This article has an erratum available at: http://dx.doi.org/10.21037/tcr-2023-04 the article has been update on 2023-10-23 at here.
Atorvastatin inhibits pancreatic cancer cells proliferation and invasion likely by suppressing neurotrophin receptor signaling
Introduction
Pancreatic cancer (PC) is a devastating disease with an extremely poor median survival of less than 6 months due to its aggressive nature. Each year, approximately 40,000 individuals are diagnosed with this lethal malignancy worldwide. It is now the 7th leading cause of cancer-related death globally (1-3). At the time of diagnosis, only approximately 15% of patients are candidates for potentially curative surgery, the remainders are already in advanced stages with unresectable lesions (2,4). For patients with unresectable disease, chemotherapy and radiotherapy remain the standard treatment options. However, PC cells are typically resistant to most of these conventional therapeutic modalities (5-7). Therefore, novel therapeutic strategies are in urgent need to reverse this dismal condition and improve prognosis associated with PC.
Recent evidence has suggested an essential role of cholesterol in the tumorigenesis and development of several human cancers including PC. In one meta-analysis, the authors analyzed 20 published articles encompassing 2,245,052 participants and concluded that dietary cholesterol may be associated with an increased risk for PC (8). Meanwhile, another study found that cholesterol uptake is aberrantly activated in PC cells and blocking cholesterol uptake not only inhibited proliferation but also increased the chemo-sensitivity of human PC cells (9). Furthermore, the prevention of cholesterol biosynthesis has been reported to sensitize PC cells to radiotherapy (10). Taken together, these data suggest that cholesterol is a promising target for clinical PC treatment.
Atorvastatin is a cholesterol-lowering agent that works by competitively inhibiting 3-hydroxy-3-methylglutarylcoenzyme-A (HMG CoA) reductase; it has been in clinical use for many years with an established safety profile (11). Several recent studies have found that statins exert antitumor activities in various common malignancies including breast, liver and colon cancer (12,13). Therefore, the aim of this present study is to investigate the effect and potential underlying mechanism of atorvastatin on the proliferation, apoptosis, cell cycle distribution, migration, invasion of human PC cell lines, providing rationale for atorvastatin as a novel targeted molecular agent for clinical PC therapy.
Methods
Cell culture and reagents
The human PC cell lines PANC-1 and SW1990 were purchased from Shanghai Cell Bank (Shanghai, China). Both cell types were cultured in Dulbecco’s Modified Eagle Media (DMEM) medium supplemented with 10% fetal bovine serum, 1% penicillin and streptomycin, and grown in a humidified incubator at 37 °C with 5% CO2. All tissue culture reagents and atorvastatin were purchased from Sigma-Aldrich (St. Louis, MO, USA).
Cell viability assay
Cell viability was determined by the CellTiter-Glo luminescent cell viability assay. Briefly, PANC-1 and SW1990 cells (2×103) were seeded onto 96-well culture plates. After incubation overnight, the medium was replaced with fresh medium containing dimethyl sulfoxide (DMSO) (vehicle control) or different concentrations of atorvastatin. After incubation for the indicated time, 20 µL of CellTiter 96® AQueous One Solution Reagent (Promega, Madison, WI, USA) was added to each well. After incubation for another 2 h, the optical density (OD) was measured using an ELx800 plate reader (Bio-Tek; Winooski, VT, USA) at a wavelength of 490 nm. All samples were run in triplicate.
Colony formation assay
PANC-1 and SW1990 cells were trypsinized and plated (800 cells/well) onto 12-well tissue culture plates; the medium was replaced with fresh medium containing different concentrations of atorvastatin 24 h later. After 6 days of incubation, cells were rinsed with 1× PBS, fixed with methanol and stained with 0.1% crystal violet. The numbers of colonies were counted manually.
Apoptosis and cell cycle distribution analysis by flow cytometry
The apoptosis and cell cycle distribution of PANC-1 and SW1990 cells were determined by the Annexin V-FITC detection kit (Becton, Dickinson and Company, USA). Cells were inoculated onto 6-well tissue culture plates (3×104 cells/well) and treated with different concentrations of atorvastatin for the indicated times. The cells were then trypsinized, washed twice with ice-cold PBS and resuspended in 1× binding buffer at a concentration of 105/mL in a total volume of 100 µL. Subsequently, 5 µL of both Annexin V-FITC and PI were added, and the samples were oscillated gently to evaluate the apoptosis rate and cell cycle distribution via flow cytometry. All samples were run in triplicate.
Wound healing assay
For the wound healing assay, 90% confluent monolayers of PANC-1 and SW1990 cells with or without atorvastatin treatment were “wounded” using a pipette tip. The wounds were photographed (0, 24 and 48 h) in the same area, and the wound healing rate was measured.
Migration and invasion assay
Briefly, PANC-1 or SW1990 (5×104) cells were seeded onto the upper chamber in fatal bovine serum (FBS)-free medium with different concentrations of atorvastatin, and medium with 10% FBS was added into each lower chamber of 24-well tissue culture plates; cells were allowed to migrate and invade through the membrane pores with or without Matrigel for 20 h. The cells on the surface of the lower side of the chamber were then fixed, stained (Calcein AM), and photographed. Five visual fields were photographed in every membrane, and the stained cells were manually counted. All samples were run in triplicate. For the invasion assay, crystal violet was dissolved in 70% acetic acid, and the absorbance of each well was measured by a microplate reader at a wavelength of 450 nm.
Western blotting
Cells were harvested and lysed in Radio-Immunoprecipitation Assay (RIPA) lysis buffer (Beyotime Biotechnology, China) containing protease inhibitors for 20 min at 4 °C. The proteins were separated by 10% dodecyl sulfate, sodium salt-Polyacrylamide gel electrophoresis (SDS-PAGE) and transferred to polyvinylidene fluoride (PVDF) membranes (Millipore, USA). After blocking with 5% milk, the samples were incubated with the primary antibodies. The membranes were then incubated with a horseradish peroxidase (HRP)-conjugated anti-rabbit or anti-mouse secondary antibody (Jackson ImmunoResearch, USA). The protein bands were visualized using enhanced chemiluminescence (ECL, Beyotime Biotechnology, China). Endogenous β-actin protein expression was detected as the internal control for each sample.
The primary antibodies included β-actin (Beyotime Biotechnology, China), caspase-3 (Abcam, USA), p21 (Abcam, USA), p-chk2 (Abcam, USA), NGF/TrkA, BDN F/TrkB, NT3/TrkC and p75 (Abcam, USA).
Statistics
Results are expressed as the means ± SEM and were analyzed using SPSS 22.0. Statistical comparisons between groups were performed using two-way ANOVA. P<0.05 was considered statistically significant.
Results
Atorvastatin inhibits the proliferation of PC cells
To assess the impact of atorvastatin on the proliferation of human PC, PANC-1 and SW1990 cells were treated with different concentrations of atorvastatin for the indicated times. Then, the CellTiter-Glo luminescent cell viability assay was conducted. As shown in Figure 1A and B, atorvastatin treatment inhibited the proliferation of PC cells in a dose-dependent manner. The colony formation assay was also conducted. As shown in Figure 1C and D, the atorvastatin treatment exerted anti-proliferation effect in PC cells. Particularly, the numbers of viable PANC-1 and SW1990 colonies were significantly decreased after atorvastatin (1 nM or higher) treatment, as shown in Figure 1E and F. These above results indicated that atorvastatin can inhibit the proliferation ability of PC cells in vitro.
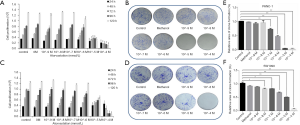
Atorvastatin induces apoptosis in PC cells (Figures 2,3)
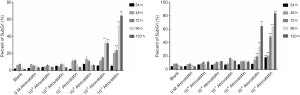
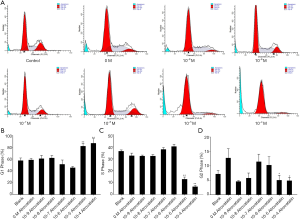
Next, we studied whether atorvastatin treatment can induce apoptosis in PC cells by flow cytometry. The results showed that 10 nM or higher dose of atorvastatin treatments efficiently induced apoptosis of both PANC-1 (Figures 2A,3A) and SW1990 cells (Figures 2B,3B).
Atorvastatin induces the G1-cell cycle arrest of PC cells
We further investigated whether atorvastatin-mediated anti-proliferation effect in PC cells was related to cell cycle arrest. As shown in Figures 3A and 4A, 10 nM or higher dose of atorvastatin treatment significantly increased the percent of G1 phase in both PANC-1 and SW1990 cells. Correspondingly, the percentages of S and G2 phase cells were significantly decreased (Figures 3B,C,4B,C). Meanwhile, western blot analysis showed the increase of p21 expression (Figure 5A,B) and decrease of phosphorylated checkpoint kinase 2 Thr68 (Chk2-T68) expression (Figure 5C,D) after atorvastatin treatment in both PANC-1 and SW1990 cells. The above results demonstrate that atorvastatin induces G1 arrest in PC cells.
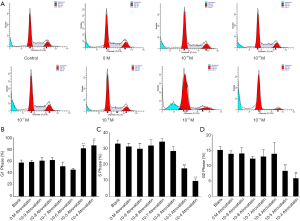
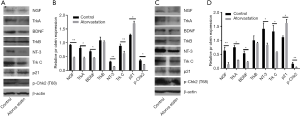
Atorvastatin inhibits the migration and invasion of PC cells
We further explored the impact of atorvastatin on the migration and invasion of PC cells. Wound healing assay results showed that 10 nM or higher dose of atorvastatin treatment significantly decreased the rate of wound closure in both cells compared to control group (Figure 6). Meanwhile, transwell invasion assay results demonstrated that atorvastatin treatment markedly reduced the invasion ability of both cells (Figure 7). These results above indicated that atorvastatin can inhibit the migration and invasion ability of PC cells.
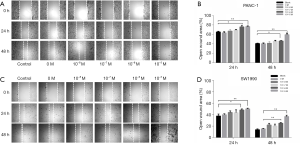
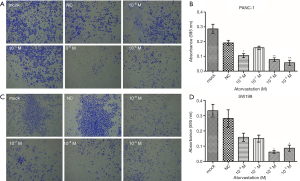
Atorvastatin decreased the expression of neurotrophins and their receptors
Finally, the potential mechanism underlying the antitumor effect of atorvastatin on PC cells was assessed. Since the neurotrophin signaling pathway has been extensively implicated in the malignant behavior in various types of human malignancies including PC, several neurotrophins and their downstream receptors in PC cells after atorvastatin treatment were tested by western blotting. As shown in Figure 7, 10 nM of atorvastatin treatment significantly decreased the expression of NGF, BDNF, and NT-3 as well as that of their downstream receptors Trk A and Trk C but not that of Trk B. These results above indicated that atorvastatin may exert the anti-tumor effect in PC via the inhibition of neurotrophin signaling pathway.
Discussion
The results of the current study showed that atorvastatin, a cholesterol-lowering agent of the statin family, could be a potential anti-PC agent. Our data demonstrated that atorvastatin inhibited the proliferation of PC cells, as evidenced by the decreased viability and colony formation ability, induction of apoptosis and G1 phase cell cycle arrest. Furthermore, our results showed that atorvastatin activated p21 expression and reduced phosphorylation of Chk2-T68. We also found that atorvastatin treatment inhibited the migration and invasive capacity of PC cells. We further showed that atorvastatin treatment blocked neurotrophin signaling in two PC cells.
PC is one of the most aggressive human malignancies, with an extremely rapid proliferation ability and a high distant metastasis rate; it is resistant to conventional therapies, including chemotherapy and radiotherapy, and has a dismal survival rate of 6 months. To improve the outlook of this disease, additional novel therapeutic strategies are urgently needed. Some studies have assessed the antitumor effects of the statin family of drugs in several human malignancies, including breast, colon and ovarian cancers. However, the effect of statins on PC has not yet been fully studied. Guillaumond et al. (9) demonstrated that low-density lipoprotein receptor (LDLR), which facilitates cholesterol uptake, was associated with an increased risk of pancreatic ductal adenocarcinoma (PDAC) recurrence. Blocking LDLR reduced the proliferation potential of PDAC cells. LDLR silencing sensitizes PDAC cells to chemotherapeutic drugs. In human PDAC, enhanced LDLR expression is associated with poor patient prognosis (9). Therefore, the use of an agent in the statin family to disrupt cholesterol uptake independently or in combination with chemotherapy may be a promising therapeutic strategy for these patients. In this study, the antitumor properties of atorvastatin were assessed by the cell viability assay, colony formation assay, flow cytometry, wound healing assay and Transwell invasion assay. The cell viability assay showed that at concentrations higher than 10 nM, atorvastatin treatment significantly inhibited the viability of PC cells in a dose-dependent manner. In the colony formation assay, atorvastatin treatment effectively inhibited the growth of PC cells. Flow cytometry data indicated that high-dose atorvastatin treatment (10 nM or higher) induced apoptosis and G1-phase arrest in PC cells; both apoptosis and cell cycle arrest are currently topics of keen interest in cancer research. Furthermore, the wound healing assay and Transwell invasion assay showed that atorvastatin treatment (10 nM or higher) significantly inhibited the migration and invasion ability of PC cells. Taken together, we have shown that atorvastatin does exert anti-tumor effect in PC.
Neurotrophin signaling contains the upstream neurotrophins and their downstream receptors. The former is a family of structurally conserved growth factors, including NGF, BDNF, NT-3 and NT-4/5, while the latter includes TrkA, TrkB and TrkC. Increasing evidence suggests that dysregulated neurotrophin signaling plays a pivotal role in the development of cancer, including PC (14,15). A recently published Cancer Cell study found that NGF is overexpressed and promotes accelerated tumor development in PDAC cells, and therapy with a Trk inhibitor in conjunction with gemcitabine increased survival in a PC mouse model (16). Meanwhile, several clinical studies have indicated that NGF, TrkA, TrkB, TrkC and other neurotrophin signaling members are aberrantly overexpressed in PC samples compared to that in normal adjacent tissues (17-19). Thus, neurotrophin signaling is one of the most attractive therapeutic targets in PC. To our knowledge, our results are the first to demonstrate that atorvastatin exerts anti-tumor effects and inhibits neurotrophin signaling in PC, as evidenced by the decreased NGF, NT-3, Trk A and Trk C levels after atorvastatin treatment. However, assessing the dependency of the atorvastatin-mediated antitumor effect on the inhibition of neurotrophin signaling is necessary.
Conclusions
The association between statin use and the prognosis of cancer patients is complicated. However, this study demonstrated that atorvastatin alone inhibited the proliferation, migration and invasion of PC cells by suppressing neurotrophin signaling. Thus, atorvastatin, an inexpensive, widely used lipid-lowering agent with a well-established safety profile, may be a potential anti PC agent. The antitumor effects of atorvastatin and the precise mechanisms underlying these effects will need to be further investigated in vivo in the future.
Acknowledgments
Funding: This study was supported by
Footnote
Conflicts of Interest: All authors have completed the ICMJE uniform disclosure form (available at http://dx.doi.org/10.21037/tcr.2020.01.27). The authors have no conflicts of interest to declare.
Ethical Statement: The authors are accountable for all aspects of the work in ensuring that questions related to the accuracy or integrity of any part of the work are appropriately investigated and resolved. This research was approved by the medical ethics committee of Nanjing Medical University. The study was conducted in accordance with the Declaration of Helsinki (as revised in 2013). Informed consent was waived.
Open Access Statement: This is an Open Access article distributed in accordance with the Creative Commons Attribution-NonCommercial-NoDerivs 4.0 International License (CC BY-NC-ND 4.0), which permits the non-commercial replication and distribution of the article with the strict proviso that no changes or edits are made and the original work is properly cited (including links to both the formal publication through the relevant DOI and the license). See: https://creativecommons.org/licenses/by-nc-nd/4.0/.
References
- Siegel RL, Miller KD, Jemal A. Cancer statistics, 2019. CA-Cancer J Clin 2019;69:7-34. [Crossref] [PubMed]
- Miller KD, Nogueira L, Mariotto AB, et al. Cancer treatment and survivorship statistics, 2019. CA-Cancer J Clin 2019;69:363-85. [Crossref] [PubMed]
- Feng RM, Zong YN, Cao SM, et al. Current cancer situation in China: good or bad news from the 2018 Global Cancer Statistics? Cancer Commun (Lond) 2019;39:22. [Crossref] [PubMed]
- Allemani C, Weir HK, Carreira H, et al. Global surveillance of cancer survival 1995–2009: analysis of individual data for 25 676 887 patients from 279 population-based registries in 67 countries (CONCORD-2). Lancet 2015;385:977-1010. [Crossref] [PubMed]
- Hazard L. The role of radiation therapy in pancreas cancer. Gastrointest Cancer Res 2009;3:20-8. [PubMed]
- Cintas C, Douche T, Therville N, et al. Signal-Targeted Therapies and Resistance Mechanisms in Pancreatic Cancer: Future Developments Reside in Proteomics. Cancers 2018;10. [PubMed]
- Grasso C, Jansen G, Giovannetti E. Drug resistance in pancreatic cancer: Impact of altered energy metabolism. Crit Rev Oncol Hematol 2017;114:139-52. [Crossref] [PubMed]
- Wang J, Wang WJ, Zhai L, et al. Association of cholesterol with risk of pancreatic cancer: a meta-analysis. World J Gastroenterol 2015;21:3711-9. [Crossref] [PubMed]
- Guillaumond F, Bidaut G, Ouaissi M, et al. Cholesterol uptake disruption, in association with chemotherapy, is a promising combined metabolic therapy for pancreatic adenocarcinoma. P Natl Acad Sci USA 2015;112:2473-8. [Crossref] [PubMed]
- Souchek JJ, Baine MJ, Lin C, et al. Unbiased analysis of pancreatic cancer radiation resistance reveals cholesterol biosynthesis as a novel target for radiosensitisation. Brit J Cancer 2014;111:1139-49. [Crossref] [PubMed]
- Zuo Y, Li T, Lei Z. Should we add atorvastatin to irbesartan for improving renoprotective effects in early diabetic nephropathy? A meta-analysis of randomized controlled trials. Pharmacol Res 2019;146:104286. [Crossref] [PubMed]
- Ahern TP, Lash TL, Damkier P, et al. Statins and breast cancer prognosis: evidence and opportunities. Lancet Oncol 2014;15:e461-e468. [Crossref] [PubMed]
- Ling Y, Yang L, Huang H, et al. Prognostic Significance of Statin Use in Colorectal Cancer: A Systematic Review and Meta-Analysis. Medicine (Baltimore) 2015;94:e908. [Crossref] [PubMed]
- Johnson MD, Stone B, Thibodeau BJ, et al. The significance of Trk receptors in pancreatic cancer. Tumour Biol 2017;39:1010428317692256. [Crossref] [PubMed]
- Khotskaya YB, Holla VR, Farago AF, et al. Targeting TRK family proteins in cancer. Pharmacol. Ther 2017;173:58-66. [Crossref] [PubMed]
- Renz BW, Takahashi R, Tanaka T, et al. β2 Adrenergic-Neurotrophin Feedforward Loop Promotes Pancreatic Cancer. Cancer Cell 2018;34:863-7. [Crossref] [PubMed]
- Rubin JB, Segal RA. Growth, survival and migration: the Trk to cancer. Cancer Treat Res 2003;115:1-18. [PubMed]
- Ma J, Jiang Y, Jiang Y, et al. Expression of nerve growth factor and tyrosine kinase receptor A and correlation with perineural invasion in pancreatic cancer. J Gastroenterol Hepatol 2008;23:1852-9. [Crossref] [PubMed]
- Kue CS, Kamkaew A, Voon SH, et al. Tropomyosin Receptor Kinase C Targeted Delivery of a Peptidomimetic Ligand-Photosensitizer Conjugate Induces Antitumor Immune Responses Following Photodynamic Therapy. Sci Rep 2016;6:37209. [Crossref] [PubMed]