Ligustrazine inhibits the viability and motility of colon cancer cells
Introduction
Colon cancer, a gastrointestinal malignancy, is the third most frequently occurring cancer in the world (1). The Global Cancer Status Report in 2018 estimated that 18.1 million new cancer cases and 9.6 million related deaths would occur in 2018, with the morbidity and mortality of colorectal cancer as 6.1% and 9.2%, respectively (2). Between 2005 and 2014, cancer prevention, early diagnosis, and better treatment saw the incidence of colon cancer decrease by about 2.9% per year (3). Unfortunately, only a small number of patients with disseminated disease were able to undergo radical resection without relapse. Despite the improvements seen in relation to colon cancer, some studies have shown that individuals under 50 years of age have experienced an increase in incidence of colorectal cancer (4).
Ligustrazine (LSZ) is an alkaloid extracted from the rhizome of the traditional Chinese herb Ligusticum wallichii, which was demonstrated to hold the properties of inhibiting inflammation, ant fibrosis, and modulating the immune system (5,6). Moreover, Feng et al. found that LSZ was an effective blocker of vasoconstriction, scavenging oxygen free radicals, and the promotion of apoptosis in tubules (7), and further reported that LSZ inhibited apoptosis in ischemia-reperfusion injury and protected from hypoxic-ischemic brain damage (8). In addition, other research has demonstrated LSZ to have significant anti-tumor effects on melanoma, breast cancer, and prostate cancer (9-11). However, the role of LSZ in colon cancer is yet to be fully elucidated.
PI3K-AKT-mTOR pathway is currently considered to be a likely biological target that is commonly activated in a variety of human cancers (12). AKT controls cell growth via mTOR, regulating translation initiation, ribosome biogenesis, and cell cycle progression (13). In addition, epidermal growth factor receptor (EGFR), Ras and Raf mutation leads to downstream activation of the Smad-PI3K-Akt-mTOR pathway contributing to growth and metastasis in colorectal cancer. Thus, EGFR inhibitor has been introduced as an important means of treating metastasic colorectal cancer (14). Unfortunately, however, EGFR inhibitor is not able to shut down the pathway when the mutation happens downstream (15). A novel drug to target the PI3K-Akt-mToR downstream pathway is still needed in colorectal cancer treatment.
This study’s purpose was to elucidate the anti-tumor effect of LSZ in relation to colon cancer cells and its potential biological mechanism of modulating cell viability, cell motility, and epithelial mesenchymal transformation (EMT) involving the PI3K-AKT-mTOR pathway.
Methods
Cell culture
We obtained HCoEpiC (normal colonic epithelial cell) and SW480 (colon cancer cell) cell lines from American Type Culture Collection (ATCC, Manassas, USA) and maintained them in RPMI Media 1640 (Cat#: 11875-093, Gibco, USA) supplemented with 10% fetal bovine serum (Life Technologies Inc., USA). Cell culturing took place at 37 °C in a 5% CO2 atmosphere. All of the cells were treated with different concentrations of LSZ (0, 0.01, 0.025, 0.05, 0.1, 0.25, 0.5, 1, 1.5, 2, 2.5, 5, and 10 mM) for a 24-hour period.
Cell proliferation
SW480 cells were seeded for a short time in 96-well plates at a density of around 6×103 cells per well and treated with LSZ at 3 different concentrations (0.1, 0.5, and 1.5 mM) for 24, 48 and 72 h, respectively. Cell proliferation capacity was tested by CCK8 kit (Mskbio Technology Ltd., China) according to the manufacturer’s specification, and the 450 nm absorbance value was calculated.
Clone formation assay
Three different concentrations of LSZ (0.1, 0.5, and 1.5 mM) were treated for 72 hours with 3×102 cells, and then inoculated into 35 mm culture dishes and cultured over 14 days. PBS was used to rinse the cells, before the were fixed in 4% paraformaldehyde (Sigma, USA) at room temperature for a period of 15 min. Following this, the cells were incubated with Wright-Giemsa stain (Nanjing Jiancheng Bioengineering Institute, China) for 5 minutes. Finally, we collected images and counted colony numbers.
Flow cytometry
SW480 cells (cell number 1×106/mL) were treated with LSZ (0.1, 0.5 and 1.5 mM) and collected in a 10 mL centrifuge tube, before being centrifuged at 1,000 r/min for a period of 5 min. The culture solution was then disposed of, and the cells were washed once with buffer and centrifuged at 1,000 r/min for 5 minutes. Next, the incubation of 100 µL of resuspended cells took place at room temperature for 15 min in darkness. They were centrifuged at 1,000 r/min for 5 min and washed once with buffer. The fluorescent (SA-FLOUS) solution was then applied, before incubation at 4 °C for 20 min (avoiding light) and vibrating from time to time. Finally, the results were tested using a flow cytometer (Thermo Fisher Scientific, USA) within 1 hour.
Reverse transcription quantitative polymerase chain reaction (RT-qPCR)
The extraction of total RNA from cell lines and tumor tissues was conducted with the help of Trizol reagent (Invitrogen, USA), according to the instructions of the manufacturer. Extracted RNA was stored at –80 °C and eluted with RNase-free water. Relative mRNA level of Ki67, caspase-3, Bcl-2/Bax, E-cadherin, N-cadherin, Vimentin and α-SMA were detected by RT-qPCR using SYBR-green PCR Master Mix (TaKaRa, Japan) in a Fast Real-time PCR 7500 System (Applied Biosystems, USA). We sourced RT-qPCR primers from GeneCopoeia (SanDiego, USA). The sequences of the primers are presented in Table 1.
Table 1
Gene | Sequence |
---|---|
Ki67 | F: 5'-GCAGGACTTCACTTGCTTCC-3' |
R: 5'-TCATTTGCGTTTGTTTCACG-3' | |
caspase-3 | F: 5'-TGGAACAAATGGACCTGTTGACC-3' |
R: 5'-AGGACTCAAATTCTGTTGCCACC-3' | |
Bax | F: 5'-TCCACCAAGAAGCTGAGCGAG-3' |
R: 5'-GTCCAGCCCATGATGGTTCT-3' | |
Bcl-2 | F: 5'-TTCTTTGAGTTCGGTGGGGTC-3' |
R: 5'-TGCATATTTGTTTGGGGCAGG-3' | |
N-cadherin | F: 5'-ATTGTGGGTGCGGGGCTTGG-3' |
R: 5'-GGGTGTGGGGCTGCAGATCG-3' | |
E-cadherin | F: 5'-TGCCCAGAAAATGAAAAAGG-3' |
R: 5'-GTGTATGTGGCAATGCGTTC-3' | |
Vimentin | F: 5'-ATGACCGCTTCGCCAACTAC-3' |
R: 5'-CGGGCTTTGTCGTTGGTTAG-3' | |
α-SMA | F: 5'-ATGCTCCCAGGGCTGTTTTC-3' |
R: 5'-CTTTTGCTCTGTGCTTCGTC-3' | |
GAPHG | F: 5'-CCATCTTCCAGGAGCGAGAT-3' |
R: 5'-TGCTGATGATCTTGAGGCTG-3' |
RT-qPCR, reverse transcription quantitative polymerase chain reaction.
Western blotting
Lysing was carried out using lysis buffer (Beyotime, Shanghai, China). Protein extracts were resolved by SDS-page, before transferral to polyvinylidene fluoride (PVDF) membranes (Millipore, USA), blocking in 5% non-fat milk/TBST, and next incubation with corresponding primary antibodies against Ki67 (ab92742, 1:1,000), Cleaved-Caspase-3 (ab2302, 1:1,000), Bcl-2 (ab32124, 1:1,000), Bax (ab32503, 1:1,000), E-cadherin (ab15148, 1:1,000) and N-cadherin (ab76057, 1:1,000), Vimentin (ab45939, 1:1,000), α-SMA (ab32575, 1:1,000), PI3K (ab40755, 1:1,000), p-PI3K (ab18265, 1:1,000), AKT (ab8805, 1:1,000), p-AKT (ab38449, 1:1,000), mTOR (ab32028, 1:1,000), and p-mTOR (ab84400, 1:1,000) overnight at 4 °C. β-actin was served as an endogenous control and detected using a rabbit polyclonal (ab8226, 1:4,000). Following this, we carried out incubation of the secondary antibody of goat anti-rabbit IgG (ab6721, 1:1,000) for 1 hour at room temperature. After washing, signals were visualized using a ChemiDoc XRS imaging system and Quantity One analysis software (Bio-Rad, USA). All antibodies were obtained from Abcam (Cambridge, UK).
Transwell assays
The two invasion chambers containing Matrigel (1 mg/mL) (Becton-Dickinson, USA) were used to determine SW480 cell invasion. The upward chamber held 200 µL serum-free medium containing 1×105 cells/well, and the lower chamber held 0.6 mL medium containing 20% FBS. Following incubation at 37 °C for 24 hours, a cotton bud was used to remove non-invading cells on the upward membranes, and the migrated cells were fixed in 95% ethanol before staining with hematoxylin. Finally, the number of cells was counted with ImageJ software, and 10 fields of view were randomly selected under an inverted microscope.
Wound healing assay
SW480 cells were cultured using a 6-well culture plate with a density of 1×105 until the formation of fusion monolayer cells occurred. Then, the fusion monolayer cells were scraped off using a sterile 20 µL micro pipette, washed with PBS for three times, and cultured in serum-free medium for 0 and 24 hours. Next, three visible regions (200× magnification) were randomly selected with an inverted microscope. Finally, the open area ratios for 24 to 0 hours were calculated.
Mouse model
We obtained SPF BALB/c mice (male, 6 to 8 weeks old) from the Animal Center of Chengdu Anorectal Hospital. The mice ranged in weight from 18 to 20 g. All mice were housed in cages and kept under controlled environmental conditions (temperature 24±2 °C, humidity 60%±5%, and 12 h light/dark cycles). To produce xenografts, SW480 cells were inoculated into the colons of the mice via subcutaneous injection. By intraperitoneal injection, 150 mg/kg of LSZ (10) was administered to the mice 3 times a week for 30 days. All animal experiments were performed in accordance with the NIH Guide for the Care and Use of Laboratory Animals and were approved by the Medical Ethics Committee of Chengdu Anorectal Hospital.
Immunohistochemistry
Paraffin was used to embed the colon tissues of the mice, which were further sliced to a thickness of 5 µm for immunohistochemistry. After the completion of antigen repair, the slices were incubated with Anti-Mouse Serum Albumin antibody (ab19194, 1:500, Abcam, UK) against Ki67 (ab92742, 1:500, Abcam, UK) and N-cadherin (ab76057, 1:300, Abcam, UK) at 4 °C for 12 hours. Next, the secondary antibody, goat anti-rabbit IgG (ab150077, 1:1,000, Abcam, UK), was used and incubated for 30 min at 37 °C. Detection of proteins was carried out with the DAB kit (ab64264, Abcam, UK) according to the manufacturer’s instructions. Finally, Ki67 and N-cadherin distribution in colon cancer cells was observed under 400× amplification.
Statistical analysis
Assays were independently performed three times and data were shown as mean ± standard deviation (SD). Student’s t-test was applied to analyze differences between means, while Dunnett’s test was used to perform comparisons of parameters. Statistical significance was considered to exist when P<0.05.
Results
LSZ effected SW480 cells viability and LSZ toxicity detection
First, we detected the toxic effect of LSZ on SW480 cells and HCoEpiC cells using a CCK8 kit. As shown in the Figure 1A, the cells were treated with different concentrations of LSZ (0, 0.01, 0.025, 0.05, 0.1, 0.25, 0.5, 1, 1.5, 2, 2.5, 5, and 10 mM) for 24 hours. The data showed that LSZ significantly interfered with the viability of SW480 cells at a concentration greater than 1 mM, and LSZ was cytotoxic to all cells at concentration over 2 mM. Whereupon, SW480 cells underwent treatment with 3 different concentrations of LSZ (0.1, 0.5 and 1.5 mM) for 24, 48, and 72 h, respectively. As shown in Figure 1B, compared with the control, the proliferation of SW480 cells was significantly suppressed at 72 hours (P<0.05), suggesting that LSZ inhibited the growth of SW480 cells in a concentration-dependent manner, and the 3 concentrations of 0.1, 0.5 and 1.5 mM were selected to treat cells at 72 hours for the following experiment.
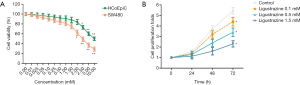
LSZ inhibited SW480 cell proliferation and induced apoptosis in colon cancer
Next, we determined SW480 cell apoptosis using flow cytometry, the results (Figure 2A) showed that the apoptosis ratio (19.2%, 24.5%, and 35.8%, respectively) of cells obviously increased after diverse concentrations of LSZ treatment compared with the control group (7.6%). Besides, colony formation analysis showed that the control cells had the potential to form more colonies, and the proliferation rate of SW480 cells was significantly decreased after LSZ treatment (Figure 2B). We also examined the level of the cell proliferation marker Ki67 by RT-qPCR and Western blot, and the results revealed that the different concentrations of LSZ decreased the expression of Ki67 at the levels of mRNA and protein, compared to the control group (Figure 2C,D). Simultaneously, we examined the cell apoptosis markers, as shown in Figure 2C. In comparison with the control group, the mRNA level of caspase-3 increased, while the expression of Bcl-2/Bax decreased after LSZ treatment. Interestingly, the protein levels of cleaved-Caspase-3 and Bcl-2/Bax were the same as those of mRNA at different concentrations (Figure 2D), suggesting LSZ inhibited the proliferation of SW480 cells and induced apoptosis in a concentration-dependent manner.
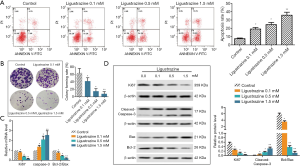
LSZ suppressed SW480 cell migration, invasion and EMT of SW480 cells
In this study, we tested the invasion ability of SW480 by Transwell assay. As Figure 3A sets out, the invasive capabilities of SW480 cells were remarkably decreased after different concentrations of LSZ treatment in comparison with the control group. In addition, wound healing assay showed that the migration activity of SW480 cells was prominently lower in the treated cells than in the control cells (Figure 3B). After different concentrations (0.1, 0.5, and 1.5 mM) of LSZ treatment, the cell morphology of SW480 cells gradually transformed to flat squamous morphology (Figure 3C). We therefore hypothesized that LSZ was likely involved in the EMT of CRC cells, which is a key event in the process of tumor invasion and metastasis. We found that the E-cadherin (epithelial marker) mRNA level was significantly increased while the mesenchymal markers (N-cadherin, Vimentin and α-SMA) were decreased with LSZ treatment (Figure 3D). In addition, LSZ treatment markedly increased the protein expression of E-cadherin and decreased the expression of N-cadherin, Vimentin, and α-SMA in a concentration-dependent manner (Figure 3E). Thus, these results suggested that LSZ suppressed the migration, invasion, and EMT of SW480 cells.
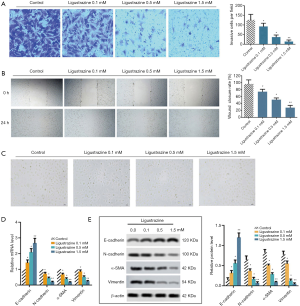
LSZ inhibited the SW480 proliferation and EMT via PI3K/AKT/mTOR pathway
To investigate the role of LSZ in the PI3K/AKT/mTOR pathway at 3 different concentrations (0.1, 0.5, and 1.5 mM) of LSZ treatment, we detected the protein expressions of PI3K, AKT, and mTOR by Western blotting. The results revealed that when compared to the control group, the phosphorylation levels of PI3K, AKT, and mTOR were significantly decreased in the cells treated with different concentrations of LSZ (Figure 4A). In addition, we added the activator 740 Y-P (concentration, 1.53 µM) of PI3K to further explore its mechanism, and SW480 cells were divided into four groups: control, 1.6 mM LSZ, 1.53 µM 740 Y-P, and LSZ (1.6 mM) +740 Y-P (1.53 µM). We then explored the SW480 proliferation by colony formation assay, and the results showed that LSZ inhibited colony formation and that more colonies were formed after the addition of 740 Y-P. Moreover, the number of colonies was significantly reduced by co-treatment of LSZ and 740 Y-P (Figure 4B). The Western blot assay revealed that expressions of Ki67, N-cadherin, Vimentin, and α-SMA were significantly decreased after LSZ treatment, while the expression of E-cadherin was increased. This process was reversed after 740 Y-P treatment. Notably, following co-treatment with 740 Y-P and LSZ, the expressions of Ki67, N-cadherin, Vimentin, and α-SMA decreased and the protein level of E-cadherin increased, compared to treatment with 740 Y-P alone (Figure 4C). Altogether, the data demonstrated that LSZ regulated cell proliferation and EMT by inactivating the PI3K/AKT/mTOR pathway in colon cancer.
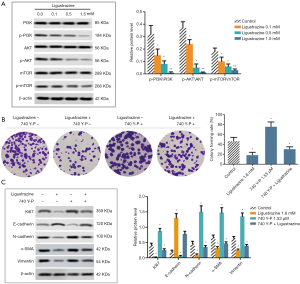
LSZ suppressed the growth of xenograft tumors in vivo
To confirm the anti-tumor effect of AS-IV in mice, we established a SW480 xenograft tumor model. The tumor weights of the LSZ-treated mice were found to be significantly lower than those of the corresponding control mice (Figure 5A). In addition, we also tested for Ki67 and N-cadherin expression in tumor tissues by immunohistochemistry. As shown in Figure 5B, the positive cell expressions of both Ki67 and N-cadherin were remarkably decreased in the LSZ-treated group when compared to the control group, suggesting LSZ exhibited anti-tumor activity in vivo.
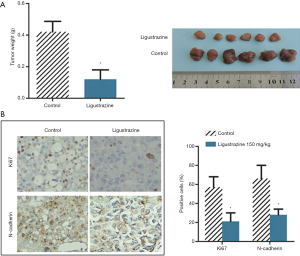
Discussion
At present, colon cancer is the third most likely to be diagnosed across the globe. The disease’s tendency to metastasize is behind the poor prognosis faced by colon cancer patients (16). So far, chemotherapy is the primary treatment for inhibiting colon cell proliferation and angiogenesis. However, the emergence of some anti-tumor drugs has provided more treatment options to be used in combination with chemotherapy including bevacizumab, cetuximab, and vemurafenib monoclonal antibodies, which block the activity of VEGF and Ras/Raf and inactivate the MAPK and PI3K/AKT pathways to improve survival in patients with metastatic colorectal cancer (17). In recent years, Chinese medicine has drawn continuous attention due to its positive effects in the treatment of various diseases. In this study, we demonstrated for the first time that LSZ induced apoptosis, inhibited cell growth, motility, and EMT via the PI3K/AKT/mTOR pathway, providing a new possibility for colon cancer treatment.
LSZ originates from a Chinese herb that has been proven to suppress tumor activity in a range of different cancers (18,19). Past researches have reported that LSZ stimulates anion secretion in the distal colon of rats (20,21). In addition, LSZ was found to inhibit the activity, migration, invasion and induced apoptosis of breast cancer cells by affecting AKT and caspase-3-related activity (22). Zou et al. found that novel LSZ-based synthetic piperlongumine analogue strongly inhibited proliferation and metastasis in colorectal cancer cells (23). Combined with the previous paper, we studied the effect of LSZ on colon cancer. The data showed that LSZ inhibited the viability and motility of SW480 cell lines and stimulated its apoptosis. In addition, we found that LSZ inhibited the growth of SW480 cells through downregulation of the mRNA levels of Ki67 and Bcl-2/Bax and upregulation of caspase-3, which was consistent with the previous results. Simultaneously, LSZ also slowed down cell metastasis by regulating the EMT process. EMT, a biological process, sees polarized epithelial cells subjected to multiple biochemical changes through their basal surface interacting with the basement membrane. enabling them to exhibit mesenchymal phenotypes, including enhanced migration and invasion (24). It is divided into three different subtypes, of which type 3 EMT is associated with cancer progression and metastasis (25). The main feature of EMT is the reduction of epithelial marker membrane (E-cadherin) and the increase of mesenchymal markers (N-cadherin and Vimentin) (26). Our study found that the cell morphology was altered in SW480 cells, and the E-cadherin (epithelial marker) mRNA level was significantly increased, while the levels of mesenchymal markers (N-cadherin, Vimentin, and α-SMA) were decreased, suggesting changes in cell morphology may be related to EMT, which mediates cell metastasis. In our animal experiments, LSZ reduced the weight of colon tumors, accompanied by downregulation of Ki67 and N-cadherin. Together, these results demonstrate that LSZ effectively hindered the progression of colon cancer and prevented further deterioration of the tumor.
In many different types of cancer, the PI3K/AKT/mTOR signal pathway is dysregulated and is considered to be a promising target for therapy (12). Attention has been increasingly drawn to the development of targeted therapies that inhibit this signal, as the PI3K/AKT/mTOR pathway regulates cell cycles, angiogenesis, invasion, apoptosis, and even drug resistance. Ma et al. reported that the activation of PI3K/AKT/mTOR signaling is associated with colon cancer metastasis (16). Wang et al. demonstrated that the PI3K/AKT signaling pathway was involved in the regulation of metastasis-associated colon cancer 1 (MACC1) in 5-fluorouracil resistant cells (27). In addition, Chen et al. found that the PI3K/Akt/mTOR pathway mediated colon cancer stem cells (CCSC) proliferation and survival attributed to increased cleaved-caspase-3 (28). Importantly, the RAS/PI3K/AKT/mTOR cascade carries a vital responsibility in ghrelin-induced colon cancer cell proliferation (29). In this study, we confirmed that LSZ significantly decreased the phosphorylation levels of PI3K, AKT, and mTOR. After adding the activator 740 Y-P of PI3K, we found that LSZ regulated SW480 cell proliferation and EMT-mediated cell movement by inhibiting the activity of the PI3K/AKT/mTOR pathway, supporting previous literature conclusions.
Conclusions
In conclusion, our study demonstrated the potential value of LSZ in slowing the progress of colon cancer, by inhibiting cell proliferation, migration, invasion and EMT processes and inducing apoptosis through the PI3K/AKT/mTOR pathway in vitro. It may prove to be a candidate drug for the treatment of colon cancer and even other malignancies. Although we found that LSZ inhibited tumor growth in mice, the mechanism of this action in vivo requires more in-depth research.
Acknowledgments
Funding: None.
Footnote
Conflicts of Interest: All authors have completed the ICMJE uniform disclosure form (available at http://dx.doi.org/10.21037/tcr-20-940). The authors have no conflicts of interest to declare.
Ethical Statement: The authors are accountable for all aspects of the work in ensuring that questions related to the accuracy or integrity of any part of the work are appropriately investigated and resolved.
Open Access Statement: This is an Open Access article distributed in accordance with the Creative Commons Attribution-NonCommercial-NoDerivs 4.0 International License (CC BY-NC-ND 4.0), which permits the non-commercial replication and distribution of the article with the strict proviso that no changes or edits are made and the original work is properly cited (including links to both the formal publication through the relevant DOI and the license). See: https://creativecommons.org/licenses/by-nc-nd/4.0/.
References
- Terzić J, Grivennikov S, Karin E, et al. Inflammation and colon cancer. Gastroenterology 2010;138:2101-14.e5. [Crossref] [PubMed]
- Bray F, Ferlay J, Soerjomataram I, et al. Global cancer statistics 2018: GLOBOCAN estimates of incidence and mortality worldwide for 36 cancers in 185 countries. CA Cancer J Clin 2018;68:394-424. [Crossref] [PubMed]
- Siegel RL, Miller KD, Jemal A. Cancer statistics, 2018. CA Cancer J Clin 2018;68:7-30. [Crossref] [PubMed]
- Bailey CE, Hu CY, You YN, et al. Increasing disparities in the age-related incidences of colon and rectal cancers in the United States, 1975-2010. JAMA Surg 2015;150:17-22. [Crossref] [PubMed]
- Chen J, Chen J, Wang X, et al. Ligustrazine alleviates acute pancreatitis by accelerating acinar cell apoptosis at early phase via the suppression of p38 and Erk MAPK pathways. Biomed Pharmacother 2016;82:1-7. [Crossref] [PubMed]
- Xiong L, Fang ZY, Tao XN, et al. Effect and mechanism of ligustrazine on Th1/Th2 cytokines in a rat asthma model. Am J Chin Med 2007;35:1011-20. [Crossref] [PubMed]
- Feng L, Xiong Y, Cheng F, et al. Effect of ligustrazine on ischemia-reperfusion injury in murine kidney. Transplant Proc 2004;36:1949-51. [Crossref] [PubMed]
- Feng L, Ke N, Cheng F, et al. The protective mechanism of ligustrazine against renal ischemia/reperfusion injury. J Surg Res 2011;166:298-305. [Crossref] [PubMed]
- Chen L, Lu Y, Wu JM, et al. Ligustrazine inhibits B16F10 melanoma metastasis and suppresses angiogenesis induced by Vascular Endothelial Growth Factor. Biochem Biophys Res Commun 2009;386:374-9. [Crossref] [PubMed]
- Pan J, Shang JF, Jiang GQ, et al. Ligustrazine induces apoptosis of breast cancer cells in vitro and in vivo. J Cancer Res Ther 2015;11:454-8. [Crossref] [PubMed]
- Han J, Song J, Li X, et al. Ligustrazine suppresses the growth of HRPC cells through the inhibition of cap- dependent translation via both the mTOR and the MEK/ERK pathways. Anticancer Agents Med Chem 2015;15:764-72. [Crossref] [PubMed]
- Aoki M, Fujishita T. Oncogenic roles of the PI3K/AKT/mTOR axis. Curr Top Microbiol Immunol 2017;407:153-89. [Crossref] [PubMed]
- Hers I, Vincent EE, Tavaré JM. Akt signalling in health and disease. Cell Signal 2011;23:1515-27. [Crossref] [PubMed]
- Lian G, Chen S, Ouyang M, et al. Colon cancer cell secretes EGF to promote M2 polarization of TAM through EGFR/PI3K/AKT/mTOR pathway. Technol Cancer Res Treat 2019;18:1533033819849068. [Crossref] [PubMed]
- Cicenas J, Tamosaitis L, Kvederaviciute K, et al. KRAS, NRAS and BRAF mutations in colorectal cancer and melanoma. Med Oncol 2017;34:26. [Crossref] [PubMed]
- Ma JC, Sun XW, Su H, et al. Fibroblast-derived CXCL12/SDF-1α promotes CXCL6 secretion and co-operatively enhances metastatic potential through the PI3K/Akt/mTOR pathway in colon cancer. World J Gastroenterol 2017;23:5167-78. [Crossref] [PubMed]
- Price T, Shen L, Ma B, et al. Phase II APEC trial: The impact of primary tumor side on outcomes of first-line cetuximab plus FOLFOX or FOLFIRI in patients with RAS wild-type metastatic colorectal cancer. Asia Pac J Clin Oncol 2019;15:225-30. [PubMed]
- Ai Y, Zhu B, Ren C, et al. Discovery of new monocarbonyl ligustrazine-curcumin hybrids for intervention of drug-sensitive and drug-resistant lung cancer. J Med Chem 2016;59:1747-60. [Crossref] [PubMed]
- Song YN, Guo XL, Zheng BB, et al. Ligustrazine derivate DLJ14 reduces multidrug resistance of K562/A02 cells by modulating GSTπ activity. Toxicol In Vitro 2011;25:937-43. [Crossref] [PubMed]
- Xing Y, He Q, Zhu JX, et al. Basolateral membrane mechanisms involved in ligustrazine-stimulated anion secretion in rat distal colon. Sheng Li Xue Bao 2003;55:653-7. [PubMed]
- Zhao WC, Duan DX, Wang ZJ, et al. The underlying cellular mechanism in the effect of tetramethylpyrazine on the anion secretion of colonic mucosa. Jpn J Physiol 2005;55:325-9. [Crossref] [PubMed]
- Shen J, Zeng L, Pan L, et al. Tetramethylpyrazine regulates breast cancer cell viability, migration, invasion and apoptosis by affecting the activity of Akt and caspase-3. Oncol Lett 2018;15:4557-63. [PubMed]
- Zou Y, Zhao D, Yan C, et al. Novel ligustrazine-based analogs of piperlongumine potently suppress proliferation and metastasis of colorectal cancer cells in vitro and in vivo. J Med Chem 2018;61:1821-2. [Crossref] [PubMed]
- Kalluri R, Neilson EG. Epithelial-mesenchymal transition and its implications for fibrosis. J Clin Invest 2003;112:1776-84. [Crossref] [PubMed]
- Kalluri R, Weinberg RA. The basics of epithelial-mesenchymal transition. J Clin Invest 2009;119:1420-8. [Crossref] [PubMed]
- Bae SY, Kim HJ, Lee KJ, et al. Translationally controlled tumor protein induces epithelial to mesenchymal transition and promotes cell migration, invasion and metastasis. Sci Rep 2015;5:8061. [Crossref] [PubMed]
- Wang J, Wang W, Cai H, et al. MACC1 facilitates chemoresistance and cancer stem cell-like properties of colon cancer cells through the PI3K/AKT signaling pathway. Mol Med Rep 2017;16:8747-54. [Crossref] [PubMed]
- Chen S, Fisher RC, Signs S, et al. Inhibition of PI3K/Akt/mTOR signaling in PI3KR2-overexpressing colon cancer stem cells reduces tumor growth due to apoptosis. Oncotarget 2016;8:50476-88. [Crossref] [PubMed]
- Lien GS, Lin CH, Yang YL, et al. Ghrelin induces colon cancer cell proliferation through the GHS-R, Ras, PI3K, Akt, and mTOR signaling pathways. Eur J Pharmacol 2016;776:124-31. [Crossref] [PubMed]