Validation of the diagnostic efficiency of folate receptor-positive circulating tumor cells in lung cancers: a prospective observational study
Introduction
Thoracic cancers, especially for lung cancers, are the leading causes of cancer mortality in developed countries (1). In China, lung cancer is the most lethal malignant disease, causing over 600,000 of deaths in 2015 (2). The 5-year relative survival rate of thoracic cancers was dismal, with only 15.8–19.7% for lung cancers (3). This could be partly due to the late diagnosis (4).
Effective cancer screening method would help improve the situation (5,6). Since the National Lung Screening Trial reported that lung cancer screening in the high-risk population with low-dose computed tomography (LDCT) can reduce the cancer-related mortality by 20% compared to chest X-ray (7), LDCT has become the standard method for lung cancer screening. However, the high false-positive rate (8,9), high cost for follow-up (10), and repeated radiation exposure have limited the widespread application of LDCT. Therefore, a more reliable and non-invasive method that can be used in parallel with LDCT assessment to improve the diagnostic performance is urgently warranted.
Circulating tumor cells (CTCs) are cells that shed from the tumor and entered the bloodstream. As a source of liquid biopsy, CTC is a potential biomarker for non-invasive cancer diagnosis, prediction of treatment efficacy, and monitoring disease progression (11). Indeed, numerous researches have already proved the clinical value of CTC in lung cancer management (12-14). There are currently several major techniques for CTC enumeration and analysis, which include immunomagnetic-based capture, size-based filtration, microfluidic-based separation, etc. Each technique has its strength and weakness (15). For example, CellSearch, the only FDA-cleared platform for CTC enumeration, captures CTCs through immunomagnetic nanoparticles with antibodies that target epithelial cell adhesion molecules (EpCAM) and detects CTCs using fluorescent immunostaining assay (16). As a positive enrichment method, CellSearch has a high specificity in identifying CTCs. However, it cannot detect CTCs that lack EpCAM expression, which greatly hampered its application to cancer types that have a low EpCAM expression level.
Folate receptor (FR) could be a possible substitute to label and detect CTCs. folate receptor alpha (FRα), a subtype of FR, is highly expressed in lung adenocarcinoma tissues but rarely observed in other blood cells (17,18), making it an ideal target for CTC detection. Several studies had already reported the clinical significance of FR+CTC in lung cancer diagnosis, with sensitivity and specificity ranging from 72.5% to 81.8% and 82.4% to 93.2%, respectively (19-22). The current study aims to validate the feasibility of FR+CTC analysis in the diagnosis of lung cancers.
Methods
Study design
This was a prospective study conducted at The Affiliated Wuxi No. 2 People’s Hospital of Nanjing Medical University and the Shanghai Pulmonary Hospital Affiliated to Tongji University School of Medicine. Seventy-five lung cancer patients were enrolled from January 2015 to March 2018. All cancer patients were confirmed by histopathological examination. None of the patients had received any anti-cancer treatment before the study or had a prior cancer history within 5 years. The International Association for the Study of Lung Cancer 7th Tumor, Node, Metastasis Staging System was used for clinical disease staging. In addition, 48 patients with benign lung disease (diagnosed based on multidisciplinary examination including imaging, laboratory tests, and lung biopsy) and 23 healthy subjects (individuals who exhibited no evidence of any clinically detectable disease after health checkups) were recruited. The Ethics Committees of participating institutes have approved the study (approval number: 2014W-12). Written informed consent was obtained from all the participants.
FR+CTC analysis
Three milliliters of the whole blood sample was collected with 4 mL EDTA anticoagulant tubes (REF 367861, Beckman Dickson, NJ, USA). Samples were temporarily stored at 4–10 °C and processed within 24 hours after blood withdrawal. FR+CTC enumeration was performed using “CytoploRare Detection Kit” provided by Genosaber Biotech Co., Ltd. (Shanghai, China) according to the manufacturer’s instruction. In brief, erythrocytes were lysed and CTCs were negatively enriched by immunomagnetic depletion with leukocyte-specific magnetic beads. Next, CTCs were labeled with a tumor-specific probe which is a folic acid conjugated to a synthetic oligonucleotide sequence. The folic acid was used as a ligand to target the tumor-specific FRα, and the synthetic oligonucleotide was used as a template for CTC enumeration. Quantitative polymerase chain reaction (PCR) was then performed to enumerate FR+CTC in the samples. A serial of standards containing 10−14 to 10−9 M oligonucleotides (corresponding to 2 to 2×105 CTC units/3 mL blood), was used for FR+CTC quantification.
Analysis of routine serum biomarkers
An extra 3 mL of peripheral blood was collected from all cancer and benign lung disease patients for tumor biomarker analysis. Well-established tumor biomarkers for thoracic cancers, including carcinoembryonic antigen (CEA), cytokeratin fragment 19 (CYFRA21-1), and neuron-specific enolase (NSE), were analyzed by flow fluorescence assay (Tellgen, Shanghai, China) on the day of sample collection.
Statistical analysis
The comparison of FR+CTC levels between two groups was performed using the Mann-Whitney U test. The diagnostic efficiency of FR+CTC and biomarkers were analyzed using receiver operating characteristic (ROC) curve analysis. Youden index was calculated to determine the optimal cut-off thresholds to discriminate cancer patients from non-malignant participants. All P values were based on two-sided testing, with a value less than 0.05 indicating a statistically significant difference. Statistical analyses were performed with the Prism 5.0 (GraphPad Software Inc, San Diego, CA, USA).
Results
Patients’ characteristic
Seventy-five lung cancer patients and 71 non-malignant participants were enrolled in this study (Table 1). Non-malignant participants included 48 benign lung disease patients and 23 healthy subjects, in which benign lung disease included bronchiectasis (n=9), chronic obstructive pulmonary diseases (n=2), connective tissue disease (n=1), interstitial lung diseases (n=4), lung infection (n=1), pulmonary cryptococcosis (n=1), pulmonary inflammatory diseases (n=4), and pulmonary tuberculosis (n=26).
Table 1
Parameters | Lung cancer | Benign lung disease | Healthy subjects |
---|---|---|---|
Number of patients | 75 | 48 | 23 |
Gender | |||
Male | 41 | 23 | 6 |
Female | 34 | 25 | 17 |
Age, median [range] | 61 [34–80] | 53.5 [23–77] | 34 [22–54] |
Clinical stage | |||
I | 25 | – | – |
II | 9 | – | – |
III | 24 | – | – |
IV | 10 | – | – |
Uncertain | 7 | – | – |
Pathological subtype | |||
Adenocarcinoma | 45 | – | – |
Squamous cell carcinoma | 15 | – | – |
Small cell lung cancer | 3 | – | – |
Others† | 6 | – | – |
Uncertain | 6 | – | – |
†, other pathological subtypes included 3 non-small cell lung cancer not otherwise specified, 2 large cell carcinoma, and 1 neuroendocrine carcinoma.
FR+CTC expression level
The median FR+CTC levels of lung cancer patients and non-malignant participants were 10.5 and 5.9 CTC units/3 mL blood (mean: 20.0 and 6.0 CTC units/3 mL blood), respectively (Figure 1A). For benign lung disease patients and healthy subjects, the corresponding median FR+CTC levels were 6.5 and 4.9 CTC units/3 mL blood (mean: 6.4 and 5.0 CTC units/3 mL blood). The FR+CTC level of lung cancer patients was significantly higher than that of the control group (P<0.0001). For patients with early-stage (stage I) lung cancer, the median FR+CTC level was 10.3 CTC units/3 mL blood. The FR+CTC level of stage I lung cancer patients was significantly higher than that of the control group (P<0.0001, Figure 1B).
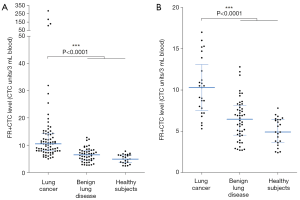
For all lung cancer patients, no significant difference in FR+CTC level was observed between patients with different age (<60 versus ≥60 years, P=0.1980, Figure 2A), gender (male versus female, P=0.5799, Figure 2B), and pathological subtype (adenocarcinoma versus squamous cell carcinoma, P=0.1330, Figure 2C). However, stage IV lung cancers showed a significantly higher FR+CTC level compared to stage I–III lung cancers (P=0.0169, Figure 2D).
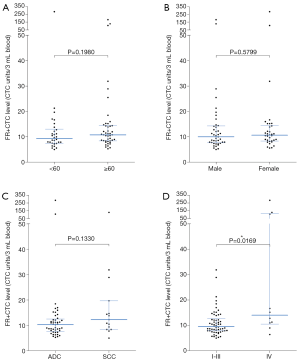
ROC analysis
With 7.9 CTC units/3 mL blood as the cut-off threshold, FR+CTC showed a sensitivity of 78.7% and specificity of 81.7% in the diagnosis of lung cancers (AUC =0.8746, 95% CI: 0.8199–0.9293, P<0.0001, Figure S1A). For stage I lung cancer (n=25), the sensitivity and specificity of FR+CTC were 68.0% and 90.1%, respectively, with 8.7 CTC units/3 mL blood as the cut-off threshold (AUC =0.8592, 95% CI: 0.7777–0.9406, P<0.0001, Figure S1B).
Comparison of the diagnostic performance of FR+CTC with routine serum biomarkers
The sensitivity and specificity of FR+CTC in differentiating lung cancers from benign lung diseases were compared with that of other clinical available tumor biomarkers which include CEA, CYFRA21-1, and NSE. As shown in Table 2, the sensitivity of FR+CTC was the highest among all tested biomarkers (Figure 3A), although the specificity was relatively lower than other biomarkers (Figure 3B). For stage I lung cancer, the sensitivity of FR+CTC was superior over other tumor biomarkers.
Table 2
Biomarkers | Cut-off threshold | Sensitivity | Specificity | Stage I lung cancer sensitivity |
---|---|---|---|---|
FR+CTC | 7.9 CTC units/3 mL blood | 78.7% (59/75) | 81.7% (58/71) | 68.0%† (17/25) |
CEA | 5.2 ng/mL | 24.0% (18/75) | 93.8% (45/48) | 4.0% (1/25) |
CYFRA21-1 | 3.3 ng/mL | 48.0% (36/75) | 85.4% (41/48) | 32.0% (8/25) |
NSE | 16.3 ng/mL | 16.0% (12/75) | 85.4% (41/48) | 8.0% (2/25) |
†, for stage I lung cancer, 8.7 CTC units/3 mL blood was used as the cut-off threshold. FR+CTC, folate receptor-positive circulating tumor cell; CEA, carcinoembryonic antigen; CYFRA21-1, cytokeratin fragment 19; NSE, neuron-specific enolase.
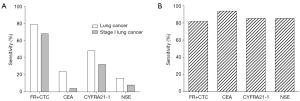
Discussion
To improve the prognosis and quality of life of thoracic cancer patients, the development of an effective screening method is needed so that patients could benefit from early diagnosis and in-time treatment (5). In this study, we showed that FR+CTC is a possible biomarker to assist in the diagnosis of lung cancers, with high sensitivity (78.7%) and specificity (81.7%). The results were in concordance with the previous studies (19-22). It should be noted that the detection platform used in our study is PCR-based. Background noise could lead to the virtual positive value which did not exactly represent the presence of CTCs. Only CTC levels higher than the determined cut-off value (7.9 CTC units/3 mL blood) are considered to be positive for CTC. FR+CTC levels were not associated with clinical factors such as age, gender, and pathological subtype. However, stage IV lung cancers showed a significantly higher FR+CTC level compared to stage I–III lung cancers, probably due to the higher tumor burden in metastatic diseases. Notably, the diagnostic efficiency of FR+CTC in stage I lung cancer (AUC =0.8592) was comparable to that of all-stage lung cancer (AUC =0.8746), indicated that FR+CTC was released into and existed in the circulation at the early stage of lung cancer. This is particularly favorable for cancer screening as the ability of the biomarker to determine malignancy is not limited by cancer stage.
Compared to CellSearch, FR+CTCs showed advantages in lung cancers (Table 3). According to a previous study, the detection rate of CellSearch in advanced lung cancer was only 22.5% (23). This is probably due to the high frequency of epithelial-to-mesenchymal transition in lung cancer, which leads to the loss of EpCAM expression in cancer cells (24,25). In contrast, the performance of FR+CTC in lung cancer diagnosis was superior in this study. The detection rate of FR+CTC (78.7%) was comparable to that of Isolation by Size of Tumor Cells method (80%, a size-based filtration method) (23).
Table 3
Features | CellSearch (16) | CytoploRare Detection Kit |
---|---|---|
Required blood sample volume | 7.5 mL | 3 mL |
Principle of CTC enrichment | EpCAM-based positive enrichment | Negative enrichment |
Detection of CTC | Fluorescent immunostaining (CD45−, cytokeratin+, DAPI−) | Ligand-targeted PCR (FRα) |
Clinical application | FDA-approved for assessment of prognosis of patients with metastatic breast, colorectal, and prostate cancer | CFDA-approved for assisting in diagnosis and management of lung cancer |
Detection rate in lung cancer | 22.5% (23) | 78.7% |
CTC, circulating tumor cell; PCR, polymerase chain reaction; FRα, folate receptor alpha.
Compared to routine serum biomarkers, such as CEA, CYFRA21-1, and NSE, the sensitivity of FR+CTC was the highest (78.7%) in lung cancers. The sensitivity of FR+CTC slightly decreased in stage I lung cancer (68.0%), but the performance was still superior over other tumor biomarkers. In previous studies, FR+CTC in combination with the above-mentioned serum biomarkers can significantly improve the diagnostic efficiency of lung cancer (21,22). Unfortunately, the exact values of serum biomarker expression level for the negative cases were not recorded in this study and thus the corresponding analysis cannot be carried out.
Conclusions
In conclusion, this pilot study validated the performance of FR+CTC quantification in lung cancer diagnosis. FR+CTC detection can be used to assist LDCT in early-stage thoracic cancer diagnosis.
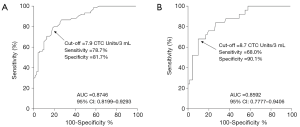
Acknowledgments
Funding: None.
Footnote
Conflicts of Interest: All authors have completed the ICMJE uniform disclosure form (available at http://dx.doi.org/10.21037/tcr.2019.07.10). The authors have no conflicts of interest to declare.
Ethical Statement: The authors are accountable for all aspects of the work in ensuring that questions related to the accuracy or integrity of any part of the work are appropriately investigated and resolved. The study was conducted in accordance with the Declaration of Helsinki (as revised in 2013). The Ethics Committees of participating institutes have approved the study (approval number: 2014W-12). Written informed consent was obtained from all the participants.
Open Access Statement: This is an Open Access article distributed in accordance with the Creative Commons Attribution-NonCommercial-NoDerivs 4.0 International License (CC BY-NC-ND 4.0), which permits the non-commercial replication and distribution of the article with the strict proviso that no changes or edits are made and the original work is properly cited (including links to both the formal publication through the relevant DOI and the license). See: https://creativecommons.org/licenses/by-nc-nd/4.0/.
References
- Siegel RL, Miller KD, Jemal A. Cancer statistics, 2016. CA Cancer J Clin 2016;66:7-30. [Crossref] [PubMed]
- Chen W, Zheng R, Baade PD, et al. Cancer statistics in China, 2015. CA Cancer J Clin 2016;66:115-32. [Crossref] [PubMed]
- Zeng H, Chen W, Zheng R, et al. Changing cancer survival in China during 2003-15: a pooled analysis of 17 population-based cancer registries. Lancet Glob Health 2018;6:e555-67. [Crossref] [PubMed]
- Burki TK. Late detection of lung cancer. Lancet Oncol 2014;15:e590. [Crossref] [PubMed]
- Hong QY, Wu GM, Qian GS, et al. Prevention and management of lung cancer in China. Cancer 2015;121:3080-8. [Crossref] [PubMed]
- Zou XN. Epidemic trend, screening, and early detection and treatment of cancer in Chinese population. Cancer Biol Med 2017;14:50-9. [Crossref] [PubMed]
- National Lung Screening Trial Research Team. Reduced lung-cancer mortality with low-dose computed tomographic screening. N Engl J Med 2011;365:395-409. [Crossref] [PubMed]
- Nahorecki A, Chabowski M, Kuźniar T, et al. Low-dose computer tomography as a screening tool for lung cancer in a high risk population. Adv Exp Med Biol 2015;852:31-7. [Crossref] [PubMed]
- Fu C, Liu Z, Zhu F, et al. A meta-analysis: is low-dose computed tomography a superior method for risky lung cancers screening population? Clin Respir J 2016;10:333-41. [Crossref] [PubMed]
- Goulart BH, Bensink ME, Mummy DG, et al. Lung cancer screening with low-dose computed tomography: costs, national expenditures, and cost-effectiveness. J Natl Compr Canc Netw 2012;10:267-75. [Crossref] [PubMed]
- Mateo J, Gerlinger M, Rodrigues DN, et al. The promise of circulating tumor cell analysis in cancer management. Genome Biol 2014;15:448. [Crossref] [PubMed]
- Zhong CH, Tong D, Zhou ZQ, et al. Performance evaluation of detecting circulating tumor cells in bronchoalveolar lavage fluid in diagnosis of peripheral lung cancer. J Thorac Dis 2018;10:S830-7. [Crossref] [PubMed]
- Pailler E, Oulhen M, Borget I, et al. Circulating tumor cells with aberrant ALK copy number predict progression-free survival during crizotinib treatment in ALK-rearranged non-small cell lung cancer patients. Cancer Res 2017;77:2222-30. [Crossref] [PubMed]
- Morabito A, Sandomenico C, Costanzo R, et al. Positron emission tomography and circulating tumor cells to monitor a dramatic response to gefitinib. J Thorac Oncol 2012;7:e27-8. [Crossref] [PubMed]
- Krebs MG, Metcalf RL, Carter L, et al. Molecular analysis of circulating tumor cells-biology and biomarkers. Nat Rev Clin Oncol 2014;11:129-44. [Crossref] [PubMed]
- Cristofanilli M, Budd GT, Ellis MJ, et al. Circulating tumor cells, disease progression, and survival in metastatic breast cancer. N Engl J Med 2004;351:781-91. [Crossref] [PubMed]
- Driver BR, Barrios R, Ge Y, et al. Folate receptor α expression level correlates with histologic grade in lung adenocarcinoma. Arch Pathol Lab Med 2016;140:682-5. [Crossref] [PubMed]
- He W, Kularatne SA, Kalli KR, et al. Quantitation of circulating tumor cells in blood samples from ovarian and prostate cancer patients using tumor-specific fluorescent ligands. Int J Cancer 2008;123:1968-73. [Crossref] [PubMed]
- Yu Y, Chen Z, Dong J, et al. Folate receptor-positive circulating tumor cells as a novel diagnostic biomarker in non-small cell lung cancer. Transl Oncol 2013;6:697-702. [Crossref] [PubMed]
- Lou J, Ben S, Yang G, et al. Quantification of rare circulating tumor cells in non-small cell lung cancer by ligand-targeted PCR. PLoS One 2013;8:e80458. [Crossref] [PubMed]
- Chen X, Zhou F, Li X, et al. Folate receptor-positive circulating tumor cell detected by LT-PCR-based method as a diagnostic biomarker for non-small-cell lung cancer. J Thorac Oncol 2015;10:1163-71. [Crossref] [PubMed]
- Wang L, Wu C, Qiao L, et al. Clinical significance of folate receptor-positive circulating tumor cells detected by ligand-targeted polymerase chain reaction in lung cancer. J Cancer 2017;8:104-10. [Crossref] [PubMed]
- Krebs MG, Hou JM, Sloane R, et al. Analysis of circulating tumor cells in patients with non-small cell lung cancer using epithelial marker-dependent and –independent approaches. J Thorac Oncol 2012;7:306-15. [Crossref] [PubMed]
- Lecharpentier A, Vielh P, Perez-Moreno P, et al. Detection of circulating tumor cells with a hybrid (epithelial/mesenchymal) phenotype in patients with metastatic non-small cell lung cancer. Br J Cancer 2011;105:1338-41. [Crossref] [PubMed]
- Gorges TM, Tinhofer I, Drosch M, et al. Circulating tumor cells escape from EpCAM-based detection due to epithelial-to-mesenchymal transition. BMC Cancer 2012;12:178. [Crossref] [PubMed]