Different primers for diagnosing circulating cell-free DNA of colorectal cancer
Introduction
Colorectal cancer (CRC) is currently the fourth most common cancer (1). Five-year survival for stages I and II is 90%, which drops to 14% for stage IV disease (2). Therefore, early detection and treatment are significant for the management of CRC. Many diagnostic methods have been used to detect cancer, such as protein tumor markers, imaging techniques, and tissue biopsy (3). Serum protein biomarkers such as carcinoembryonic antigen (CEA) and carbohydrate antigen 19-9 (CA19-9) have limited sensitivity and specificity (4,5). Imaging techniques are challenged by small lesions and may be influenced by structures. Tissue biopsy, a gold standard for diagnosing cancer, has disadvantages from invasion and medical complications.
Liquid biopsy has advantages such as easy accessibility, minimal invasiveness, and repeatability (6). Cell-free DNA (cfDNA), one of the markers of liquid biopsies, has been applied in all phases of cancer patient management, including predictive and early detection, monitoring disease progression, and survival recurrence and minimal residual disease (7). The cfDNA is released by viable and necrosis cancer cells and may reflect the entire tumor genome. Molecular characteristics of cfDNA have been extensively studied, including concentration, genomic alterations, copy number mutations, methylation changes, single-nucleotide mutations, cancer-derived viral sequences, and microsatellite alterations (8,9).
The concentration of cfDNA has been identified as a potential prognostic biomarkers in CRC. The concentration of cfDNA based on different primers has been found to be significantly higher in CRC than in healthy volunteers and intestinal polyp patients (10-13). However, different primers in CRC have not yet been compared. We presented a comparison study in plasma samples to monitor the variation and efficiency of cfDNA primers.
Methods
Sample collection
Seventy-one patients with CRC and 20 patients with proliferative intestinal polyps at Yongchuan Hospital of Chongqing Medical University from May 2018 to February 2019 were enrolled. The CRC group consisted of 45 non-metastatic colorectal cancer (non-mCRC) patients, 13 patients with metastatic colorectal cancer (mCRC), and 13 patients with recurrent colorectal cancer (rCRC). The diagnosis of all patients was confirmed by biopsy, computed tomography (CT), magnetic resonance imaging (MRI), and positron emission tomography (PET) scans. All peripheral venous blood samples were collected in EDTA-K2-coated tubes and separated by centrifugation at 1,600 g for 10 min at 4 °C (Neofuge 15R, Heal Force). The supernatant was transferred to a new tube and centrifuged again at 16,000 g for 10 min at 4 °C (Neofuge 13R, Heal Force). Purified plasma was carefully removed without disturbing the lower residual layer and stored at −80 °C for further analyses (14). Plasma cfDNA was extracted from 400 µL blood plasma using the plasma cfDNA extraction kit (GO-MXCF-100, Changchun China, http://www.geneonbio.com/) according to the manufacturer’s protocol. The total eluted cfDNA (~40 µL) was transferred into 0.2 mL Eppendorf tubes and then stored at −20 °C.
Quatitative polymerase chain reaction (q-PCR)
Three primer sequences were used to compare the efficiency of different genes: ALU elements (ALU), Beta-actin (ACTB), glyceraldehyde-3-phosphate dehydrogenase (GAPDH) were previously published and used to quantify the level of cfDNA. Three fragments of 108 bp of the GAPDH gene, 115 bp of the ALU gene, and 100 bp of the ACTB gene were amplified with the following primers to quantify the yield of cfDNA. The ALU primer forward: 5'-CCTGAGGTCAGGAGTTCGAG-3' and reverse: 5'-CCCGAGTAGCTGGGATTACA-3' (11); The ACTB primer forward: 5'-GCACCACACCTTCTACAATGA-3' and reverse: 5'-GTCATCTTCTCGCGGTTGGC-3' (15). The GAPDH primer forward: 5'-ATGTTCGTCATGGGTGTGAA-3' and reverse: 5'-GGTGCTAAGCAGTTGGTGGT-3' (13). The total q-PCR reaction volume of 25 µL contained 0.4 µM of forward and reversed primers, 12.5 µL of TB Green®Premix Ex Taq™ II (Tli RNaseH Plus) (Takara, Japan), 8.5 µL double-distilled water and 2 µL of cfDNA. The subsequent progress involved incubation at 95 °C for 30 s, 39 cycles of denaturation at 95 °C for 5 s, annealing at 55 °C for 30 s, extension at 72 °C for 30 s, and ended with a melt curve from 65 to 95 °C for 5 s in Real-time Quantitative PCR Detecting System (Bio-Rad CFX96, USA). The absolute equivalent amount of cfDNA in each sample was determined using a standard curve with serial dilutions (from 9.813 to 98,130 ng/mL) of human genomic DNA. Standard curves were created for ALU, ACTB, and GAPDH primer sets by PCR amplifying 10-fold serially diluted DNA samples. The concentration of cfDNA for different primers was represented by the concentration of ALU, ACTB, and GAPDH, respectively. The results were presented as the median and interquartile range.
CEA analysis
Venous blood (5 mL) was collected from the patients simultaneously. The concentrations of CEA were measured at the Clinic Pathology Laboratory of Yongchuan Hospital affiliated to Chongqing Medical University by Elecsys. COBAS (e601 Germany) was adopted to calculate their quantity.
Receiver-operating characteristic (ROC) curve analysis
The ROC curve was used to assess the diagnostic value of ALU, ACTB, GAPDH, CEA and the combination diagnostic for non-mCRC.
Statistical analysis
The Mann-Whiney U-test and Kruskal-Wallis H-test were used to compare the ALU, ACTB, and GAPDH between the groups. Statistical analyses were performed using SPSS 22.0 software (SPSS Inc., Chicago, IL, USA). The results were considered statistically significant at P<0.05. The corresponding figures were drawn in GraphPad Prism v 8.01 software (Graphpad Software Inc.).
Results
Patient characteristics
A total of 91 individuals were enrolled. The data obtained from plasma samples of 71 CRC patients (male/female: 35/36) were compared to 20 patients with intestinal polyps (male/female: 11/9) as controls. For controls and CRC patients, the median of age was 55.00 (range, 44.75–64.00) and 66.00 (range, 61.00–71.00) years, respectively. The patients did not differ statistically by age and gender, as verified by independent χ2 and t-tests (P>0.05 for both). The abnormal value was excluded in further analysis.
The cfDNA concentration in intestinal polyp and CRC patients
The concentration of ALU and GAPDH in the CRC group was significantly higher than in the intestinal polyp group (P=0.003 and P=0.000, respectively). However, there was no difference in ACTB (P=0.806) (Table 1; Figure 1A,B,C). Compared with the control group, the concentration of ALU and GAPDH in non-mCRC patients and mCRC patients was significantly higher (P<0.05), while there was no significant difference in the concentration of ACTB. The rCRC group also had higher concentration than the control group, expect for ACTB. Compared with the non-mCRC and mCRC groups, the concentration of the rCRC group was decreased, although there was no statistical difference (Table 2; Figure 1D,E,F).
Table 1
Groups | Intestinal polyp | CRC | Z | P |
---|---|---|---|---|
ALU | −2.983 | 0.003 | ||
n | 18 | 65 | ||
C (ng/mL) | 13.03 (0.01, 120.43) | 97.51 (20.48, 412.70) | ||
ACTB | −0.246 | 0.806 | ||
n | 12 | 67 | ||
C (ng/mL) | 364.49 (143.97, 2,749.28) | 484.33 (162.79, 2,390.97) | ||
GAPDH | −3.757 | 0.000 | ||
n | 20 | 71 | ||
C (ng/mL) | 79.54 (27.83, 115.15) | 168.63 (113.68, 270.76) |
cfDNA, cell-free DNA; CRC, colorectal cancer.
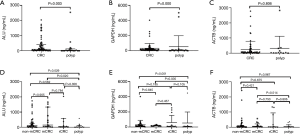
Table 2
Groups | Non-mCRC | mCRC | rCRC | Intestinal polyp | F | P |
---|---|---|---|---|---|---|
ALU | 11.084 | 0.011 | ||||
n | 41 | 13 | 11 | 18 | ||
C (ng/mL) | 124.57 (21.92, 341.42)# | 143.84 (39.04, 1,048.16)# | 47.23 (6.42, 369.34) | 13.03 (0.01, 120.43) | ||
ACTB | 0.769 | 0.857 | ||||
n | 43 | 13 | 11 | 12 | ||
C (ng/mL) | 486.34 (134.86, 1,761.76) | 593.29 (331.68, 2,152.72) | 351.97 (112.49, 13,097.78) | 364.49 (143.97, 2,749.28) | ||
GAPDH | 15.832 | 0.001 | ||||
n | 45 | 13 | 13 | 20 | ||
C (ng/mL) | 171.44 (123.34, 287.50)# | 194.78 (113.43, 235.29)# | 117.68 (72.74, 1,102.51) | 79.54 (27.83, 115.15) |
#, compared with intestinal polyp, there were statistical differences (P<0.05). cfDNA, cell-free DNA; CRC, colorectal cancer; mCRC, metastatic colorectal cancer; rCRC, recurrent colorectal cancer.
ROC curve analysis between the non-mCRC and intestinal polyp groups
The area under curves (AUCs) of ALU, GAPDH, and ACTB were 0.734, 0.800, and 0.503, respectively. The AUC of ALU, GAPDH, and CEA combined was 0.974 (Table 3; Figure 2A,B).
Table 3
Primers | AUC | Cut-off | Sensitivity (%) | Specificity (%) | 95% CI | P value | PPV (%) | NPV (%) |
---|---|---|---|---|---|---|---|---|
ALU | 0.734 | 0.17 | 100 | 44 | 0.579–0.889 | 0.004 | 80.39 | 100 |
GAPDH | 0.800 | 122.09 | 77.8 | 85 | 0.660–0.939 | <0.001 | 92.1 | 62.96 |
ACTB | 0.503 | 451.79 | 51.2 | 66.7 | 0.315–0.692 | 0.967 | 84.61 | 27.58 |
CEA | 0.843 | 3.19 | 73.3 | 86.7 | 0.727–0.959 | <0.001 | 94.28 | 52 |
CEA + GAPDH | 0.834 | 0.69 | 71.1 | 86.7 | 0.718–0.951 | <0.001 | 94.12 | 50 |
CEA + ACTB | 0.848 | 0.72 | 74.4 | 90.9 | 0.711–0.985 | <0.001 | 96.96 | 47.62 |
CEA + ALU | 0.882 | 0.76 | 73.2 | 92.3 | 0.789–0.974 | <0.001 | 96.77 | 52.17 |
ALU + GAPDH + CEA | 0.974 | 0.63 | 92.7 | 100 | 0.973–1.000 | <0.001 | 100 | 81.25 |
CEA, carcinoembryonic antigen.
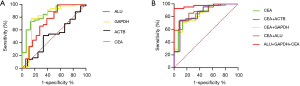
Clinical variables of CRC patients
The analysis showed that the concentration of ALU was correlated with size (P=0.004), histological differentiation (P=0.001), and lymphatic metastasis (P=0.004). The concentration of ACTB was correlated with histological differentiation (P=0.018), local invasion (P=0.003), and lymphatic metastasis (P=0.011). The concentration of GAPDH was correlated with histological differentiation (P=0.017) (Table 4).
Table 4
Clinical variables | ALU | ACTB | GAPDH | ||||||||
---|---|---|---|---|---|---|---|---|---|---|---|
n | C (ng/mL) | P | n | C (ng/mL) | P | n | C (ng/mL) | P | |||
Age (years) | 0.334 | 0.647 | 0.215 | ||||||||
≥60 | 50 | 111.50 (22.57, 463.21) | 55 | 593.29 (149.98, 2,573.03) | 56 | 175.62 (115.12, 276.54) | |||||
<60 | 15 | 50.78 (10.90, 287.92) | 12 | 355.24 (170.89, 845.99) | 15 | 147.97 (89.14, 222.16) | |||||
Gender | 0.401 | 0.164 | 0.872 | ||||||||
Male | 33 | 143.84 (19.40, 532.62) | 32 | 790.05 (214.93, 3,277.28) | 35 | 165.56 (113.71, 270.76) | |||||
Female | 32 | 54.75 (21.27, 312.84) | 35 | 364.77 (134.86, 860.94) | 36 | 170.03 (112.60, 262.89) | |||||
Location | 0.955 | 0.682 | 0.296 | ||||||||
Rectum | 44 | 97.11 (21.27, 347.12) | 44 | 422.55 (153.19, 2,233.67) | 47 | 160.23 (113.19, 235.87) | |||||
Colon | 21 | 98.44 (19.28, 514.86) | 23 | 677.44 (195.18, 3,105.92) | 24 | 194.58 (115.12, 447.09) | |||||
Tumor sizes (cm) | 0.004 | 0.455 | 0.643 | ||||||||
≥4 | 37 | 166.64 (49.07, 522.05) | 38 | 744.93 (184.56, 2,675.31) | 40 | 182.30 (122.94, 275.15) | |||||
<4 | 28 | 30.59 (10.27, 235.21) | 29 | 304.84 (156.39, 2,020.54) | 31 | 151.21 (113.19, 268.83) | |||||
Histologic differentiation | 0.001 | 0.018 | 0.017 | ||||||||
Low | 17 | 456.06 (113.49, 1,113.15) | 19 | 1,058.66 (769.23, 4,606.17) | 20 | 209.79 (172.21, 447.09) | |||||
Middle | 37 | 97.50 (22.88, 341.42) | 38 | 354.88 (121.19, 1,678.02) | 40 | 154.44 (113.85, 276.54) | |||||
High | 11 | 20.33 (6.27, 41.64) | 10 | 238.36 (140.61, 360.27) | 11 | 113.19 (87.00, 160.23) | |||||
Local invasion | 0.749 | 0.003 | 0.711 | ||||||||
Muscularis propria | 10 | 48.85 (14.07, 401.77) | 10 | 235.17 (8.20, 1,880.84) | 11 | 268.83 (108.37, 328.22) | |||||
Subserosa | 14 | 121.57 (22.57, 796.18) | 13 | 805.77 (218.45, 1,542.53) | 14 | 165.83 (106.99, 272.22) | |||||
Visceral peritoneum | 41 | 98.44 (22.89, 392.74) | 44 | 441.76 (172.38, 3,240.72) | 46 | 167.09 (113.56, 238.90) | |||||
Lymphatic metastasis | 0.004 | 0.011 | 0.486 | ||||||||
0 | 33 | 29.05 (10.36, 341.42) | 32 | 331.81 (156.86, 1,066.39) | 35 | 157.67 (108.37, 276.32) | |||||
≤3 | 24 | 134.20 (41.89, 327.35) | 27 | 486.33 (162.79, 3,334.40) | 28 | 175.62 (113.69, 265.18) | |||||
≥4 | 8 | 949.39 (146.90, 1,621.20) | 8 | 1,893.96 (284.70, 7,585.63) | 8 | 218.80 (144.06, 306.58) | |||||
Distant metastasis | 0.738 | 0.364 | 0.559 | ||||||||
Yes | 39 | 97.50 (20.62, 352.81) | 40 | 441.76 (138.64, 1,587.98) | 42 | 170.03 (123.61, 276.39) | |||||
No | 26 | 97.12 (19.66, 483.58) | 27 | 593.29 (188.60, 3,334.40) | 29 | 165.56 (108.27, 261.53) | |||||
Tumor stage (TNM) | 0.971 | 0.524 | 0.807 | ||||||||
I | 7 | 50.86 (18.47, 499.07) | 8 | 235.17 (41.06, 2,096.51) | 8 | 216.99 (112.51, 321.76) | |||||
II | 17 | 47.36 (19.43, 514.86) | 17 | 774.32 (249.34, 2,076.36) | 18 | 170.03 (120.67, 282.06) | |||||
III | 15 | 130.27 (25.44, 201.93) | 15 | 357.79 (121.50, 1,058.66) | 16 | 161.25 (124.70, 213.93) | |||||
IV | 26 | 97.12 (19.66, 483.58) | 27 | 593.29 (188.60, 3,334.40) | 29 | 165.56 (108.27, 261.53) |
Italic P values are statistic value in clinical variable (P<0.05).
Discussion
CfDNA consists of three constituents: circulating tumor DNA (ctDNA), non-malignant tumor cell-derived DNA, and normal cell germline DNA (16). The DNA half-life is short in the circulation, ranging from 15 min to several hours, which can present the real-time status of a tumor (17,18). It has been estimated that from a tumor weighing 100 g, corresponding to 3×1010 tumor cells, up to 3.3% of tumor DNA may enter the blood every day (19). CtDNA holds a lot of information, but the information is highly fragmented, of a low amount, and is costly to extract, which limits its usage (20,21). However, quantification of cfDNA by qPCR is simple, inexpensive, and reproducible (22). The concentration of cfDNA in plasma has been widely studied in patients with various neoplasms, including hepatocellular carcinoma (HCC), breast cancer, renal cell carcinoma (RCC), gastric cancer, and CRC (10-13,23-27). The concentration of ALU and GAPDH in CRC was found to be significantly higher than in standard control groups (10-13). A higher concentration of ACTB has been observed in HCC and RCC than in healthy groups (23,26). To date, many genes have been reported for diagnosing cfDNA but are still technically challenging.
In our study, three genes served as the models to facilitate the comparison of the yields of cfDNA by q-PCR. The cfDNA concentration of ALU and GAPDH in the CRC group was higher than in the intestinal polyp group, and there was no significant difference in ACTB. Meanwhile, the yields of cfDNA with ALU and GAPDH were higher in malignancy. The results implied that ALU and GAPDH would be more suitable for detecting cfDNA.
The diagnostic value of the concentration of cfDNA in ALU, ACTB, and GAPDH was compared between the non-mCRC and intestinal polyp groups. The GAPDH gene showed the best diagnostic value (AUC =0.800) compared with ALU (AUC =0.734) and ACTB (AUC =0.503). The AUC for distinguishing non-mCRC from normal controls by ALU has been reported as 0.85 (11). In comparing HCC and chronic hepatitis B virus (HBV)-infected groups, the AUC value of ACTB was found to be 0.56 (23). Consequently, the concentration of cfDNA in GAPDH and ALU is superior to ACTB in distinguishing between non-mCRC and intestinal polyp groups. The diagnostic value of ALU and GAPDH could be improved significantly by combining them with CEA. The concentration of cfDNA was correlated with clinical variables, as reported (11,13). The results showed that the yield of cfDNA was strictly related to tumor size, histologic differentiation, local invasion, and lymphatic metastasis.
Conclusions
In conclusion, the yields of cfDNA from the GAPDH and ALU genes are expected to be sensitive indicators for the diagnosis of CRC. The GAPDH and ALU genes are superior to ACTB. The diagnostic value of ALU and GAPDH could be significantly improved by combining them with CEA.
Acknowledgments
Thanks for Eric Xia (Mariana University College of Osteopathic Medicine) and Lang Cha revised the grammar in this article.
Funding: This work was supported by
Footnote
Conflicts of Interest: All authors have completed the ICMJE uniform disclosure form (available at http://dx.doi.org/10.21037/tcr-19-2017). All authors report grants from Science and Health Joint Medical Research Project (2019QNXM013), grants from Natural Science Foundation of Yongchuan Hospital (Ycstc, 2014nc5010; Ycstc, 2019nb0202), grants from General Foundation of Yongchuan Hospital of Chongqing Medical University (YJJC201904), grants from Innovation Fund for Postgraduates of Yongchuan Hospital of Chongqing Medical University (YJSCX201808), during the conduct of the study.
Ethical Statement: The authors are accountable for all aspects of the work in ensuring that questions related to the accuracy or integrity of any part of the work are appropriately investigated and resolved. This research was approved by the Human Research Ethics Committee of Yongchuan Hospital Affiliated to Chongqing Medical University (approval ID: 20180401).
Open Access Statement: This is an Open Access article distributed in accordance with the Creative Commons Attribution-NonCommercial-NoDerivs 4.0 International License (CC BY-NC-ND 4.0), which permits the non-commercial replication and distribution of the article with the strict proviso that no changes or edits are made and the original work is properly cited (including links to both the formal publication through the relevant DOI and the license). See: https://creativecommons.org/licenses/by-nc-nd/4.0/.
References
- Luo K, Zhang Y, Xv C, et al. Fusobacterium nucleatum, the communication with colorectal cancer. Biomed Pharmacother 2019;116:108988. [Crossref] [PubMed]
- Siegel RL, Miller KD, Jemal A. Cancer statistics, 2019. CA Cancer J Clin 2019;69:7-34. [Crossref] [PubMed]
- Cheng J, Tang Q, Cao X, et al. Cell-Free Circulating DNA Integrity Based on Peripheral Blood as a Biomarker for Diagnosis of Cancer: A Systematic Review. Cancer Epidemiol Biomarkers Prev 2017;26:1595-602. [Crossref] [PubMed]
- Nikolaou S, Qiu S, Fiorentino F, et al. Systematic review of blood diagnostic markers in colorectal cancer. Tech Coloproctol 2018;22:481-98. [Crossref] [PubMed]
- Ning S, Wei W, Li J, et al. Clinical significance and diagnostic capacity of serum TK1, CEA, CA 19-9 and CA 72-4 levels in gastric and colorectal cancer patients. J Cancer 2018;9:494-501. [Crossref] [PubMed]
- Desmond BJ, Dennett ER, Danielson KM. Circulating Extracellular Vesicle MicroRNA as Diagnostic Biomarkers in Early Colorectal Cancer—A Review. Cancers 2019;12:1-16. [Crossref] [PubMed]
- Otandault A, Anker P, Al Amir Dache Z, et al. Recent advances in Circulating Nucleic Acids in oncology. Ann Oncol 2019;30:374-84. [Crossref] [PubMed]
- Bardelli A, Pantel K. Liquid Biopsies, What We Do Not Know (Yet). Cancer Cell 2017;31:172-9. [Crossref] [PubMed]
- Schwarzenbach H, Hoon DS, Pantel K. Cell-free nucleic acids as biomarkers in cancer patients. Nat Rev Cancer 2011;11:426-37. [Crossref] [PubMed]
- Alhanafy A, El Shafei M, Safan M, et al. Circulating cell free DNA as a biomarker in the serum of colorectal cancer patients. Ann Oncol 2017;28:vii31-Vii2. [Crossref]
- Hao TB, Shi W, Shen XJ, et al. Circulating cell-free DNA in serum as a biomarker for diagnosis and prognostic prediction of colorectal cancer. British Journal of Cancer 2014;111:1482-9. [Crossref] [PubMed]
- Bhangu JS, Taghizadeh H, Braunschmid T, et al. Circulating cell-free DNA in plasma of colorectal cancer patients - A potential biomarker for tumor burden. Surg Oncol 2017;26:395-401. [Crossref] [PubMed]
- Agah S, Akbari A, Talebi A, et al. Quantification of Plasma Cell-Free Circulating DNA at Different Stages of Colorectal Cancer. Cancer Invest 2017;35:625-32. [Crossref] [PubMed]
- Wang X, Wang L, Su Y, et al. Plasma cell-free DNA quantification is highly correlated to tumor burden in children with neuroblastoma. Cancer Med 2018;7:3022-30. [Crossref] [PubMed]
- Szpechcinski A, Rudzinski P, Kupis W, et al. Plasma cell-free DNA levels and integrity in patients with chest radiological findings: NSCLC versus benign lung nodules. Cancer Lett 2016;374:202-7. [Crossref] [PubMed]
- Thierry AR. A Step Closer to Cancer Screening by Blood Test. Clin Chem 2018;64:1420-2. [Crossref] [PubMed]
- Heidary M, Auer M, Ulz P, et al. The dynamic range of circulating tumor DNA in metastatic breast cancer. Breast Cancer Res 2014;16:421-30. [Crossref] [PubMed]
- Diehl F, Schmidt K, Choti MA, et al. Circulating mutant DNA to assess tumor dynamics. Nat Med 2008;14:985-90. [Crossref] [PubMed]
- Diehl F, Li M, Dressman D, et al. Detection and quantification of mutations in the plasma of patients with colorectal tumors. Proc Natl Acad Sci U S A 2005;102:16368-73. [Crossref] [PubMed]
- Diaz LA Jr, Bardelli A. Liquid biopsies: genotyping circulating tumor DNA. J Clin Oncol 2014;32:579-86. [Crossref] [PubMed]
- Siravegna G, Mussolin B, Venesio T, et al. How liquid biopsies can change clinical practice in oncology. Ann Oncol 2019;30:1580-90. [Crossref] [PubMed]
- Su Y, Wang L, Jiang C, et al. Increased plasma concentration of cell-free DNA precedes disease recurrence in children with high-risk neuroblastoma. BMC Cancer 2020;20:102-9. [Crossref] [PubMed]
- Chen H, Sun LY, Zheng HQ, et al. Total serum DNA and DNA integrity: diagnostic value in patients with hepatitis B virus-related hepatocellular carcinoma. Pathology 2012;44:318-24. [Crossref]
- Qu C, Wang Y, Wang P, et al. Detection of early-stage hepatocellular carcinoma in asymptomatic HBsAg-seropositive individuals by liquid biopsy. Proc Natl Acad Sci U S A 2019;116:6308-12. [Crossref] [PubMed]
- Cheng J, Holland-Letz T, Wallwiener M, et al. Circulating free DNA integrity and concentration as independent prognostic markers in metastatic breast cancer. Breast Cancer Res Treat 2018;169:69-82. [Crossref] [PubMed]
- Yamamoto Y, Uemura M, Nakano K, et al. Increased level and fragmentation of plasma circulating cell-free DNA are diagnostic and prognostic markers for renal cell carcinoma. Oncotarget 2018;9:20467-75. [Crossref] [PubMed]
- Normando SRC, Delgado PO, Rodrigues AKSB, et al. Circulating free plasma tumor DNA in patients with advanced gastric cancer receiving systemic chemotherapy. BMC Clin Pathol 2018;18:12. [Crossref] [PubMed]