Targeting the folate receptor for the treatment of ovarian cancer
Introduction
Ovarian cancer ranks as the ninth most common cancer in women, the second most common gynecological cancer and first for the most deaths due to gynecological cancers (1). The National Cancer Institute estimates that there were 22,000 new cases of ovarian cancer in 2014 in the U.S. alone. About 90% of ovarian tumors are epithelial in origin, with the remainder comprising germ and stromal tumors, and there are three major classified types of ovarian epithelial adenocarcinomas—serous, mucinous and endometrioid. Chemotherapy plays a major role in the treatment of patients with advanced ovarian cancer with platinum-based therapies being the mainstay of first-line treatment. However, most patients with advanced disease at diagnosis will have recurrence of their disease within 5 years. Patients whose disease recurs within 6 months of platinum-based therapy are referred to as “platinum-resistant” and these patients generally have a very poor outcome with a need for new treatment options (2). The overexpression of the folate receptor α (FRα) in epithelial ovarian cancers, especially in platinum-resistant patients, makes it an excellent target for new targeted therapies.
FRα is a glycosylphosphatidylinositol (GPI) anchored cell surface protein (3). It is distinct from the reduced folate carrier (RFC) (4) and the proton coupled folate transporter (PCFT) (5), which facilitate the bidirectional transport of reduced folate across membranes. In contrast, the family of receptors that includes FRα mediates the unidirectional transport of folates into cells.
FRα and FRβ share the highest degree of homology within the family of FRs, and have high affinity for physiological folates. The expression patterns of these two proteins are distinct. While FRβ expression is mostly limited to normal hematopoietic myeloid cells, FRα expression is limited to epithelial cells in the choroid plexus, proximal kidney tubules, fallopian tubes, uterus, epididymis, submandibular salivary gland, bronchial gland, acinar cells of the breast, type I and type II lung pneumocytes, and placenta (3,6-9). It is believed that the physiological role of FRα in the kidney is to scavenge folates back into the bloodstream (3), while its physiological functions in other FRα-positive tissues are largely unknown. Most normal tissues do not express FRα, and folate intake by cells and tissues is believed to be largely provided by the RFC.
Several studies have demonstrated FRα to be frequently expressed at high levels in ovarian cancer, in significant fractions of patients with non-small cell lung cancer (NSCLC) and in several other cancers (6,10-15). First identified as an ovarian-associated marker by immunohistochemical staining (16), the marker was later shown to be FRα (17,18). In an early study to evaluate FRα as a predictor of response to chemotherapy and survival, Toffoli et al. (19) noted that in patients with residual disease after surgery, higher levels of FRα expression were predictive of failure to respond to chemotherapy and subsequent lower survival outcomes. Immunohistochemistry (IHC) analysis of primary and recurrent ovarian tumors, including samples obtained from patients at diagnosis and later debulking surgery or simultaneous metastatic lesions demonstrated FRα expression in 70-80% of primary and recurrent tumors (15). Metastatic foci were similar to their matched primary tumor and recurrent tumors generally matched the expression status at diagnosis. In addition, FRα staining of samples from patients with epithelial ovarian or endometrial cancer showed no significant change in FRα expression following chemotherapy (20).
Targeting FR
The overexpression of FRα in certain cancer indications has led to great interest in using the receptor as a target for potential anticancer therapies. Two major targeting approaches have been pursued. One approach is to take advantage of the high affinity of FRs for folate and folate analogs. Folic acid (vitamin B9) binds to the FR with high affinity and is capable of targeting covalently attached payloads specifically to FR-positive cells (21). The first class of payloads evaluated for delivery through the FR were folate-protein conjugates, and these protein payloads were shown to be delivered into the target cell by natural receptor-mediated endocytosis (21-24). Functional assessment of a folate-protein toxin conjugate demonstrated that the conjugate could reach the cytoplasm of a target cell in a functionally active form (25). FR-mediated delivery of radiopharmaceuticals for imaging and radiation therapy has also been evaluated (26), as well as delivery of antisense oligonucleotides (27).
Delivery of cytotoxic drugs through FRs was first demonstrated by the targeted uptake of liposome-encapsulated drug molecules. Incorporation of folate constructs onto the surface of drug-loaded liposomes was shown to dramatically improve the potency and specificity of the liposomes against FR-positive tumor cells (28,29). The first description of direct folate-cytotoxic drug conjugates was by Ladino et al. (30), where the potency and specificity of folate-maytansinoid conjugates was evaluated. These conjugates showed high affinity for the FR, internalized via a folate-receptor mediated pathway, and demonstrated high specific cytotoxic potency toward folate-positive tumor cells in vitro. Later studies demonstrated marked FR-dependent antitumor activity of folate-maytansinoid conjugates in tumor xenograft models in mice at doses that were well tolerated, while a non-targeted maytansinoid was not active even at is maximum tolerated dose (MTD) (31). Folate-conjugates of many cytotoxic agents have been evaluated including 5-FU analogs (32), carboplatin analogues (33), mitomycin C (34), paclitaxel-loaded dendrimer nanodevices (35), thiolate histone deactylase prodrugs (36), camptothecin (37), rhaponticin (38), and drug-loaded magnetic nanoparticles (39). A novel folate conjugate with a vinblastine analog (EC140) was demonstrated to have antitumor activity against FR-positive tumors in mouse models (40). Further linker optimization studies with this vinca alkaloid payload identified an optimal design utilizing a disulfide-bond containing linker, EC145, which demonstrated a higher therapeutic index with improved activity and tolerability compared to EC140 (41). This optimized conjugate (illustrated in Figure 1), denoted vintafolide, has moved into clinical testing and is described in more detail below. Clinical evaluation of an additional folate-cytotoxic conjugate with a more potent tubulin targeting payload, EC1456, has also been initiated (42). This folic acid-tubulysin B hydrazide conjugate is an order of magnitude more potent against FRα-positive cells in vitro than EC145 (43).
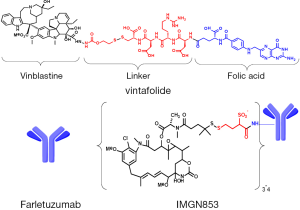
A second targeting approach has used FRα-binding antibodies, antibody-like fragments, or other antibody-like binding moieties to deliver anti-tumor activity in various formats. Early approaches included radioimmunotherapy with radioisotope-labeled chimeric anti-FRα antibody (44-46) and an anti-FRα single chain variable fragment antibody (scFv)-interleukin-2 (IL-2) fusion protein which reduced tumor volumes in FRα-positive xenograft tumors in mice by stimulating lymphocyte proliferation (47). Other targeted immunotherapy concepts have been evaluated including folate targeting of haptens, such as conjugated fluorescein or dinitrophenyl, to cancer cells to mobilize immune response against poorly immunogenic tumors (48,49). A bispecific antibody approach for FRα-directed autologous T cells targeting FRα and CD3 in combination with IL-2 provided promising data for adoptive T cell transfer approaches (50). Intraperitoneal (IP) delivery resulted in significant clinical responses with a toxicity profile most related to IL-2 administration. More recently, FRα has emerged as an attractive target utilizing the chimeric antibody receptor (CAR)-T lymphocyte approach. CARs are fusion molecules comprised of an extracellular binding domain (often an scFv) joined through a peptide linker to an intracellular lymphocyte signaling domain or domains which can mediate T cell activation. Early efforts with this approach have yielded exciting clinical results against a variety of tumor types and targeting a variety of tumor-associated antigens (51). However, a first phase I study using FRα-targeting CAR-T cells did not show anti-tumor activity, though the poor results may likely be explained by technical limitations in the study, such as low expression of the CAR construct and short persistence of the transferred T cells (52). A novel trial design to maximize the efficacy and safety using adoptive transfer of FRα-targeted CAR-T cells for the treatment of ovarian cancer has been proposed (53). In addition, efforts to increase the specificity of these adoptive transfer approaches using bispecific targeting, including FRα as a target have been evaluated in preclinical studies (54).
Clinical studies are ongoing with two FRα-targeting monoclonal antibody-based therapeutic approaches and are discussed in detail below. Farletuzumab (illustrated in Figure 1) is a humanized high affinity FRα-binding antibody that is thought to mediate tumor cell cytotoxicity principally through antibody-dependent cellular cytotoxicity (ADCC) and complement-dependent cytotoxicity (CDC) (55). The most recent FRα-directed therapy to enter clinical trials is the antibody-drug conjugate (ADC) IMGN853 (illustrated in Figure 1). IMGN853 comprises a humanized anti-FRα antibody conjugated with the cytotoxic maytansinoid DM4, through a hydrophilic disulfide-containing linker [unpublished data, (56)]. Such maytansinoid conjugates bind to their target antigen on the surface of cancer cells and are internalized, whereupon release of the cytotoxic payload causes disruption of microtubule function, cell cycle arrest and subsequent apoptosis (57).
Vintafolide
Vintafolide (also known as EC145) is a water-soluble folate-vinca alkaloid conjugate consisting of a hydrophilic folate-peptide conjugated to the microtubule destabilizing agent, desacetylvinblastine monohydrate (DAVLBH), via a disulfide-bond containing cleavable linker. The compound disrupts the formation of the mitotic spindle, which results in cell cycle arrest and subsequently cell death. Folate-drug conjugates bind to a FR and enter the cell via endocytosis (21), while antifolate drugs such as pemetrexed and methotrexate enter cells via the low affinity RFC. Vintafolide is not a substrate for RFC and therefore targets only FR-expressing cells.
Target-dependent antitumor activity of vintafolide was demonstrated against FR-positive tumor xenograft models (58), though the level of FR expression in these models was not correlated to that observed in patient samples. No significant antitumor activity was observed if animals were co-administered excess folate and the unconjugated vinca alkaloid was inactive at nontoxic dose levels and only marginally active at highly toxic dose levels. As expected due to the rapid clearance of this small molecule conjugate, frequent repeat dosing improved in vivo efficacy.
The observations regarding the pharmacokinetics (PK) and efficacy of vintafolide supported a frequent dosing schedule, and the first-in-human phase I trial evaluated a dosing schedule with treatment on days 1, 3, 5, 15, 17, 19 on a 28-day cycle (59). Two modes of IV administration were evaluated in the phase I trial, however, MTD was found to be the same for both bolus injection and 1-hour infusion. The bolus injection mode was chosen for further clinical development likely due to patient convenience.
Thirty two patients were enrolled and treated with vintafolide in the phase I study, with patients having had a median of eight prior therapies (range, 2-22), generally including a platinum-based regimen. About two thirds of the patients had also received prior radiotherapy. In this first trial, patients were not preselected for FRα expression based on concerns over the limitation of FRα IHC evaluations due to tumor heterogeneity, antibody specificity and staining reproducibility. No assessment of FRα expression was reported for these patients.
The MTD for vintafolide was determined to be 2.5 mg/kg when administered as a bolus injection or by infusion. Constipation was the dose-limiting toxicity (DLT) with the most common adverse events (AEs) being constipation, nausea, fatigue, and vomiting. These gastrointestinal toxicities are similar to those described for unconjugated vinca alkaloids (60) suggesting exposure of the GI tract to active metabolites from vintafolide during excretion, a phenomenon which has been demonstrated in preclinical models (61). Toxicity assessment reported for rodents and dogs defined the DLT of vintafolide to be gastrointestinal and hematologic toxicities, though clinically the hematologic toxicities characteristic of unconjugated vinca alkaloids may be reduced with vintafolide with no significant grade 3 or 4 thrombocytopenia or granulocytopenia observed. The level of neuropathy appeared characteristic of vinblastine, which is generally less nerve toxic, than vincristine. Evaluation of exposure-toxicity relationships using a population PK model identified body surface area as a significant covariate for vintafolide clearance, with reduced clearance and subsequent increased overall exposure being a significant predictor for the incidence of vintafolide-induced constipation (62). Clearance and AUC were not predictors of peripheral neuropathy, however.
Limited signs of efficacy were observed in the phase I trial. Seven patients had stable disease (SD) for 42-211 days during therapy, with one of these patients showing decreases in CA125 levels. One partial response (PR) with duration of 111 days was observed in a patient with metastatic ovarian cancer, also accompanied by a decrease in CA125 levels.
Given the potential importance of FR-targeting to the activity of vintafolide as demonstrated in preclinical models (58), the lack of screening patients for receptor expression may have significantly limited the overall level of efficacy observed in the phase I study. To address the relationship of target expression with therapeutic response, a phase II study using a companion SPECT-based imaging approach was conducted (63). Etarfolatide is a 99mTechnetium(Tc)-labeled folic acid conjugate imaging agent with a half-life and FR binding profile similar to vintafolide. Patients were imaged with 99mTc-etarfolatide prior to treatment with vintafolide. Forty nine patients were enrolled in the study, with 43 of these patients evaluable for efficacy. Eighty-four percent of the patients were visually positive for 99mTc-etarfolatide uptake in at least one tumor lesion. Overall, tumor lesions with positive uptake were more likely to show a decrease or remain stable in size compared to FR-negative lesions (56.4% vs. 20.7%), though there did not appear to be a correlation between higher levels of etarfolatide uptake and improved response. Overall response rate (ORR), disease control rate (DCR = responses + SD) and overall survival (OS) were assessed for patients with 100% of their lesions being imaging positive (high), 10-90% positive (intermediate), or 0% positive (negative). Two PRs were observed, with one in a patient identified to be 100% FR positive and the other in a patient with 50% positive lesions. The data suggested a correlation of FR positivity and DCR, where patients in the high FR group (n=14) had a DCR of 57% compared to 36% and 33% in the intermediate FR (n+22) and negative FR (n=3) groups, respectively. A trend to longer survival was also observed in patients with higher FR positivity (14.6, 9.6, and 3.0 months in the three groups, respectively). One concern with the analysis is the small number of patients representing the analysis groups (for example, the negative FR group with only three patients).
The toxicity profile of vintafolide identified during its clinical evaluation suggested the possibility of combination with certain standard-of-care anticancer drugs. Combination of vintafolide with pegylated liposomal doxorubicin (PLD) and other anticancer agents was evaluated in vitro and in vivo (64). Antitumor effects of vintafolide in combination with PLD, carboplatin, cisplatin, paclitaxel, docetaxel, topotecan, or irinotecan were greater than the single chemotherapeutic agents or vintafolide alone.
Based on the preclinical combination data, a randomized phase II trial (PRECEDENT) comparing vintafolide and PLD in combination vs. PLD alone was conducted in patients with platinum-resistant ovarian cancer (65). The primary objective was to compare progression-free survival (PFS) between the two groups. Patients with recurrent platinum-resistant ovarian cancer with two or less prior cytotoxic regimens (n=149) were randomized 2:1 to the combination arm or the PLD alone arm, respectively. The combination treatment was generally well tolerated with the frequency of leukopenia, neutropenia, abdominal pain and peripheral sensory neuropathy statistically higher in the combination arm than in the PLD alone arm. The FR-targeting imaging agent, etarfolatide, was evaluated as a biomarker for response. Overall, the median PFS was 5 months for the combination regimen and 2.7 months for PLD alone (hazard ratio 0.63; 95% confidence interval, 0.41-0.96; P=0.013). Interestingly, while the median PFS in patients with 100% etarfolatide-positive lesions was similar to the overall PFS in the combination arm (5.5 vs. 5 months), the median PFS in the PLD alone arm for patients with high FR was only 1.5 months, compared to the 2.7 months for all patients in this arm. The data suggest a possible correlation of FR expression with poor outcome in the chemotherapy alone arm and indeed previous analyses have suggested that FR expression may be a negative predictor of chemotherapy response (12,19). However, it is important to note that the numbers of patients in these subsets are likely too small for correlative assertions.
The finding of improved PFS for the patients with 100% etarfolatide-positive tumor lesions in the combination arm compared to PLD alone led to the initiation of a phase III randomized, double-blind clinical trial (PROCEED) evaluating vintafolide in combination with PLD compared to PLD plus placebo in patients with platinum-resistant ovarian cancer (NCT01170650). Patients included in this trial were required to have FR expression in all tumor lesions by etarfolatide imaging. The primary endpoint was PFS. The trial was stopped in early 2014 based on a pre-specified interim futility analysis which demonstrated that vintafolide did not meet the pre-specified PFS outcomes.
Exploration of vintafolide for the treatment of FRα-positive tumors continues with a randomized phase II combination trial in NSCLC (NCT01577654) and preclinical and clinical evaluation of other folate-drug conjugates with different cytotoxic moieties are also on-going.
Farletuzumab
Farletuzumab (MORAb-003) is a humanized monoclonal antibody with high affinity for FRα developed from the murine LK26 antibody using by a whole cell mutagenesis screening approach (66). Farletuzumab was shown to modulate the folate-dependent growth of FRα-transfected cells and mediate tumor cell cytotoxicity via CDC and ADCC. The antibody showed similar limited binding to normal tissues in human and monkeys, with no adverse findings upon repeated dosing in toxicity studies in monkeys.
The role of ADCC in the activity of farletuzumab was evaluated by comparing the activity of wild type farletuzumab with a mutated version that showed similar binding to FRα, but greatly reduced ADCC activity (55). In an orthotopic mouse model with IP implantation of FRα-positive IGROV-1 human ovarian adenocarcinoma cells, the ADCC-impaired mutant farletuzumab had no anti-tumor activity compared to significant tumor reduction by the wild type antibody, suggesting that in this in vivo model, ADCC-mediated cytotoxicity plays a major role in the anti-tumor activity of farletuzumab. Indeed, farletuzumab was shown to have no direct impact on the binding of folic acid and its analogs, and did not affect cell viability nor the cell growth inhibition induced by the anti-folates in in vitro evaluations (67).
The anti-tumor efficacy of farletuzumab in preclinical studies provided the rationale for initiation of the clinical evaluation of the FRα-targeting antibody. A first-in-human phase I study was conducted in patients with platinum-refractory or -resistant epithelial ovarian cancer. Twenty-five patients received farletuzumab dosed every week for 4 weeks in a 5-week cycle up to a maximal administered dose of 400 mg/m2. No DLTs were observed and the MTD was not reached. The most common treatment-related AEs were hypersensitivity reactions (60%), fatigue (48%) and diarrhea (16%). None of the toxicity findings seemed to be indicative of FRα-targeting of normal tissue. No objective responses were observed; however, nine patients had SD and four patients had reductions in CA-125 levels, with one patient showing a progressive decline in CA125 to 43% of baseline over 3 months. Three patients with SD received treatment for up to three cycles with mean target lesion decreases of 3-17%.
The clinical activity of farletuzumab was also evaluated alone and combined with carboplatin and a taxane (paclitaxel or docetaxel) in a phase II study for 54 patients with first-relapse, platinum-sensitive ovarian cancer (68). Farletuzumab was given weekly every 21 days and subjects receiving single agent farletuzumab could receive the combination therapy after single-agent progression. In the combination arm, the ORR was 75% with 21% of patients having a longer progression-free interval (PFI) than on their first response to chemotherapy. This compared favorably to the historical rate for improved PFI (3%) with retreatment with chemotherapy alone (69). In addition, there was a high rate of response among patients with <12 months first response.
A population PK model for farletuzumab was developed using concentration/time data from the phase I and phase II trials with 79 advanced ovarian cancer patients (70). The PK parameters of farletuzumab are similar to those of other IgG monoclonal antibodies. Body weight was identified as a covariate that explained inter-patient variability in clearance and volume of distribution.
Results from the phase II study suggested that farletuzumab in combination with conventional platinum-based therapies may improve the duration of a second response to chemotherapy. Therefore, a large randomized phase III, FAR-131, was undertaken to confirm these results. Patients were enrolled into three parallel groups and received placebo or farletuzumab at 1.25 or 2.5 mg/kg. Following the completion of carboplatin/taxane therapy, maintenance treatment with weekly farletuzumab or placebo was continued until disease progression. Patients were stratified based on length of first remission, route of administration of first line therapy (IP or IV) and taxane received (paclitaxel or docetaxel). Results of the phase III trial, however, found that the combination of farletuzumab with platinum/taxane therapy did not meet the primary objective of improved PFS. Additional analyses and testing will be required to determine whether farletuzumab may improve outcome for patients with FRα-positive disease and to that end, a phase II study in patients with low CA125 platinum-sensitive recurrent ovarian cancer is planned (NCT02289950).
IMGN853
IMGN853 is a FRα-targeting ADC, consisting of an anti-FRα antibody coupled to a highly potent cytotoxic maytansinoid payload [unpublished data, (56)]. Delivery of the maytansinoid payload by the ADC into a target cell results in the release of the active payload, cell cycle arrest and cell death due to disruption of microtubule dynamics (57,71). The anti-FRα monoclonal antibody chosen for the ADC is a humanized version of Mov19 (16,18), M9346A. This antibody was the most effective, out of a large panel of antibodies evaluated, for antigen-selective delivery of a maytansinoid payload into FRα-positive cells. Evaluation of conjugates of M9346A with various linkers and maytansinoid moieties demonstrated that conjugation with the N2'-deacetyl-N2'-(4-mercapto-4-methyl-1-oxopentyl)-maytansine (DM4) payload, using the N-succinimidyl 4-(2-pyridyldithio)-2-sulfobutanoate (sulfo-SPDB) linker, yielded the most potent conjugate overall (denoted as IMGN853). The level of expression of FRα on the surface of cells was found to be a major determinant of sensitivity of tumor cells to IMGN853. Efficacy studies of IMGN853 in xenografts of ovarian cancer and NSCLC cell lines and of a patient-derived NSCLC tumor xenograft model demonstrated that the ADC was highly active against tumors that expressed FRα at levels similar to that found on a large fraction of ovarian and NSCLC patient tumors, as assessed by IHC. IMGN853 displayed cytotoxic activity against FRα-negative cancer cells situated near FRα-positive cancer cells (bystander cytotoxic activity), providing mechanistic explanation for its ability to eradicate tumors with heterogeneous expression of FRα. These findings supported initiation of clinical development of IMGN853 as a novel targeted therapy for patients with FRα-expressing tumors.
In an ongoing first-in-human phase I study, 30 patients have been treated with IMGN853 across seven dose levels (0.5 to 7.0 mg/kg based on total body weight, dosed once every 21 days) (56). Patients with any type of FRα -expressing, refractory solid tumor were enrolled. A dose-limiting reversible ocular toxicity, thought to be related to the maytansinoid ADC format and not FRα-target related, was associated with high peak exposure levels. Covariate analysis indicated a correlation between body weight and Cmax. PK modeling suggested that dosing by adjusted ideal body weight (AIBW) instead of total body weight would result in a decrease in inter-patient PK variability allowing maximal IMGN853 exposure levels while keeping exposures below those associated with ocular toxicity. In addition, modeling also suggested that a modified weekly dosing schedule (dosing weekly for 3 weeks in a 4-week cycle) would lead to an increase in overall exposure while keeping peak levels below those associated with ocular toxicity. An evaluation of the potential benefit of AIBW dosing on an every 3 weeks and a modified weekly schedule is currently ongoing in the phase I clinical trial. Preliminary evidence of clinical activity (CA125 Gynecologic Cancer Intergroup response criteria, PR, and SD ≥6 cycles) was reported for 10 of 24 evaluable patients receiving doses ≥3.3 mg/kg (TBW). In patients at higher exposure levels (AUC0-∞ at or above 13,000 h µg/mL), clinical activity was observed in 5 of 6 serous or transitional epithelial ovarian cancer patients and 2 of 4 endometrial patients.
Conclusions
The development of therapies that target FRα remains an active area. The extensive evaluation of the multitude of compounds that bind to FRα, either through the folate binding site or using antibody-based approaches has paved the way for the development of a new generation of FRα-targeting agents. The increased potency of the most recent FRα-directed therapies, such as EC1456 and IMGN853, may be the key to the development of successful FRα-targeting agents that improve the clinical outcome for patients with ovarian cancer.
Acknowledgments
The author acknowledges the members of the IMGN853 development team within ImmunoGen and their clinical investigators for their contribution to this review. Valuable manuscript review contributions were provided by V. Goldmacher, O. Ab, Carol Hausner and Rodrigo Ruiz Soto (ImmunoGen). Figure 1 was provided by R. Chari (ImmunoGen).
Funding: None.
Footnote
Provenance and Peer Review: This article was commissioned by the Guest Editors (Franco Muggia and Eleonora Teplinsky) for the series “Epithelial Ovarian Cancer Treatment: Integrating Molecular Targeting” published in Translational Cancer Research. The article has undergone external peer review.
Conflicts of Interest: The series “Epithelial Ovarian Cancer Treatment: Integrating Molecular Targeting” was commissioned by the editorial office without any funding or sponsorship. R.J. Lutz is an employee of ImmunoGen, Inc. The authors have no other conflicts of interest to declare.
Ethical Statement: The author is accountable for all aspects of the work in ensuring that questions related to the accuracy or integrity of any part of the work are appropriately investigated and resolved.
Open Access Statement: This is an Open Access article distributed in accordance with the Creative Commons Attribution-NonCommercial-NoDerivs 4.0 International License (CC BY-NC-ND 4.0), which permits the non-commercial replication and distribution of the article with the strict proviso that no changes or edits are made and the original work is properly cited (including links to both the formal publication through the relevant DOI and the license). See: https://creativecommons.org/licenses/by-nc-nd/4.0/.
References
- American Cancer Society. Cancer Facts & Figures 2013. Atlanta: American Cancer Society; 2013.
- Luvero D, Milani A, Ledermann JA. Treatment options in recurrent ovarian cancer: latest evidence and clinical potential. Ther Adv Med Oncol 2014;6:229-39. [PubMed]
- Elnakat H, Ratnam M. Distribution, functionality and gene regulation of folate receptor isoforms: implications in targeted therapy. Adv Drug Deliv Rev 2004;56:1067-84. [PubMed]
- Matherly LH, Hou Z, Deng Y. Human reduced folate carrier: translation of basic biology to cancer etiology and therapy. Cancer Metastasis Rev 2007;26:111-28. [PubMed]
- Desmoulin SK, Hou Z, Gangjee A, et al. The human proton-coupled folate transporter: Biology and therapeutic applications to cancer. Cancer Biol Ther 2012;13:1355-73. [PubMed]
- Weitman SD, Lark RH, Coney LR, et al. Distribution of the folate receptor GP38 in normal and malignant cell lines and tissues. Cancer Res 1992;52:3396-401. [PubMed]
- Weitman SD, Weinberg AG, Coney LR, et al. Cellular localization of the folate receptor: potential role in drug toxicity and folate homeostasis. Cancer Res 1992;52:6708-11. [PubMed]
- Mantovani LT, Miotti S, Ménard S, et al. Folate binding protein distribution in normal tissues and biological fluids from ovarian carcinoma patients as detected by the monoclonal antibodies MOv18 and MOv19. Eur J Cancer 1994;30A:363-9. [PubMed]
- Stein R, Goldenberg DM, Mattes MJ. Normal tissue reactivity of four anti-tumor monoclonal antibodies of clinical interest. Int J Cancer 1991;47:163-9. [PubMed]
- O'Shannessy DJ, Somers EB, Maltzman J, et al. Folate receptor alpha (FRA) expression in breast cancer: identification of a new molecular subtype and association with triple negative disease. Springerplus 2012;1:22. [PubMed]
- O'Shannessy DJ, Somers EB, Smale R, et al. Expression of folate receptor-α (FRA) in gynecologic malignancies and its relationship to the tumor type. Int J Gynecol Pathol 2013;32:258-68. [PubMed]
- Toffoli G, Cernigoi C, Russo A, et al. Overexpression of folate binding protein in ovarian cancers. Int J Cancer 1997;74:193-8. [PubMed]
- Parker N, Turk MJ, Westrick E, et al. Folate receptor expression in carcinomas and normal tissues determined by a quantitative radioligand binding assay. Anal Biochem 2005;338:284-93. [PubMed]
- Christoph DC, Asuncion BR, Hassan B, et al. Significance of folate receptor alpha and thymidylate synthase protein expression in patients with non-small-cell lung cancer treated with pemetrexed. J Thorac Oncol 2013;8:19-30. [PubMed]
- Kalli KR, Oberg AL, Keeney GL, et al. Folate receptor alpha as a tumor target in epithelial ovarian cancer. Gynecol Oncol 2008;108:619-26. [PubMed]
- Miotti S, Canevari S, Ménard S, et al. Characterization of human ovarian carcinoma-associated antigens defined by novel monoclonal antibodies with tumor-restricted specificity. Int J Cancer 1987;39:297-303. [PubMed]
- Campbell IG, Jones TA, Foulkes WD, et al. Folate-binding protein is a marker for ovarian cancer. Cancer Res 1991;51:5329-38. [PubMed]
- Coney LR, Tomassetti A, Carayannopoulos L, et al. Cloning of a tumor-associated antigen: MOv18 and MOv19 antibodies recognize a folate-binding protein. Cancer Res 1991;51:6125-32. [PubMed]
- Toffoli G, Russo A, Gallo A, et al. Expression of folate binding protein as a prognostic factor for response to platinum-containing chemotherapy and survival in human ovarian cancer. Int J Cancer 1998;79:121-6. [PubMed]
- Despierre E, Lambrechts S, Leunen K, et al. Folate receptor alpha (FRA) expression remains unchanged in epithelial ovarian and endometrial cancer after chemotherapy. Gynecol Oncol 2013;130:192-9. [PubMed]
- Leamon CP, Low PS. Delivery of macromolecules into living cells: a method that exploits folate receptor endocytosis. Proc Natl Acad Sci U S A 1991;88:5572-6. [PubMed]
- Leamon CP, Low PS. Membrane folate-binding proteins are responsible for folate-protein conjugate endocytosis into cultured cells. Biochem J 1993;291:855-60. [PubMed]
- Kamen BA, Capdevila A. Receptor-mediated folate accumulation is regulated by the cellular folate content. Proc Natl Acad Sci U S A 1986;83:5983-7. [PubMed]
- Turek JJ, Leamon CP, Low PS. Endocytosis of folate-protein conjugates: ultrastructural localization in KB cells. J Cell Sci 1993;106:423-30. [PubMed]
- Leamon CP, Low PS. Cytotoxicity of momordin-folate conjugates in cultured human cells. J Biol Chem 1992;267:24966-71. [PubMed]
- Mathias CJ, Wang S, Lee RJ, et al. Tumor-selective radiopharmaceutical targeting via receptor-mediated endocytosis of gallium-67-deferoxamine-folate. J Nucl Med 1996;37:1003-8. [PubMed]
- Li S, Deshmukh HM, Huang L. Folate-mediated targeting of antisense oligodeoxynucleotides to ovarian cancer cells. Pharm Res 1998;15:1540-5. [PubMed]
- Lee RJ, Low PS. Folate-mediated tumor cell targeting of liposome-entrapped doxorubicin in vitro. Biochim Biophys Acta 1995;1233:134-44. [PubMed]
- Pan X, Lee RJ. Tumour-selective drug delivery via folate receptor-targeted liposomes. Expert Opin Drug Deliv 2004;1:7-17. [PubMed]
- Ladino CA, Chari RV, Bourret LA, et al. Folate-maytansinoids: target-selective drugs of low molecular weight. Int J Cancer 1997;73:859-64. [PubMed]
- Reddy JA, Westrick E, Santhapuram HK, et al. Folate receptor-specific antitumor activity of EC131, a folate-maytansinoid conjugate. Cancer Res 2007;67:6376-82. [PubMed]
- Liu J, Kolar C, Lawson TA, et al. Targeted drug delivery to chemoresistant cells: folic acid derivatization of FdUMP[10] enhances cytotoxicity toward 5-FU-resistant human colorectal tumor cells. J Org Chem 2001;66:5655-63. [PubMed]
- Aronov O, Horowitz AT, Gabizon A, et al. Folate-targeted PEG as a potential carrier for carboplatin analogs. Synthesis and in vitro studies. Bioconjug Chem 2003;14:563-74. [PubMed]
- Leamon CP, Reddy JA, Vlahov IR, et al. Synthesis and biological evaluation of EC72: a new folate-targeted chemotherapeutic. Bioconjug Chem 2005;16:803-11. [PubMed]
- Majoros IJ, Myc A, Thomas T, et al. PAMAM dendrimer-based multifunctional conjugate for cancer therapy: synthesis, characterization, and functionality. Biomacromolecules 2006;7:572-9. [PubMed]
- Suzuki T, Hisakawa S, Itoh Y, et al. Design, synthesis, and biological activity of folate receptor-targeted prodrugs of thiolate histone deacetylase inhibitors. Bioorg Med Chem Lett 2007;17:4208-12. [PubMed]
- Henne WA, Kularatne SA, Hakenjos J, et al. Synthesis and activity of a folate targeted monodisperse PEG camptothecin conjugate. Bioorg Med Chem Lett 2013;23:5810-3. [PubMed]
- Liang X, Sun Y, Zeng W, et al. Synthesis and biological evaluation of a folate-targeted rhaponticin conjugate. Bioorg Med Chem 2013;21:178-85. [PubMed]
- Balasubramanian S, Girija AR, Nagaoka Y, et al. Curcumin and 5-fluorouracil-loaded, folate- and transferrin-decorated polymeric magnetic nanoformulation: a synergistic cancer therapeutic approach, accelerated by magnetic hyperthermia. Int J Nanomedicine 2014;9:437-59. [PubMed]
- Leamon CP, Reddy JA, Vlahov IR, et al. Synthesis and biological evaluation of EC140: a novel folate-targeted vinca alkaloid conjugate. Bioconjug Chem 2006;17:1226-32. [PubMed]
- Leamon CP, Reddy JA, Vlahov IR, et al. Comparative preclinical activity of the folate-targeted Vinca alkaloid conjugates EC140 and EC145. Int J Cancer 2007;121:1585-92. [PubMed]
- Sausville E, Harb W, Ramanathan RK, et al. Phase 1 dose-escalation study of the folic acid-tubulysin small-molecule drug conjugate EC1456 in patients (pts) with advanced solid tumors. Ann Oncol 2014;25:iv146-64.
- Reddy JA, Bloomfield A, Nelson M, et al. Abstract 832: Pre-clinical development of EC1456: A potent Folate targeted Tubulysin SMDC. Cancer Research 2014;74:832.
- Buist MR, Kenemans P, den Hollander W, et al. Kinetics and tissue distribution of the radiolabeled chimeric monoclonal antibody MOv18 IgG and F(ab')2 fragments in ovarian carcinoma patients. Cancer Res 1993;53:5413-8. [PubMed]
- Molthoff CF, Buist MR, Kenemans P, et al. Experimental and clinical analysis of the characteristics of a chimeric monoclonal antibody, MOv18, reactive with an ovarian cancer-associated antigen. J Nucl Med 1992;33:2000-5. [PubMed]
- van Zanten-Przybysz I, Molthoff CF, Roos JC, et al. Radioimmunotherapy with intravenously administered 131I-labeled chimeric monoclonal antibody MOv18 in patients with ovarian cancer. J Nucl Med 2000;41:1168-76. [PubMed]
- Melani C, Figini M, Nicosia D, et al. Targeting of interleukin 2 to human ovarian carcinoma by fusion with a single-chain Fv of antifolate receptor antibody. Cancer Res 1998;58:4146-54. [PubMed]
- Lu Y, Low PS. Folate targeting of haptens to cancer cell surfaces mediates immunotherapy of syngeneic murine tumors. Cancer Immunol Immunother 2002;51:153-62. [PubMed]
- Lu Y, You F, Vlahov I, et al. Folate-targeted dinitrophenyl hapten immunotherapy: effect of linker chemistry on antitumor activity and allergic potential. Mol Pharm 2007;4:695-706. [PubMed]
- Canevari S, Stoter G, Arienti F, et al. Regression of advanced ovarian carcinoma by intraperitoneal treatment with autologous T lymphocytes retargeted by a bispecific monoclonal antibody. J Natl Cancer Inst 1995;87:1463-9. [PubMed]
- Kershaw MH, Westwood JA, Darcy PK. Gene-engineered T cells for cancer therapy. Nat Rev Cancer 2013;13:525-41. [PubMed]
- Kershaw MH, Westwood JA, Parker LL, et al. A phase I study on adoptive immunotherapy using gene-modified T cells for ovarian cancer. Clin Cancer Res 2006;12:6106-15. [PubMed]
- Kandalaft LE, Powell DJ Jr, Coukos G. A phase I clinical trial of adoptive transfer of folate receptor-alpha redirected autologous T cells for recurrent ovarian cancer. J Transl Med 2012;10:157. [PubMed]
- Lanitis E, Poussin M, Klattenhoff AW, et al. Chimeric antigen receptor T Cells with dissociated signaling domains exhibit focused antitumor activity with reduced potential for toxicity in vivo. Cancer Immunol Res 2013;1:43-53. [PubMed]
- Lin J, Spidel JL, Maddage CJ, et al. The antitumor activity of the human FOLR1-specific monoclonal antibody, farletuzumab, in an ovarian cancer mouse model is mediated by antibody-dependent cellular cytotoxicity. Cancer Biol Ther 2013;14:1032-8. [PubMed]
- Moore KN, Ponte J, LoRusso P, et al. Relationship of pharmacokinetics (PK), toxicity, and initial evidence of clinical activity with IMGN853, a folate receptor alpha (FRa) targeting antibody drug conjugate in patients (Pts) with epithelial ovarian cancer (EOC) and other FRa-positive solid tumors. J Clin Oncol 2014;32:abstr 5571.
- Lambert JM. Drug-conjugated antibodies for the treatment of cancer. Br J Clin Pharmacol 2013;76:248-62. [PubMed]
- Reddy JA, Dorton R, Westrick E, et al. Preclinical evaluation of EC145, a folate-vinca alkaloid conjugate. Cancer Res 2007;67:4434-42. [PubMed]
- Lorusso PM, Edelman MJ, Bever SL, et al. Phase I study of folate conjugate EC145 (Vintafolide) in patients with refractory solid tumors. J Clin Oncol 2012;30:4011-6. [PubMed]
- Moudi M, Go R, Yien CY, et al. Vinca alkaloids. Int J Prev Med 2013;4:1231-5. [PubMed]
- Leamon CP, Reddy JA, Klein PJ, et al. Reducing undesirable hepatic clearance of a tumor-targeted vinca alkaloid via novel saccharopeptidic modifications. J Pharmacol Exp Ther 2011;336:336-43. [PubMed]
- Li J, Sausville EA, Klein PJ, et al. Clinical pharmacokinetics and exposure-toxicity relationship of a folate-Vinca alkaloid conjugate EC145 in cancer patients. J Clin Pharmacol 2009;49:1467-76. [PubMed]
- Morris RT, Joyrich RN, Naumann RW, et al. Phase II study of treatment of advanced ovarian cancer with folate-receptor-targeted therapeutic (vintafolide) and companion SPECT-based imaging agent (99mTc-etarfolatide). Ann Oncol 2014;25:852-8. [PubMed]
- Reddy JA, Dorton R, Bloomfield A, et al. Rational combination therapy of vintafolide (EC145) with commonly used chemotherapeutic drugs. Clin Cancer Res 2014;20:2104-14. [PubMed]
- Naumann RW, Coleman RL, Burger RA, et al. PRECEDENT: a randomized phase II trial comparing vintafolide (EC145) and pegylated liposomal doxorubicin (PLD) in combination versus PLD alone in patients with platinum-resistant ovarian cancer. J Clin Oncol 2013;31:4400-6. [PubMed]
- Ebel W, Routhier EL, Foley B, et al. Preclinical evaluation of MORAb-003, a humanized monoclonal antibody antagonizing folate receptor-alpha. Cancer Immun 2007;7:6. [PubMed]
- Kamen BA, Smith AK. Farletuzumab, an anti-folate receptor α antibody, does not block binding of folate or anti-folates to receptor nor does it alter the potency of anti-folates in vitro. Cancer Chemother Pharmacol 2012;70:113-20. [PubMed]
- Armstrong DK, White AJ, Weil SC, et al. Farletuzumab (a monoclonal antibody against folate receptor alpha) in relapsed platinum-sensitive ovarian cancer. Gynecol Oncol 2013;129:452-8. [PubMed]
- Markman M, Markman J, Webster K, et al. Duration of response to second-line, platinum-based chemotherapy for ovarian cancer: implications for patient management and clinical trial design. J Clin Oncol 2004;22:3120-5. [PubMed]
- Farrell C, Schweizer C, Wustner J, et al. Population pharmacokinetics of farletuzumab, a humanized monoclonal antibody against folate receptor alpha, in epithelial ovarian cancer. Cancer Chemother Pharmacol 2012;70:727-34. [PubMed]
- Oroudjev E, Lopus M, Wilson L, et al. Maytansinoid-antibody conjugates induce mitotic arrest by suppressing microtubule dynamic instability. Mol Cancer Ther 2010;9:2700-13. [PubMed]