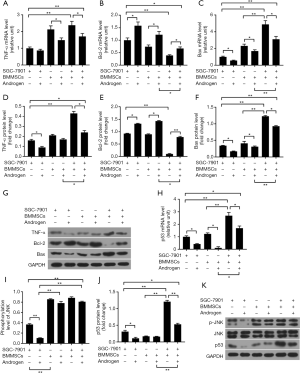
Androgen-stimulated BMMSCs restrain over-activation of JNK signaling
JNK signaling plays important roles in regulating biological activities, which could be activated by several factors, such as TNF-α and IL-6 (20). Therefore, JNK signaling was considered to help investigate the underlying mechanism among BMMSCs, androgen and SGC-7901 cells. We found that androgen significantly inhibited the expressions of phosphorylation-JNK (P<0.01) and p53 (P<0.05) in SGC-7901 cells (Figure 2H,I,J,K), however, BMMSCs increased the phosphorylation-JNK and p53 expression levels (P<0.01), indicating that BMMSCs could activate the JNK signaling. Then, SGC-7901 cells were co-cultured with BMMSCs and androgen, and we found that the expressions of p-JNK and p53 (mRNA, P<0.05, protein, P<0.01) were reduced, though p-JNK expression was reduced slightly.
Androgen antagonist partially reverses the elevated cell proliferation and suppresses cell apoptosis effects of androgen in co-cultured BMMSCs and SGC-7901 cells system
To validate the effects of BMMSCs with the induction of androgens on the viability and apoptosis of SGC-7901 cells, androgen antagonists were added to the cells. Cell viability was detected by CCK-8 assay and cell apoptosis analysis was carried out by flow cytometry. As Figure 3 shown, compared to the group containing androgen and its antagonist in SGC-7901, we found that BMMSCs inhibited SGC-7901 cell viability (P<0.05, Figure 3A) and induced apoptosis (from 6.97% to 20.45%, P<0.01, Figure 3B,C). In addition, cell viability in SGC-7901 cells co-cultured with BMMSCs, androgens and androgen antagonists were significantly inhibited at 48h, compared with SGC-7901 cells co-cultured with BMMSCs and androgens (P<0.05, Figure 3A), whereas the opposite tendency was observed in cell apoptosis rate of SGC-7901 cells (P<0.05, Figure 3B,C). The results revealed that androgen antagonists could partially reverse the negative effect of androgens on BMMSCs in SGC-7901 cells.
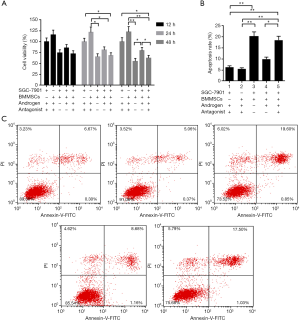
Androgen antagonist promotes antitumor effects through activating JNK signaling in co-cultured BMMSCs and SGC-7901 cells with androgen
To further verify the possible mechanism between BMMSCs and SGC-7901 cells, we added androgen antagonist in the co-cultured system, and the results confirmed that BMMSCs up-regulated the expressions of TNF-α (P<0.01) and Bax (P<0.01), and down-regulated the expression of Bcl-2 (P<0.01) at mRNA (Figure 4A,B,C) and protein levels (Figure 4D,E,F,G). Data also showed that SGC-7901 cells co-cultured with BMMSCs, androgens and androgen antagonists had higher expression levels of TNF-α and Bax than SGC-7901 cells co-cultured with BMMSCs and androgens (P<0.05). Nevertheless, Bcl-2 expression levels in SGC-7901 cells was the opposite to the situation above (P<0.01). Furthermore, the results also showed that androgen antagonist promoted the expressions of p-JNK (P<0.01) and p53 (P<0.05, Figure 4H,I,J,K), suggesting the activation of JNK signaling.
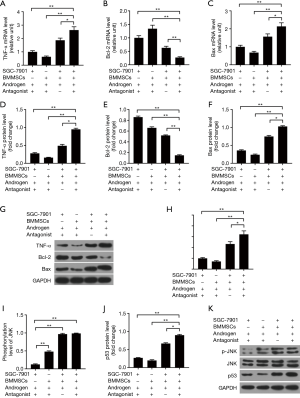
Discussion
The occurrence and metastasis of tumor are not only related to tumor cells themselves, but also to the surrounding environment of tumor cells (21). BMMSCs are one of the important components of tumor microenvironment. Over the recent years, a great number of studies have shown that mesenchymal stem cells had excellent migratory behavior and tumor tropism, and that their surface could express a variety of growth factor receptors, which can be directed to tumor sites by various cytokines in the tumor microenvironment, therefore regulating the growth of tumor cells (22-26). To investigate the effects of BMMSCs on the proliferation and apoptosis of SGC-7901 cells, we established a co-cultured system of SGC-7901 and BMMSCs in vitro. Noticeably, BMMSCs could noticeably inhibit SGC-7901 cell viability and induce cell apoptosis, though Klopp reported that co-injection with MSC in vivo could increase the growth of MDA-IBC-3 tumors and decrease the expression of EMT marker E-cadherin (27). Liu also demonstrated that BMMSCs could promote head and neck cancer (HNC) progression and induce EMT of HNC cells (the expressions of Snail, Twist, N-cadherin and Vimentin were increased and the expression of E-cadherin were decreased) (28), and such a phenomenon might be explained by the different tumor types. In addition, we also found that BMMSCs not only up-regulated the Bax/Bcl-2 expression, but also promoted TNF-α release. To the best of our knowledge, TNF-α is a cytokine with extensive biological activities produced by multinuclear giant cells. Krajewska previously suggested that mesenchymal stem cells had TNF receptors (29). TNF-α mediates the pathophysiological responses such as inflammation, tissue injury and shock. It regulates the immune function of the body and can make some tumor cells necrotic, of which one of the important mechanisms is the activation of JNK signaling (30-33).
At present, the relationship between JNK signaling pathway and tumor diseases is still controversial. Nateri et al. demonstrated when c-Jun gene was eliminated or Jun phosphorylation site was changed, the intestinal tumor cells of the mice would be reduced and the life span of the mice would be prolonged (34). Induction of liver cancer in mice with the chemical diethylnitrosamine revealed that JNK was permanently activated, whereas mice with JNK1 knockout had reduced sensitivity to diethylnitrosamine-induced liver cancer (35). However, Winn reported that in non-small cell lung cancer (NSCLC), the activation of JNK could inhibit tumor formation, maintain epithelial differentiation and inhibit the growth of variant cells (36). Yang suggested that quercetin could be used for the treatment of colorectal cancer carrying KRAS mutations via the activation of JNK pathway (37). Our findings showed that BMMSCs increased the phosphorylation level of JNK and the expression of down-stream target gene of JNK signaling, which is p53, in gastric cancer SGC-7901 cells. It is consistent with Wan’s finding that the inhibition of proliferation and invasion in gastric cancer HGC-27 cell was realized via activating of JNK and p38 signaling pathways (38).
The incidence of gastric cancer between men and women was significantly different, which could be explained by the fact that the incidence of gastric cancer in men is about twice as high as that in women and the cause is still unclear (39). Not surprisingly, sex hormones and their receptors are closely related to the tumorigenesis of prostate, breast and cervix cancers (40-43). Moreover, several studies reported that sex hormones could regulate non-sex hormone target organs of tumors. For example, Ma reported that targeting androgen receptor (AR) might be a useful in treating patients with early-stage hepatocellular carcinoma, however, androgen/AR signaling showed an ability to suppress the metastasis of hepatocellular carcinoma in patients with late-stage disease (44). Sukocheva et al. also demonstrated that androgen/AR was involved in the regulation of esophageal cancer growth (45). In the present study, we found that androgen contributed to the growth of gastric cancer cell. These results were in line with Zhang’s study, in which androgen receptor was demonstrated to be helpful to the migration and invasion of gastric cancer cells (19). In addition, we also found that androgen could attenuate the effects of promotion of cell viability and inhibition of cell apoptosis by BMMSCs in the co-cultured system of SGC-7901 and BMMSCs. Gondo et al. reported that BMMSCs co-cultured with Leydig cells could differentiate into Leydig cells in vitro and stably and continuously secrete a large amount of androgen (46). Therefore, decreased antitumor effects of BMMSCs in the co-cultured system and in combination with androgen were reasonable. However, after adding androgen antagonist in the co-cultured system of SGC-7901 and BMMSCs with androgen, BMMSCs could partially recover its antitumor effect on gastric cancer cells in vitro. Furthermore, the mechanism research confirmed that the effects of androgen or its antagonist on the antitumor regulation of BMMSCs were possibly realized through JNK signaling in SGC-7901 cells.
Conclusions
Collectively, this study showed the important roles of BMMSCs on the growth and apoptosis of gastric cancer cells in vitro. Additionally, in a mimicking microenvironment of the stomach, androgen weakened the antitumor effects of BMMSCs by limiting the JNK signaling activation. Thus, our findings suggest that androgen antagonist may be a promising adjuvant drug of BMMSCs in gastric cancer therapy.
Acknowledgments
Funding: This work was supported by
Footnote
Conflicts of Interest: All authors have completed the ICMJE uniform disclosure form (available at http://dx.doi.org/10.21037/tcr.2019.06.04). The authors have no conflicts of interest to declare.
Ethical Statement: The authors are accountable for all aspects of the work in ensuring that questions related to the accuracy or integrity of any part of the work are appropriately investigated and resolved.
Open Access Statement: This is an Open Access article distributed in accordance with the Creative Commons Attribution-NonCommercial-NoDerivs 4.0 International License (CC BY-NC-ND 4.0), which permits the non-commercial replication and distribution of the article with the strict proviso that no changes or edits are made and the original work is properly cited (including links to both the formal publication through the relevant DOI and the license). See: https://creativecommons.org/licenses/by-nc-nd/4.0/.
References
- Jemal A, Bray F, Center MM, et al. Global cancer statistics. CA Cancer J Clin 2011;61:69-90. [Crossref] [PubMed]
- Siegel R, Naishadham D, Jemal A. Cancer statistics, 2012. CA Cancer J Clin 2012;62:10-29. [Crossref] [PubMed]
- Yang L. Incidence and mortality of gastric cancer in China. World J Gastroenterol 2006;12:17-20. [Crossref] [PubMed]
- Kemp KC, Hows J, Donaldson C. Bone marrow-derived mesenchymal stem cells. Leuk Lymphoma 2005;46:1531-44. [Crossref] [PubMed]
- Pittenger MF, Mackay AM, Beck SC, et al. Multilineage potential of adult human mesenchymal stem cells. Science 1999;284:143-7. [Crossref] [PubMed]
- Pratheesh MD, Gade NE, Katiyar AN, et al. Isolation, culture and characterization of caprine mesenchymal stem cells derived from amniotic fluid. Res Vet Sci 2013;94:313-9. [Crossref] [PubMed]
- Wu Y, Chen L, Scott PG, et al. Mesenchymal stem cells enhance wound healing through differentiation and angiogenesis. Stem Cells 2007;25:2648-59. [Crossref] [PubMed]
- Zhang T, Lee YW, Rui YF, et al. Bone marrow-derived mesenchymal stem cells promote growth and angiogenesis of breast and prostate tumors. Stem Cell Res Ther 2013;4:70. [Crossref] [PubMed]
- Tu B, Du L, Fan QM, et al. STAT3 activation by IL-6 from mesenchymal stem cells promotes the proliferation and metastasis of osteosarcoma. Cancer Lett 2012;325:80-8. [Crossref] [PubMed]
- Giannoni E, Parri M, Chiarugi P. EMT and oxidative stress: a bidirectional interplay affecting tumor malignancy. Antioxid Redox Signal 2012;16:1248-63. [Crossref] [PubMed]
- Jing Y, Han Z, Liu Y, et al. Mesenchymal stem cells in inflammation microenvironment accelerates hepatocellular carcinoma metastasis by inducing epithelial-mesenchymal transition. PLoS One 2012;7:e43272. [Crossref] [PubMed]
- Nakamizo A, Marini F, Amano T, et al. Human bone marrow-derived mesenchymal stem cells in the treatment of gliomas. Cancer Res 2005;65:3307-18. [Crossref] [PubMed]
- Lu YR, Yuan Y, Wang XJ, et al. The growth inhibitory effect of mesenchymal stem cells on tumor cells in vitro and in vivo. Cancer Biol Ther 2008;7:245-51. [Crossref] [PubMed]
- Khakoo AY, Pati S, Anderson SA, et al. Human mesenchymal stem cells exert potent antitumorigenic effects in a model of Kaposi's sarcoma. J Exp Med 2006;203:1235-47. [Crossref] [PubMed]
- Ohlsson LB, Varas L, Kjellman C, et al. Mesenchymal progenitor cell-mediated inhibition of tumor growth in vivo and in vitro in gelatin matrix. Exp Mol Pathol 2003;75:248-55. [Crossref] [PubMed]
- Furukawa H, Iwanaga T, Koyama H, et al. Effect of sex hormones on carcinogenesis in the stomachs of rats. Cancer Res 1982;42:5181-2. [PubMed]
- Yazawa T, Mizutani T, Yamada K, et al. Differentiation of adult stem cells derived from bone marrow stroma into Leydig or adrenocortical cells. Endocrinology 2006;147:4104-11. [Crossref] [PubMed]
- Lue Y, Erkkila K, Liu PY, et al. Fate of bone marrow stem cells transplanted into the testis: potential implication for men with testicular failure. Am J Pathol 2007;170:899-908. [Crossref] [PubMed]
- Zhang BG, Du T, Zang MD, et al. Androgen receptor promotes gastric cancer cell migration and invasion via AKT-phosphorylation dependent upregulation of matrix metalloproteinase 9. Oncotarget 2014;5:10584-95. [PubMed]
- Sadeghi A, Rostamirad A, Seyyedebrahimi S, et al. Curcumin ameliorates palmitate-induced inflammation in skeletal muscle cells by regulating JNK/NF-kB pathway and ROS production. Inflammopharmacology 2018;26:1265-72. [Crossref] [PubMed]
- Abarrategi A, Marinas-Pardo L, Mirones I, et al. Mesenchymal niches of bone marrow in cancer. Clin Transl Oncol 2011;13:611-6. [Crossref] [PubMed]
- Cuiffo BG, Karnoub AE. Mesenchymal stem cells in tumor development: emerging roles and concepts. Cell Adh Migr 2012;6:220-30. [Crossref] [PubMed]
- D’souza N, Burns JS, Grisendi G, et al. MSC and Tumors: Homing, Differentiation, and Secretion Influence Therapeutic Potential. Adv Biochem Eng Biotechnol 2013;130:209-66. [Crossref] [PubMed]
- Bergfeld SA, DeClerck YA. Bone marrow-derived mesenchymal stem cells and the tumor microenvironment. Cancer Metastasis Rev 2010;29:249-61. [Crossref] [PubMed]
- Stagg J. Mesenchymal stem cells in cancer. Stem Cell Rev 2008;4:119-24. [Crossref] [PubMed]
- Yang X, Hou J, Han Z, et al. One cell, multiple roles: contribution of mesenchymal stem cells to tumor development in tumor microenvironment. Cell Biosci 2013;3:5. [Crossref] [PubMed]
- Klopp AH, Lacerda L, Gupta A, et al. Mesenchymal stem cells promote mammosphere formation and decrease E-cadherin in normal and malignant breast cells. PLoS One 2010;5:e12180. [Crossref] [PubMed]
- Liu C, Feng X, Wang B, et al. Bone marrow mesenchymal stem cells promote head and neck cancer progression through Periostin-mediated phosphoinositide 3-kinase/Akt/mammalian target of rapamycin. Cancer Sci 2018;109:688-98. [Crossref] [PubMed]
- Krajewska M, Krajewski S, Zapata JM, et al. TRAF-4 expression in epithelial progenitor cells. Analysis in normal adult, fetal, and tumor tissues. Am J Pathol 1998;152:1549-61. [PubMed]
- Vural P, Degirmencioglu S, Saral NY, et al. Tumor necrosis factor alpha (-308), interleukin-6 (-174) and interleukin-10 (-1082) gene polymorphisms in polycystic ovary syndrome. Eur J Obstet Gynecol Reprod Biol 2010;150:61-5. [Crossref] [PubMed]
- Singh U, Kumar A, Sinha R, et al. Calreticulin transacetylase catalyzed modification of the TNF-alpha mediated pathway in the human peripheral blood mononuclear cells by polyphenolic acetates. Chem Biol Interact 2010;185:263-70. [Crossref] [PubMed]
- Giatromanolaki A, Sivridis E, Koukourakis MI, et al. Prognostic role of angiogenesis in operable carcinoma of the gallbladder. Am J Clin Oncol 2002;25:38-41. [Crossref] [PubMed]
- Rosette C, Karin M. Ultraviolet light and osmotic stress: activation of the JNK cascade through multiple growth factor and cytokine receptors. Science 1996;274:1194-7. [Crossref] [PubMed]
- Nateri AS, Spencer-Dene B, Behrens A. Interaction of phosphorylated c-Jun with TCF4 regulates intestinal cancer development. Nature 2005;437:281-5. [Crossref] [PubMed]
- Sakurai T, Maeda S, Chang L, et al. Loss of hepatic NF-kappa B activity enhances chemical hepatocarcinogenesis through sustained c-Jun N-terminal kinase 1 activation. Proc Natl Acad Sci U S A 2006;103:10544-51. [Crossref] [PubMed]
- Winn RA, Marek L, Han SY, et al. Restoration of Wnt-7a expression reverses non-small cell lung cancer cellular transformation through frizzled-9-mediated growth inhibition and promotion of cell differentiation. J Biol Chem 2005;280:19625-34. [Crossref] [PubMed]
- Yang Y, Wang T, Chen D, et al. Quercetin preferentially induces apoptosis in KRAS-mutant colorectal cancer cells via JNK signaling pathways. Cell Biol Int 2019;43:117-24. [Crossref] [PubMed]
- Wan X, Shi L, Ma X, et al. The Elevated ASK1 Expression Inhibits Proliferation and Invasion in Gastric Cancer HGC-27 Cells. Anat Rec (Hoboken) 2018;301:1815-9. [Crossref] [PubMed]
- Bray F, Ferlay J, Soerjomataram I, et al. Global cancer statistics 2018: GLOBOCAN estimates of incidence and mortality worldwide for 36 cancers in 185 countries. CA Cancer J Clin 2018;68:394-424. [Crossref] [PubMed]
- Feng J, Li L, Zhang N, et al. Androgen and AR contribute to breast cancer development and metastasis: an insight of mechanisms. Oncogene 2017;36:2775-90. [Crossref] [PubMed]
- Kong Z, Wan X, Zhang Y, et al. Androgen-responsive circular RNA circSMARCA5 is up-regulated and promotes cell proliferation in prostate cancer. Biochem Biophys Res Commun 2017;493:1217-23. [Crossref] [PubMed]
- Folkerd E, Dowsett M. Sex hormones and breast cancer risk and prognosis. Breast 2013;22:S38-43. [Crossref] [PubMed]
- Hellberg D. Sex steroids and cervical cancer. Anticancer Res 2012;32:3045-54. [PubMed]
- Ma WL, Lai HC, Yeh S, et al. Androgen receptor roles in hepatocellular carcinoma, fatty liver, cirrhosis and hepatitis. Endocr Relat Cancer 2014;21:R165-82. [Crossref] [PubMed]
- Sukocheva OA, Li B, Due SL, et al. Androgens and esophageal cancer: What do we know? World J Gastroenterol 2015;21:6146-56. [Crossref] [PubMed]
- Gondo S, Okabe T, Tanaka T, et al. Adipose tissue-derived and bone marrow-derived mesenchymal cells develop into different lineage of steroidogenic cells by forced expression of steroidogenic factor 1. Endocrinology 2008;149:4717-25. [Crossref] [PubMed]