Co-existence of myeloproliferative neoplasias and β-thalassemia with IVS-2-654 mutation—a case report
Introduction
Beta-thalassemia (β-thalassemia) is characterized by abnormal synthesis of the hemoglobin subunit beta (hemoglobin beta chain), resulting in anemia in different degrees (1). Essential thrombocythemia (ET) is a kind of myeloproliferative neoplasias (MPNs), characterized by clonal proliferation of megakaryocytes in the bone marrow and high platelet counts in peripheral blood (2). The Janus kinase 2 gene (JAK2) mutation screening is a diagnostic strategy for ET recommended by the World Health Organization (WHO). The concomitancy of MPNs and β-thalassemia are exceptionally unusual. To date, only several cases of concurrent β-thalassemia and polycythemia vera (PV) were reported (3-5). ET in β-thalassaemic patients has not been previously described, as we have seen, it’s the first time that coexistence of β-thalassemia and ET worldwide in clinical practice was reported. We present the following case in accordance with the CARE Guideline.
Case presentation
A 24-year-old female with a chipmunk face, presenting with slight weakness, previously was diagnosed as moderate β-thalassemia and underwent splenectomy in childhood. She was evaluated as a lifelong history of anemia and thrombocytosis. Data of genetic test was shown in Table 1, the mutant site of the beta-globin gene is in IVS-2-654. The hemogram of the patient showed moderate anemia with 3.61× 1012 red cells per litre (normal female range, 3.8–5.1×1012/L) and decreased hemoglobin level, 85 g/L (normal range, 115–150 g/L). The mean corpuscular volume was 78.6 fL (normal, 82–100 fL), and the mean hemoglobin mass was 23.7 pg (normal, 27–34 pg) in microcytosis. The density of white blood cells was about 9.6×109/L (normal, 3.5–9.5 ×109/L) and the platelet density was about 493×109/L (normal, 125–350 ×109/L). In the following days, her platelet count rose to 650×109/L. Erythrocyte sedimentation rate and C-reactive protein level were normal; no clinical infection was detected. Thus, it reminded us that the disease was possibly caused by myeloproliferative disorder. To confirm that, we performed a bone marrow biopsy. The result showed that megakaryocytes proliferated prominently, while neutrophil granulopoiesis and mild bone marrow reticulin fibrosis fluctuate at a normal level, as shown in Figure 1. Conventional cytogenetics revealed normal karyotype, and molecular analysis showed absence of BCR-ABL transcript and presence of JAK2V617F mutation. It was diagnosed as the low-risk ET according to the 2016 WHO Diagnostic Criteria and the revised International Prognostic Score of Thrombosis for ET (IPSET-thrombosis). In addition, we found three novel genes mutations EP300 (c.1519A>G; p.S507G, 47.0%), CUX1 (c.328G>A; p.D110N, 51.1%), and FGFR3 (c.1738G>A; p.D580N, 47.0%) (Table 2) after screening 111 genes in next-generation sequencing.
Table 1
Test object | Test result |
---|---|
α-globin gene deletion | Negative |
α-globin gene mutation | Negative |
β-globin gene mutation | IVS-2-654 heterozygous mutation |
Detection 3 α- globin gene deletion: --SEA, -α3.7, -α4.2; Detection 3 α- globin gene mutation: WS122, QS125, CS142; Detection 17 β- globin gene mutation: CD41-42, IVS-2-654, CD17, -28, CD26, CD71-72, CD43, -29, Int, CD14-15, CD27-28, -32, -30, IVS-1-1, IVS-1-5, CD31, Cap.
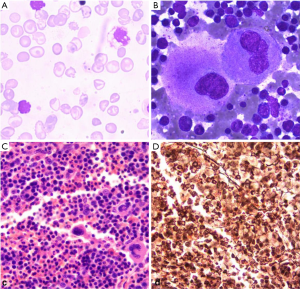
Table 2
Gene mutation | Transcript ID | Mutation site | Nucleotide | Amino acid | dbSNP | Mutation frequency |
---|---|---|---|---|---|---|
EP300 | NM-001429 | Exon6 | c.1519A>G | p.S507G | Rs146242251 | 50.6% |
FGFR3 | NM-000142 | Exon13 | c.1738G>A | p.D580N | 47.1% | |
CUX1 | NM-181552 | Exon5 | c.328G>A | p.D110N | 50.1% |
Detection all exon regions of 111 genes with NGS, showing 3 types of gene mutation. NGS, Next generation sequencing.
The following detection showed that her hemoglobin maintained between 75 and 90 g/L, platelet counts ranged from 465×109/L to 750×109/L. Further detection showed the ferritin rose up to 3,501 µg/L, and the folic acid reduced to 1.03 µg/l. The patient was treated with folic acid supplementation and Iron-chelating therapy (deferasirox, 20 mg/kg). According to NCCN Guidelines, for the low-risk ET, it can just observation, does not need aspirin or cytoreductive therapy. Until now, there are no thrombotic events, or disease-related bleeding happening to the patient.
Discussion
Mutation in IVS-2-654 was reported as a common characteristic of beta-thalassemia in Chinese (6). A research group investigated the status of thalassemia in Guangxi, China. The study showed that IVS-2-654 mutation accounted for 5.8% of β-thalassemia (7). Another study found Cd 41/42 (-TTCT) and IVS 2-654 (C-T) mutations were most prevalent in Chinese people (79.1%) (8).
The diagnosis of ET in a patient with β-thalassemia and splenectomy is a clinically challenge because some clinical features are overlapped between two diseases. Although the recognized complication of splenectomy is thrombocytosis, platelet number observed in reactive thrombocytosis generally is not as many as in primary thrombocytosis. From the viewpoint of increased thrombocytosis, bone marrow biopsy findings, and detection of JAK2V617F mutation (approximately 57% occurrence rate) (9), we primarily diagnosed the possible disease as ET. The coexistence of ET and β-thalassemia in one patient is quite unusual. The potential relationship between these two diseases in the case is unknown. A study tried to detect the JAK2V617F mutation in 20 patients with beta-thalassemia by RT-PCR, but the results were negative (10).
EP300 encodes a protein called p300, one of the three histone acetyltransferase (HAT) families. This protein plays an essential role in several fundamental biological processes, including cell growth and division, cell differentiation, cell cycle, and the DNA damage response. Mutations in the EP300 prevent the gene from encoding a functional protein and contribute to the development of some cancers. Work by Gayther et al. provided the first evidence that EP300 behaves as a classical tumour-suppressor gene, and showed that EP300 was mutated in breast and colorectal cancers (11). More studies demonstrated that a series of solid tumors, including gastric, colorectal, hepatocellular and ovarian carcinomas detected the loss of heterozygosity (LOH) at the p300 or CBP (12-15). In our study, positive EP300 mutation was detected in a β-thalassemia patient, which may contribute to the happening of MPNs. However, previous research showed that neither CREEBP nor EP300 mutations were found in 56 MPNs patients after systematic screening (16).
CUX1 protein encoded by CUT-like home box 1 (CUX1) gene is a highly conserved hemeprotein, which acts as a transcriptional repressor, and plays an essential role in cell cycle progression, gene expression, and differentiation. It also affects tumorigenesis (17). A study showed that CUX1 expression was associated with the incidence of myelodysplastic syndrome (MDS). When the CUX1 gene was knockdown in mice, it led to the exhaustion of hematopoietic stem cells, causing MDS (18). Only a few reports referred the CUX1 in MPNs; Thoennissen NH detected 15 post-MPN acute myeloid leukemia (AML) cases with aberrant chromosome 7 (-7 or 7q-), only one case with primary myelofibrosis (MF) had CUX1 mutation (19). Another study investigated 408 MPNs samples for single gene mutation, (including CUX1) and chromosomal aberration. They found that the deletion of chromosome 7q was closely related to post-MPNs AML, but only one patient with aberration of chromosome 7q harbored a single gene deletion of CUX1 (20).
Fibroblast growth factor receptor 3 (FGFR3) is one of the fibroblast growth factor receptors family. It plays a role in cell growth, proliferation, and angiogenesis. Nevertheless, its role in the development of MPN is still unknown.
There is no literature reporting β-thalassemia with CUX1, EP300, and FGFR3 mutation and the potential roles they played in β-thalassemia.
In summary, this is the first report of the coexistence of β-thalassemia and ET. A timely diagnosis of an ET patient with β-thalassemia is important so that ET may be managed appropriately. Furthermore, we found three novel genes mutations, EP300, CUX1, and FGFR3, after next-generation sequencing. The acquired mutations of CUX1and EP300 play roles in disease progression and transformation, especially the development of some cancers, which indicates that ET may have relationships with CUX1 and EP300 mutations. However, few studies have been reported. This single case, whether or not the mutations of CUX1, EP300 and FGFR3 in β-thalassemia are associated with the development of ET is still unconfirmed. More clinical data are required to identify the role of CUX1, EP300 and FGFR3 in the thrombocytosis, and the interaction mechanism between β-thalassemia and ET.
Acknowledgments
Funding: The research was supported by fundings of
Footnote
Conflicts of Interest: All authors have completed the ICMJE uniform disclosure form (available at http://dx.doi.org/10.21037/tcr.2020.01.48). The authors have no conflicts of interest to declare.
Ethical Statement: The authors are accountable for all aspects of the work in ensuring that questions related to the accuracy or integrity of any part of the work are appropriately investigated and resolved. All procedures performed in studies involving human participants were in accordance with the Declaration of Helsinki (as revised in 2013). Written informed consent was obtained from the patient for publication of this Case report and any accompanying images. A copy of the written consent is available for review by the Editor-in-Chief of this journal.
Open Access Statement: This is an Open Access article distributed in accordance with the Creative Commons Attribution-NonCommercial-NoDerivs 4.0 International License (CC BY-NC-ND 4.0), which permits the non-commercial replication and distribution of the article with the strict proviso that no changes or edits are made and the original work is properly cited (including links to both the formal publication through the relevant DOI and the license). See: https://creativecommons.org/licenses/by-nc-nd/4.0/.
References
- Higgs DR, Engel JD, Stamatoyannopoulos G. Thalassaemia. Lancet 2012;379:373-83. [Crossref] [PubMed]
- Tefferi A, Vardiman JW. Classification and diagnosis of myeloproliferative neoplasms: the 2008 World Health Organization criteria and point-of-care diagnostic algorithms. Leukemia 2008;22:14-22. [Crossref] [PubMed]
- Dincer AP, Subramanian S, Goksel M. Polycythemia vera with uncommon presentations. Clin Adv Hematol Oncol 2003;1:665-6; discussion 667. [PubMed]
- Lopes da Silva R, Silva M. Coexistence of β-thalassemia and polycythemia vera. Blood Cells Mol Dis 2011;46:171-2. [Crossref] [PubMed]
- Martinez-Lopez J. Myeloproliferative neoplasm in a thalassaemic patient: response to treatment with a JAK inhibitor. Ann Hematol 2015;94:1237-39. [Crossref] [PubMed]
- Galanello R, Origa R. Beta-thalassemia. Orphanet J Rare Dis 2010;5:11. [Crossref] [PubMed]
- Pan HF. Current status of thalassemia in minority populations in Guangxi, China. Clin Genet 2007;71:419-26. [Crossref] [PubMed]
- Hassan S. Detection of β-globin Gene Mutations Among β-thalassaemia Carriers and Patients in Malaysia: Application of Multiplex Amplification Refractory Mutation System-Polymerase Chain Reaction. Malays J Med Sci 2013;20:13-20. [PubMed]
- Baxter EJ, Scott LM, Campbell PJ, et al. Acquired mutation of the tyrosine kinase JAK2 in human myeloproliferative disorders. Lancet 2005;365:1054-61. [Crossref] [PubMed]
- Vlachaki E. Absence of JAK2V617F mutation in patients with beta-thalassemia major and thrombocytosis due to splenectomy. Mol Biol Rep 2012;39:6101-5. [Crossref] [PubMed]
- Gayther SA, Batley SJ, Linger L, et al. Mutations truncating the EP300 acetylase in human cancers. Nat Genet 2000;24:300-3. [Crossref] [PubMed]
- Bryan EJ, Jokubaitis VJ, Chamberlain NL. Mutation analysis of EP300 in colon, breast and ovarian carcinomas. Int J Cancer 2002;102:137-41. [Crossref] [PubMed]
- Tillinghast GW, Partee J, Albert P. Analysis of genetic stability at the EP300 and CREBBP loci in a panel of cancer cell lines. Genes Chromosomes Cancer 2003;37:121-31. [Crossref] [PubMed]
- Koshiishi N, Chong JM, Fukasawa T. P300 gene alterations in intestinal and diffuse types of gastric carcinoma. Gastric Cancer 2004;7:85-90. [Crossref] [PubMed]
- Dancy BM, Cole PA. Protein lysine acetylation by p300/CBP. Chem Rev 2015;115:2419-52. [Crossref] [PubMed]
- Andersen CL, Hasselbalch H, Gronbaek K. Lack of somatic mutations in the catalytic domains of CREBBP and EP300 genes implies a role for histone deacetylase inhibition in myeloproliferative neoplasms. Leuk Res 2012;36:485-7. [Crossref] [PubMed]
- Ramdzan ZM, Nepveu A. CUX1, a haploinsufficient tumour suppressor gene overexpressed in advanced cancers. Nat Rev Cancer 2014;14:673-82. [Crossref] [PubMed]
- An N, Khan S. Gene dosage effect of CUX1 in a murine model disrupts HSC homeostasis and controls the severity and mortality of MDS. Blood 2018;131:2682-97. [Crossref] [PubMed]
- Thoennissen NH. Novel CUX1 missense mutation in association with 7q- at leukemic transformation of MPN. Am J Hematol 2011;86:703-5. [Crossref] [PubMed]
- Klampfl T. Genome integrity of myeloproliferative neoplasms in chronic phase and during disease progression. Blood 2011;118:167-76. [Crossref] [PubMed]