This article has an erratum available at: http://dx.doi.org/10.21037/tcr-2024-5 the article has been update on 2024-08-20 at here.
MicroRNA-106a suppresses prostate cancer proliferation, migration and invasion by targeting tumor-derived IL-8
Introduction
Prostate cancer (PCa) is the most common solid tumor in men and the second leading cause of male mortality in North America (1). It is increasingly obvious that chemokines within the tumor microenvironment are involved in the acquired capability for invasive growth and metastasis (2-4). Multiple chemokines such as C-C motif chemokine 2, interleukin-12, interleukin-8 (IL-8) are principle factors implicated in the progression of PCa (3,5,6). IL-8 derived either from tumor cells themselves or other cellular components is crucial for PCa progression (6-8).
IL-8, also known as C-X-C motif chemokine ligand 8 (CXCL8), exerts many biological effects through its C-X-C motif chemokine receptors 1/2 (CXCR1 and CXCR2). IL-8 and its receptors were overexpressed in PCa biopsy specimens, however, they decreased in prostatic intraepithelial neoplastic (PIN) foci and even absent in normal prostate tissues (9). Tumor-derived IL-8 exhibits a profound effect on PCa cells. Autocrine IL-8 by tumor themselves promotes disease progression and castration resistance, enhances metastatic potential and increases survival in response to chemotherapy (7,10,11). Furthermore, recent findings indicated that increasing tumor-derived IL-8 caused by partial or complete loss of phosphatase and tensin homolog (PTEN) was essential to cell viability in the early stages of PCa progression (12).
MicroRNAs (miRNAs) are critical regulators of post-transcriptional target genes via repressing translation and/or by promoting mRNA degradation (13-16), and these functions have been identified in a wide variety of cancers (17-20). Accumulating studies have demonstrated that miRNAs act as importance post-transcriptional regulators in the progression of oncogenesis and metastasis, suggesting their potential roles in anti-cancer therapeutics (21-24). Till now, a wide variety of miRNA expression profiles have been conducted in PCa (25-27). Liu et al. reported miR-106a was lower expressed in both PCa stem cell lines and xenograft models (28). Moreover, an important miRNA profile recently identified miR-106a as a useful biomarker for predicting PCa aggressive progression (29). Our group previously performed microarrays to find out the different expression profiles of both miRNA and mRNA in PCa cell lines and finally found that miR-106a was downregulated, while IL-8 was upregulated in androgen-independent PCa cells lines. However, the function and mechanism of miR-106a in PCa progression have not been investigated.
Using bioinformatics analysis, we detected that IL-8 might be a target gene of miR-106a. Therefore, in the current study, we intended to investigate whether miR-106a could affect the progression of PCa via targeting IL-8 or not. Here, we identified that proliferation, apoptosis, invasion, and migration of PCa cells mediated by miR-106a through directly targeting IL-8. These data provide new insight into the mechanism of PCa progression, suggesting that targeting IL-8 by miR-106a might be useful for PCa therapy.
Methods
Cell lines
Human PCa cell lines PC-3, DU145, LNCaP, normal human prostate epithelial cell line RWPE-1 and human embryonic kidney cells 293 (HEK-293) were obtained from American Type Culture Collection (ATCC). Cells were passaged and maintained in RPMI-1640 medium (PC-3, DU145, LNCaP, RWPE-1) or in DMEM (HEK-293), with 10% fetal calf serum, penicillin (100 mg/mL) and streptomycin (100 mg/mL) (Gibco/Life Technologies, NY, USA).
RNA extraction, conventional reverse transcription-PCR, and quantitative real-time PCR
Total RNA, including miRNA, was isolated from cells using the TRIzol® reagent (Invitrogen, Carlsbad, CA) in accordance with the manufacturer’s protocols. PCR was carried out as described previously (30). The stem-loop reverse transcription-PCR (RT-PCR) primer was designed to examine miR-106a (5'-GTCGTATCCAGTGCAGGGTCCGAGGTATTCGCACTGGATACGACCTACCT-3'). The Mature level miR-106a were examined by stem-loop RT-PCR. The primer sequences were designed as follows: primers for miR-106a, forward 5'-CGGCGGTCCAGTTTTCCCAGG-3', reverse 5'-CATAGCCCGCAAAGTCT T-3'; primers for U6 gene, forward 5'-TGGAACGATACAGAGAAGATTAGCA-3', reverse 5'-AACGCTTCACGAATTTGCGT-3'. U6 was used as an endogenous control for data normalization. Quantitative real-time polymerase chain reaction (Q-PCR) was performed by using SYBR Premix ExTaq (Takara, Otsu, Japan) on the ABI 7500 real-time PCR System, with U6 used as an internal control.
Western blotting analysis and Immunocytochemistry
Western blot was performed as described previously with primary antibodies: IL-8 (1:3,000, Santa Cruz, CA, USA) and GAPDH (1:10,000, Kangcheng, China). Signals were visualized by ECL reagents (Millipore, MA, USA) (30). On immunocytochemistry, cells were fixed in 4% paraformaldehyde, permeabilized with 0.1% Triton X-100, and then incubated overnight at 4 °C with anti-IL-8 antibody 1:100 (Abcam, MA, USA).
Recombinant adenoviral vectors for overexpression of miR-106a
Pri-miR-106a sequence was cloned by PCR from HEK-293 cell genomic DNA flanked with Kpn1 and Sal1 restriction site sequences (primers: forward, 5'-GGTACCTGTAAGCCAAAGGAAAGC-3'; reverse, 5'-GTCG ACGCACCTTAACACAAGAGACAC-3'). PCR product was inserted into pMD18/T (TaKaRa) and then cloned into pAd-Track-CMV (Invitrogen) after being sequenced. pAd-Track-miR-106a was generated by homologous recombination of adenovirus vector pAdeasy-1 in E.coli BJ5183 cells (Stratagene, Wilmington, NC). The resulting pAdeasy-miR-106a was transfected into HEK-293 cells to obtain viral particles. To amplified Ad-miR-106a, several cycles of infection were repeated and the viral genome copy number was verified by PCR. The control (Ad-control) was an empty vector.
3'-UTR reporter construct cloning and luciferase reporter assay
The 3'-UTR of IL-8 with the predicted binding site of miR-106a was constructed by the pGL3-Promoter vector (Promega). Mutant IL-8 3'-UTR with mutation/deletion of seed sequences were created by overlapping PCR (primers: IL8-Mut, forward 5'-GGAAAGATCTCAGTTTTTTCATC-3', L8-Mut, reverse 5'-GAAAAAACTGAGATCTTTCCAC-3'; IL8-Del, forward 5'-CATGTGGAAAGTTTTTTCATCA TAAC-3', IL8-Del, reverse 5'-GAAAAAACTTTCCACATGTCCTC-3'. Reporter construct and the pRL-CMV plasmid were co-transfected by using Lipo 2000 (Invitrogen). The firefly and luciferase activities were measured 24 h post-transfection using Luminometer TD-20/20 (Turner Design, CA, USA).
Cell proliferation analysis and TUNEL assay
PC-3 and DU145 cell proliferation were measured by 3-(4,5)-dimethylthiahiazo (-z-y1)-3,5-di- phenytetrazoliumromide (MTT) cell assay (Sigma). Cells were plated in 96-well plates with an initial density of 5×103 per well, at 24, 48, 72, and 96 h, each well was added 20 µL of 5 mg/mL MTT, incubated 4-hour at 37 °C, removed the MTT solution, added 150 µL dimethylsulfoxide (DMSO) to each well, absorbance measured at 590 nm using 96-well plate reader (SPECTRAmax PLUS, CA, USA). TUNEL assay (Roche, Mannheim, Germany) was performed to detect the Apoptotic index of cells according to the manufacturer’s recommendations.
Migration and invasion assays
To assess cell migration and invasion abilities after transfected AD-miR-106a, we used Trans-well-polycarbonate filter membrane (8-µm pore size, Millipore, MA, USA) with Matrigel (BD Biosciences, CA, USA). Cells were incubated into the top chamber (5×104/cm2) with FBS-free medium and the bottom chamber contained RPMI 1640 with 10% fetal bovine serum (FBS) (migration assay: 8, 16, and 24 h; invasion assay: 24 and 48 h). Cells passing through the film were fixed, stained and counted under a microscope.
Statistical analysis
Statistical analyses were performed with SPSS version 20.0 (SPSS Inc., Chicago, IL, USA). All data were shown as means ± standard deviation (SD). A comparison between groups and controls was determined by One-Way ANOVA and independent sample t-test. P<0.05 was defined as statistical significance.
Statement of ethics approval
This study was basic research and did not involve any patient sample and animal. It was not required ethics approval.
Results
The expression of miR-106a and IL-8 in PCa cell lines and normal epithelial cell line
Since autocrine IL-8 is strongly associated with PCa progression, we initially evaluated the expression of IL-8 in normal and cancer cell lines. Both IL-8 mRNA and its protein expression were observed higher in androgen-independent PCa cell lines (PC-3 and DU145) compared with androgen-dependent PCa (LNCaP) and normal epithelial cell line (RWPE-1) (Figure 1A,B,C,D). Subsequently, miR-106a was determined by conventional RT-PCR and Q-PCR. In contrast to IL-8, miR-106a was observed down-regulated in PCa cell lines, whereas upregulated in normal prostate epithelial cells (Figure 1E,F). These findings indicated the negative expression of miR-106a and IL-8 in PC-3 and DU145.
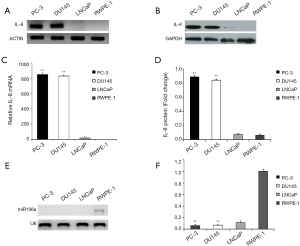
The interaction between miR-106a and IL-8 in PC-3 and DU145 cells
To investigate the interaction between miR-106a and IL-8 in PCa cell lines, miR-106a vectors were constructed. Androgen-independent PCa cells transfected with miR-106a obviously inhibited the production of IL-8 compared to Ad-control (Figure 2A,B,C,D,E,F), indicating that the expression levels of IL-8 may be inhibited by the overexpression of miR-106a.
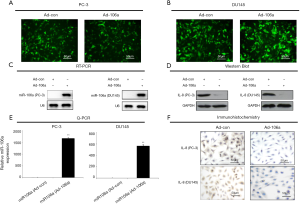
The interaction of MiR-106a with the 3'-UTR of IL-8 mRNA
To examine the binding sites of miR-106a in the 3'-UTR of IL-8 mRNA, we carried out dual reporter gene assays. First, we constructed luciferase reporter genes containing wild and mutant sequences of IL-8 3'-UTR and then cloned them into the luciferase reporter vector pGL3-IL8 (Figure 3A). As shown in Figure 3B, the luciferase levels of the reporter with wild-type IL-8 3'-UTR was significantly inhibited in the cells transfected with miR-106a (about 78%), but not these with mutant IL-8 3'-UTR. These data suggested that miR-106a could reduce IL-8 expression by directly binding to the 3'-UTR of IL-8.
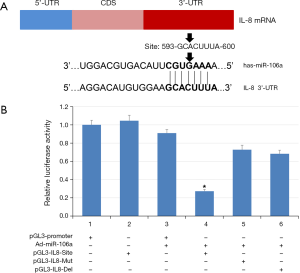
Overexpression of miR-106a suppresses the growth and promotes the apoptosis of PCa cells
To further investigate the biological function of miR-106a on PCa cells, MTT and TUNEL assays were performed. MiR-106a overexpression could inhibit the proliferation of PCa cells at 96h after Ad-106a infection compared with blank and Ad-con (Figure 4A). Moreover, the cells apoptosis was significantly increased after transfecting Ad-miR-106a (Figure 4B).
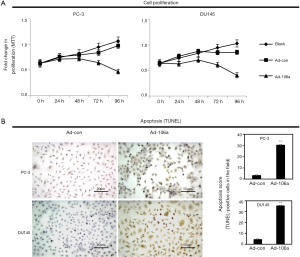
Overexpression of miR-106a suppresses the invasion and migration of PCa cells
As metastasis is the major property of hormone-independent PCa, we subsequently analyzed the effects of miR-106a on invasion and migration behavior (PC-3 and DU145). Notably, miR-106a overexpression abrogated cell migration at 8, 12 and 24 h post-transfection (Figure 5A,B). Simultaneously, the cell invasion ability was also obviously suppressed at 24 and 48 h following transfected with miR-106a (Figure 5C,D).
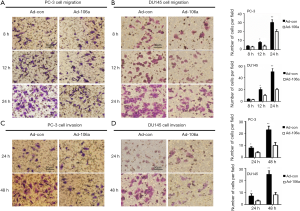
Discussion
Tumor-derived IL-8 promotes growth, invasion, metastasis, angiogenesis and enhances resistance to chemotherapies through autocrine signaling pathways in PCa. Here, we explored the potential for miR-106a to serve as a principle post-transcriptional regulator in PCa progression demonstrating that miR-106a fosters apoptosis and inhibits proliferation, invasion, and migration via targeting IL-8 in PCa cell lines. Thus, the inhibition of the autocrine effects of IL-8 by upregulating miR-106a might provide a potential therapeutic approach to block the progression of PCa.
MiR-106a, a member of the miR-17 family, is broadly expressed in a huge variety of cell types. MiR-106a-363 cluster, as well as a miR-106b-25 cluster, is the paralogs of miR-17-92 cluster given rise to gene duplications. Although predominate data show that miR-17-92 cluster could promote oncogenesis in a variety of tumors including PCa, there is some evidence addressing the reduction of this miRNA cluster might facilitate tumor progression in certain tumors (31,32). Moreover, individual miRNA biogenesis is under different control in vivo. For this reason, their biological mechanism could be either similar or completely adverse. Studies underlying the individual function of miRNA, therefore, are critical to elucidate the exact biological roles of the miR-17 family in tumorigenesis.
The expression and functionality of miR-106a vary in different cancers. Data from several studies indicated that abnormality of miR-106a expression could be either oncogenic or tumor-suppressive (33,34). Aberrant presence of miR-106a was positively correlated with poor prognosis, enhanced capability of invasive growth and metastasis in gastric and ovarian cancer, conversely, dysregulated miR-106a was an independent predictor for poor survival in breast cancer, osteosarcoma, glioblastoma and astrocytoma (4,35-41). Several genes correlated with the regulation of miR-106a were proposed on tumor development, progression and drug resistance (6,36,39,41,42). Upregulated miR-106a exerted antagonistic effects by inhibiting proliferation and inducing apoptosis via targeting E2F transcription factor 1 (E2F1) in glioma and colorectal cancer and promoting stress-induced apoptosis by targeting fas activated serine/threonine kinase (FASTK) in astrocytoma (43,44). In contrast, overexpression of miR-106a paradoxically inhibited the cellular extrinsic apoptotic pathway by targeting Fas cell surface death receptor (FAS) in gastric cancer and inhibited the cell migration and invasion of renal cell carcinoma through targeting p21 activated kinase 5 (PAK5) (23,45). Moreover, by directly targeting tissue inhibitors of metalloproteinase 2 (TIMP2), miR-106a enhanced gastric cell migration and invasion (41). Furthermore, through triggering associated apoptosis genes, runt-related transcription factor 1/3 (RUNX 1/3), myeloid cell leukemia-1 (Mcl-1), B-cell lymphoma/leukemia 10 (BCL10), caspase-7, miR-106a exerted a pivotal role in drug resistance in the gastric tumor, ovarian tumor and osteosarcoma (11,35,36,46).
Surprisingly, other than the miR-17-92 cluster, very few studies have explored miR-106a in PCa. Nevertheless, the function and mechanism of miR-106a in PCa remains to be better understood. Liu and his colleagues conducted miRNA expression profiling and found that miR-106a was dysregulated in both PCa stem/progenitor cell subsets and human PCa tissues (28). Importantly, by performing Scano-miR bioassay, Alhasan et al. identified that upregulated serum miR-106a was strongly associated with the aggressive progression of PCa (29). Therefore, the differential expression of miR-106a in different samples and PCa cell lines indicated the multiplicity of miR-106a in PCa. Our study is the first to explore the potential role of miR-106a in PCa progression.
Several genes have been reported to be direct targets of miR-106a. We chose to focus on IL-8 owing to its crucial role in PCa development and progression, especially in the castration resistance stage. IL-8 as a principle proinflammatory chemokine usually secreted by both normal and cancer cells, including PCa. Caruso et al. reported the expression of IL-8 in primary PCa tissues positively related to the biochemical recurrence (18). Furthermore, the expression of IL-8 and its receptors were detected in PCa biopsy samples, but absent or downregulated in normal tissues and PIN foci respectively (9). Tumor-derived IL-8 has the capacity to exert profound effects on tumor progression. IL-8 serves as an autocrine growth factor that promotes activation of survival and metastasis pathways and induces castration resistance in PCa via activating its classical receptors. In addition, Singh et al. also demonstrated the ligand-independent pathway by the interaction between IL-8 and C-X-C motif chemokine receptor 7 (CXCR7) in PCa progression (47). Recently, an elegant study found that PTEN deficiency could sustain proliferation and survival through selective overexpression of IL-8, underlying its crucial role in the early stages of PCa development (12).
IL-8 is frequently highly expressed in PCa, but the cause of its overexpression remains unclear. miRNAs can suppress target genes expression via target mRNA degradation, translational repression or de-adenylation. We observed that when miR-106a was strengthened, both IL-8 mRNA and protein were strongly inhibited, and IL-8 is the target gene of miR-106a. In line with our results, Hong et al. found serum miR-106a could repress IL-8 expression via targeting 3'-UTR of IL-8 in chronic hepatitis B patients (48).
To further investigate the biological effects of miR-106a on PCa cells, we examined the proliferation, invasion, migration, and apoptosis in androgen-independent PCa cells. Interestingly, PCa cell proliferation was inhibited along with increased apoptosis after transfected with miR-106a. Moreover, the migration and invasion abilities were also suppressed by upregulated miR-106a. In line with our study, previous studies found that enforced expression of miR-106a could inhibit cell proliferation and induce cell apoptosis via targeting E2F1 or FASTK in nervous system neoplasms (42,44). Taken together, our findings indicated that overexpression of miR-106a exerted tumor suppression effects through inducing apoptosis and inhibiting proliferation, migration, and invasion by targeting IL-8 in PCa. Therefore, miR-106a could act as a tumor suppressor in PCa progression.
Overall, this study sheds light on the emerging role of miR-106a in PCa progression. Our study indicates that miR-106a could exert anti-tumor effects and the overexpression of miR-106a induce apoptosis and suppress proliferation, migration, and invasion of PCa cells by directly targeting IL-8. Therefore, the modulation of IL-8 by using miR-106a could be a promising therapeutic strategy for overcoming the progression of PCa.
Acknowledgments
Funding: This work was supported by
Footnote
Conflicts of Interest: All authors have completed the ICMJE uniform disclosure form (available at http://dx.doi.org/10.21037/tcr.2020.03.70). The authors have no conflicts of interest to declare.
Ethical Statement: The authors are accountable for all aspects of the work in ensuring that questions related to the accuracy or integrity of any part of the work are appropriately investigated and resolved. This study was basic research and did not involve any patient sample and animal. It was not required ethics approval.
Open Access Statement: This is an Open Access article distributed in accordance with the Creative Commons Attribution-NonCommercial-NoDerivs 4.0 International License (CC BY-NC-ND 4.0), which permits the non-commercial replication and distribution of the article with the strict proviso that no changes or edits are made and the original work is properly cited (including links to both the formal publication through the relevant DOI and the license). See: https://creativecommons.org/licenses/by-nc-nd/4.0/.
References
- Siegel RL, Miller KD, Jemal A. Cancer statistics 2018. CA Cancer J Clin 2018;68:7-30. [Crossref] [PubMed]
- Hanahan D, Weinberg RA. Hallmarks of cancer: the next generation. Cell 2011;144:646-74. [Crossref] [PubMed]
- Liu Q, Li A, Tian Y, et al. The CXCL8-CXCR1/2 pathways in cancer. Cytokine Growth Factor Rev 2016;31:61-71. [Crossref] [PubMed]
- Zhi F, Chen X, Wang S, et al. The use of hsa-miR-21, hsa-miR-181b and hsa-miR-106a as prognostic indicators of astrocytoma. Eur J Cancer 2010;46:1640-9. [Crossref] [PubMed]
- Sun X, Cheng G, Hao M, et al. CXCL12 / CXCR4 / CXCR7 chemokine axis and cancer progression. Cancer Metastasis Rev 2010;29:709-22. [Crossref] [PubMed]
- Zhang J, Lu Y, Pienta KJ. Multiple roles of chemokine (C-C motif) ligand 2 in promoting prostate cancer growth. J Natl Cancer Inst 2010;102:522-8. [Crossref] [PubMed]
- Araki S, Omori Y, Lyn D, et al. Interleukin-8 is a molecular determinant of androgen independence and progression in prostate cancer. Cancer Res 2007;67:6854-62. [Crossref] [PubMed]
- Waugh DJ, Wilson C. The interleukin-8 pathway in cancer. Clin Cancer Res 2008;14:6735-41. [Crossref] [PubMed]
- Murphy C, McGurk M, Pettigrew J, et al. Nonapical and cytoplasmic expression of interleukin-8, CXCR1, and CXCR2 correlates with cell proliferation and microvessel density in prostate cancer. Clin Cancer Res 2005;11:4117-27. [Crossref] [PubMed]
- Seaton A, Scullin P, Maxwell PJ, et al. Interleukin-8 signaling promotes androgen-independent proliferation of prostate cancer cells via induction of androgen receptor expression and activation. Carcinogenesis 2008;29:1148-56. [Crossref] [PubMed]
- Wilson C, Wilson T, Johnston PG, et al. Interleukin-8 signaling attenuates TRAIL- and chemotherapy-induced apoptosis through transcriptional regulation of c-FLIP in prostate cancer cells. Mol Cancer Ther 2008;7:2649-61. [Crossref] [PubMed]
- Maxwell PJ, Coulter J, Walker SM, et al. Potentiation of inflammatory CXCL8 signaling sustains cell survival in PTEN-deficient prostate carcinoma. Eur Urol 2013;64:177-88. [Crossref] [PubMed]
- Bartel DP. MicroRNAs: genomics, biogenesis, mechanism, and function. Cell 2004;116:281-97. [Crossref] [PubMed]
- Kim VN. MicroRNA biogenesis: coordinated cropping and dicing. Nat Rev Mol Cell Biol 2005;6:376-85. [Crossref] [PubMed]
- Kim YK, Kim VN. Processing of intronic microRNAs. Embo J 2007;26:775-83. [Crossref] [PubMed]
- Zeng Y, Yi R, Cullen BR. MicroRNAs and small interfering RNAs can inhibit mRNA expression by similar mechanisms. Proc Natl Acad Sci U S A 2003;100:9779-84. [Crossref] [PubMed]
- Hwang HW, Mendell JT. MicroRNAs in cell proliferation, cell death, and tumorigenesis. Br J Cancer 2006;94:776-80. [Crossref] [PubMed]
- Caruso DJ, Carmack AJ, Lokeshwar VB, et al. Osteopontin and interleukin-8 expression is independently associated with prostate cancer recurrence. Clin Cancer Res 2008;14:4111-8. [Crossref] [PubMed]
- Lu J, Getz G, Miska EA, et al. MicroRNA expression profiles classify human cancers. Nature 2005;435:834-8. [Crossref] [PubMed]
- Volinia S, Calin GA, Liu CG, et al. A microRNA expression signature of human solid tumors defines cancer gene targets. Proc Natl Acad Sci U S A 2006;103:2257-61. [Crossref] [PubMed]
- Calin GA, Croce CM. MicroRNA-cancer connection: the beginning of a new tale. Cancer Res 2006;66:7390-4. [Crossref] [PubMed]
- Croce CM. miRNAs in the spotlight: Understanding cancer gene dependency. Nat Med 2011;17:935-6. [Crossref] [PubMed]
- Slack FJ, Weidhaas JB. MicroRNA in cancer prognosis. N Engl J Med 2008;359:2720-2. [Crossref] [PubMed]
- Trang P, Weidhaas JB, Slack FJ. MicroRNAs as potential cancer therapeutics. Oncogene 2008;27:S52-7. [Crossref] [PubMed]
- Ambs S, Prueitt RL, Yi M, et al. Genomic profiling of microRNA and messenger RNA reveals deregulated microRNA expression in prostate cancer. Cancer Res 2008;68:6162-70. [Crossref] [PubMed]
- Porkka KP, Pfeiffer MJ, Waltering KK, et al. MicroRNA expression profiling in prostate cancer. Cancer Res 2007;67:6130-5. [Crossref] [PubMed]
- Schaefer A, Jung M, Mollenkopf HJ, et al. Diagnostic and prognostic implications of microRNA profiling in prostate carcinoma. Int J Cancer 2010;126:1166-76. [PubMed]
- Liu C, Kelnar K, Vlassov AV, et al. Distinct microRNA expression profiles in prostate cancer stem/progenitor cells and tumor-suppressive functions of let-7. Cancer Res 2012;72:3393-404. [Crossref] [PubMed]
- Alhasan AH, Scott AW, Wu JJ, et al. Circulating microRNA signature for the diagnosis of very high-risk prostate cancer. Proc Natl Acad Sci U S A 2016;113:10655-60. [Crossref] [PubMed]
- Chen X, Gong J, Zeng H, et al. MicroRNA145 targets BNIP3 and suppresses prostate cancer progression. Cancer Res 2010;70:2728-38. [Crossref] [PubMed]
- Hayashita Y, Osada H, Tatematsu Y, et al. A polycistronic microRNA cluster, miR-17-92, is overexpressed in human lung cancers and enhances cell proliferation. Cancer Res 2005;65:9628-32. [Crossref] [PubMed]
- Hossain A, Kuo MT, Saunders GF. Mir-17-5p regulates breast cancer cell proliferation by inhibiting translation of AIB1 mRNA. Mol Cell Biol 2006;26:8191-201. [Crossref] [PubMed]
- He L, Thomson JM, Hemann MT, et al. A microRNA polycistron as a potential human oncogene. Nature 2005;435:828-33. [Crossref] [PubMed]
- Mendell JT. miRiad roles for the miR-17-92 cluster in development and disease. Cell 2008;133:217-22. [Crossref] [PubMed]
- Chen K, Pan G. Dysregulation of microRNA-106a-5p-RUNX1 axis associates with clinical progression and prognosis of osteosarcoma patients. Pathol Res Pract 2019;215:152686. [Crossref] [PubMed]
- Huh JH, Kim TH, Kim K, et al. Dysregulation of miR-106a and miR-591 confers paclitaxel resistance to ovarian cancer. Br J Cancer 2013;109:452-61. [Crossref] [PubMed]
- Kim BH, Hong SW, Kim A, et al. Prognostic implications for the high expression of oncogenic microRNAs in advanced gastric carcinoma. J Surg Oncol 2013;107:505-10. [Crossref] [PubMed]
- Liu C, Song YH, Mao Y, et al. MiRNA-106a promotes breast cancer progression by regulating DAX-1. Eur Rev Med Pharmacol Sci 2019;23:1574-83. [PubMed]
- Wang Z, Liu M, Zhu H, et al. miR-106a is frequently upregulated in gastric cancer and inhibits the extrinsic apoptotic pathway by targeting FAS. Mol Carcinog 2013;52:634-46. [Crossref] [PubMed]
- Zhao S, Yang G, Mu Y, et al. MiR-106a is an independent prognostic marker in patients with glioblastoma. Neuro Oncol 2013;15:707-17. [Crossref] [PubMed]
- Zhu M, Zhang N, He S, et al. MicroRNA-106a targets TIMP2 to regulate invasion and metastasis of gastric cancer. FEBS Lett 2014;588:600-7. [Crossref] [PubMed]
- Yang G, Zhang R, Chen X, et al. MiR-106a inhibits glioma cell growth by targeting E2F1 independent of p53 status. J Mol Med (Berl) 2011;89:1037-50. [Crossref] [PubMed]
- Huang Q, Ma Q. MicroRNA-106a inhibits cell proliferation and induces apoptosis in colorectal cancer cells. Oncol Lett 2018;15:8941-4. [PubMed]
- Zhi F, Zhou G, Shao N, et al. miR-106a-5p inhibits the proliferation and migration of astrocytoma cells and promotes apoptosis by targeting FASTK. PLoS One 2013;8:e72390. [Crossref] [PubMed]
- Pan YJ, Wei LL, Wu XJ, et al. MiR-106a-5p inhibits the cell migration and invasion of renal cell carcinoma through targeting PAK5. Cell Death Dis 2017;8:e3155. [Crossref] [PubMed]
- Zhang Y, Lu Q, Cai X. MicroRNA-106a induces multidrug resistance in gastric cancer by targeting RUNX3. FEBS Lett 2013;587:3069-75. [Crossref] [PubMed]
- Singh RK, Lokeshwar BL. The IL-8-regulated chemokine receptor CXCR7 stimulates EGFR signaling to promote prostate cancer growth. Cancer Res 2011;71:3268-77. [Crossref] [PubMed]
- Hong Z, Hong H, Liu J, et al. miR-106a Is Downregulated in Peripheral Blood Mononuclear Cells of Chronic Hepatitis B and Associated with Enhanced Levels of Interleukin-8. Mediators Inflamm 2015;2015:629862.