Pathogenesis of serous, extra-uterine Müllerian epithelial cancer and therapeutic implications
Müllerian epithelial cancers include all epithelial cancers traditionally referred to as ovarian carcinomas in addition to those from organs derived from the Müllerian ducts such as uterus and cervix. They represent a heterogeneous group with variable biological and clinical-pathological characteristics in spite of their common Müllerian features, as these cancers include several histological subtypes and originate from different anatomic sites. This chapter focuses primarily on serous tumors of the fallopian tube fimbriae and of intra- and extra-ovarian endosalpingiosis, which have been traditionally referred to as either serous ovarian, tubal, or primary peritoneal carcinomas.
The alleged Müllerian origin of tumors that were once categorized as ovarian has led some authors to regard all serous tumors of the upper reproductive tract as a single entity, regardless of whether they originate from inside (e.g., endometrium) or outside (e.g., fimbriae) the uterus. However, the clinical management of intra-uterine tumors depends on several parameters that do not apply to extra-uterine tumors, such as lymphovascular invasion, depth of myometrial invasion, and others, underscoring the merit of distinguishing intra- from extra-uterine tumors. The term serous extra-uterine Müllerian epithelial tumors (“EUMET”), which was proposed earlier (1), will be used in this chapter to reflect modern concepts about their origin and histogenesis, and to emphasize this distinction.
Although the exact molecular mechanisms intrinsically associated with the development of serous extra-uterine Müllerian tumors are still unclear, their well-established risk factors and molecular characteristics, as well as observations with in vitro models and experimental animals, can provide important clues. Current knowledge in these areas will first be reviewed, followed by an attempt to synthesize this information into a working model of cancer development.
Insights from non-genetic risk factors
Most extra-uterine Müllerian tumors occur sporadically, without evidence of genetic predisposition. Knowledge of environmental factors associated with predisposition to any cancer type can provide important insights into the mechanisms underlying its development. This is particularly true of EUMET where strong predisposing factors have been identified.
Reproductive factors
An active ovulatory cycle is the best established risk factor for the sporadic form not only of the serous subtype of EUMET, but of all subtypes except mucinous (2,3). Interruption of ovulatory activity protects against the development of this disease independently of whether such interruption is achieved through pregnancy or oral contraceptives, although there is evidence of a first pregnancy being more protective than subsequent ones. For example, use of oral contraceptives for 5 years results in an approximately 40% decrease in lifetime extra-uterine Müllerian cancer risk, which is similar to the protective effect of five pregnancies after the first (4).
Fathalla (5) sought to explain the association between ovulatory activity and cancer risk several decades ago by proposing that chronic breakage and repair of the ovarian coelomic epithelium (the epithelial cell layer that lines the ovarian surface) resulting from monthly releases of the egg might predispose this epithelium to malignant transformation (the incessant ovulation hypothesis). This hypothesis seemed attractive given the known association between cancer predisposition and cellular proliferation, one of the consequences of chronic repair. It was also well in line with the prevailing idea, at the time it was first formulated, that serous extra-uterine Müllerian tumors originated in the ovarian coelomic epithelium. However, not only is it not supported by current concepts about the cell of origin of these tumors (1,6), but also it fails to account for all epidemiological data. For example, the disproportionately increased protective effect of later pregnancies compared to early ones, as well as the progressive rise in extra-uterine Müllerian cancer incidence after menopause, cannot be readily accounted for by the incessant ovulation hypothesis (2,3). There was a 51% reduction in risk of developing these cancers in women who had given birth after the age of 35 compared to nulliparous women in a population based case-control study involving 477 patients with EUMET and 660 controls. Although prior births further reduced the risk, the magnitude of the protective effect of an early pregnancy was less than that of a pregnancy occurring after age 35 (2).
Although the incessant ovulation hypothesis is still widely quoted, most authors currently favor the view that it is the hormonal changes associated with the normal menstrual cycle that may have a lasting effect on predisposition to neoplastic transformation. Estradiol, which is unopposed during the first half (follicular phase) of the menstrual cycle, stimulates growth of benign and malignant EUMET cells in vitro, while progesterone, which is elevated during the second half (luteal phase) of the cycle, inhibits the growth of the same cells (7). The fact that pituitary gonadotropins, which have high circulating levels around menopause, stimulate the growth of EUMET in vitro suggests that hormonal changes associated with menopause may also play a role (7). Also supporting a role for the hormonal changes associated with menstrual cycle progression as risk factors for EUMET development is evidence that exogenous administration of such hormones, for example the use of hormone replacement therapy to alleviate the symptoms of menopause, is a significant risk factor (8). A study examining the long-term effects of oral contraceptives in macaques suggested that the direct action of progestins is primarily responsible for the protective effects of oral contraceptives (9).
Different authors have also attempted to account for the association between ovulatory activity and cancer risk based on additional mechanisms leading to hormonal changes in individuals with uninterrupted normal menstrual cycles. For example, the “stromal hyperactivity hypothesis” stipulates that although most ovarian follicular cells undergo apoptosis following release of the egg and the ensuing luteinization period, some may persist and retain their hormone-producing ability (10). This would result in an accumulation of steroid producing cells proportionate to the number of lifetime ovulations. Indeed, the basal levels of circulating estradiol were higher in premenopausal women with a greater lifetime number of ovulatory cycles in a longitudinal study (10). A role for androstenedione, the major ovarian hormone in post-menopausal women that is also suppressed by oral contraceptives, has been suggested by Risch (11) and further supported by the fact that circulating levels of this hormone are higher in the serum of patients with extra-uterine Müllerian cancer compared to matched controls (12).
Inflammatory factors
Although reproductive factors associated with the menstrual cycle are by far the strongest risk determinants of EUMET, a role for inflammation has also been suggested (13). Application of talc, an irritant, on the perineal area has consistently been associated with a small to moderate increase in risk of the serous subtype as well as of mucinous and clear cell subtypes (14). Inflammatory conditions such as pelvic inflammatory disease (PID) have also been associated with increased risk (15-20). The association between endometriosis and the endometrioid subtype (13,21-26) is often regarded as further supporting a role for inflammation in EUMET predisposition. Relevance to the serous subtype is supported by epidemiological evidence that endometriosis is associated with increased risk of low-grade serous extra-uterine Müllerian carcinomas (27). The apparent association between PID not involving endometriosis and risk of extra-uterine Müllerian cancer (28,29), as well as the evidence for a protective effect of anti-inflammatory drugs (30-33), provide further support for the notion that inflammation can influence the risk of extra-uterine Müllerian cancers including those showing serous differentiation.
Diet
The influence of diet has also been studied as it pertains to EUMET. Data regarding the role of dietary saturated fat is controversial. One retrospective study showed an increased risk of mucinous tumors in women with diets high in saturated fats (34) while another large study found only a weakly positive, non-linear association between extra-uterine Müllerian epithelial cancers of all subtypes except mucinous (35). Milk consumption and, more precisely, consumption of galactose, which is high in countries with elevated risks of EUMET such as Scandinavian countries and others, has been proposed as a risk factor for this disease. However, subsequent data could not confirm this association, including in individuals with a functional polymorphism in an enzyme involved in galactose metabolism (36-38).
Smoking
Multiple studies have linked cigarette smoking with risk of mucinous extra-uterine Müllerian cancer, but not of any other subtypes (39-41). This parallels the reported effect of smoking on endocervical carcinomas, which also show mucinous differentiation. The proposed mechanism is a combination of direct DNA damage by carcinogens in cigarette smoke superimposed on the ability of these carcinogens to accumulate in mucin-secreting cells. Not only has smoking not been shown to increase the rate of serous cancers, but also, it has been shown to decrease the relative risk of clear cell EUMET. These findings underscore differences in the mechanisms driving the development of various subtypes of EUMET and reinforce the view that serous tumors are not carcinogen-driven, but primarily hormone-driven.
Insights from genetic risk factors
Approximately 15% of all extra-uterine Müllerian carcinomas are familial (42). Almost all familial cases belonging to the serous subtype are due to germline mutations in the BRCA1 or BRCA2 genes, which are also associated with hereditary breast cancer. Approximately 40% of women carrying a germline BRCA1 mutation develop serous extra-uterine Müllerian epithelial cancer in their lifetime while the risk for BRCA2 mutation carriers is about 20% (43-49). Given that the risk of these tumors in the general population is only 1.7%, cancer-causing mutations in either one of these two genes are highly penetrant.
Little progress has been made in our understanding of the molecular basis for the strong association between the BRCA1 mutation carrier state and serous EUMET predisposition in spite of the decades that have elapsed since the isolation of this gene (50). Individuals with germline BRCA1 mutations are predisposed almost exclusively to cancers of the breast and reproductive organs in spite of the fact that this gene product is expressed ubiquitously in most cell types. Cellular processes associated with the full-length BRCA1 nuclear protein that are often invoked as potentially underlying the alleged tumor suppressor function of this protein include cell cycle regulation, regulation of apoptosis, DNA repair, chromatin remodeling, transcriptional regulation, X chromosome inactivation, and post-translational modification (51-55). The site specificity of cancers associated with BRCA1 mutations is surprising given the importance of these cellular processes in most mammalian cell types. The next section is an attempt to reconcile the wide tissue distribution of BRCA1 expression with the highly restricted pool of cell types that are at elevated risk of cancer in BRCA1 mutation carriers.
Potential link between genetic and reproductive risk factors
Cell non-autonomous hypothesis
BRCA1 and BRCA2 mutations, the main determinant of genetic risk for serous extra uterine Müllerian carcinomas, are rare in the sporadic form of this disease. An explanation, which would also account for the site specificity of the tumors that develop in BRCA1 and BRCA2 mutation carriers, is that inactivation of either one of these two genes might mimic important risk factors associated with sporadic extra-uterine Müllerian tumors, and would thus be redundant in individuals exposed to the sporadic risk factors. A possible scenario, given the fact that ovulatory activity is currently regarded as the most important risk factor for the sporadic form of these tumors, would be that a germline BRCA1 mutation could lead to alterations in the dynamics of the menstrual cycle, or in the levels of hormones associated with menstrual cycle progression, that would mimic the consequences of incessant ovulation. The fact that pregnancy or oral contraceptive use, both of which have a strong protective effect against sporadic EUMET, are also protective against these cancers in BRCA1 mutation carriers (56,57) is supportive of this idea of redundancy between genetic and non-genetic risk factors. The finding by Tone et al. (58) that the expression profile of high-grade serous carcinomas in BRCA mutation carriers resembles more closely that of the fallopian tube epithelium during the secretory phase than the proliferative phase of the menstrual cycle further underscores the role of ovulatory activity associated with menstrual cycle progression in the pathogenesis of BRCA-driven tumors.
This hypothesis is referred to as the cell non-autonomous hypothesis of cancer predisposition in BRCA1 mutation carriers. It implies that a germline BRCA1 mutation, in addition to directly influencing cells at risk of cancer development, also influences these cells indirectly and from a distance due to the consequences of such germline mutations on cells involved in menstrual cycle regulation that communicate with tissues at increased cancer risk via either endocrine or paracrine mechanisms. The hypothesis stipulates that the resulting changes in inter-cellular interactions between cells that regulate the menstrual cycle and serous extra-uterine Müllerian epithelium contribute to increased cancer risk in the latter. The hypothesis does not rule out the notion of direct, cell-autonomous effects superimposed on cell non-autonomous effects. The fact that cancerous tissues undergoing chemotherapy in BRCA1 mutation carriers appear to be under selective pressure to regain a normal BRCA1 function suggest a limited cell-autonomous role for BRCA1 mutations once the cancer phenotype has been established (59,60).
Insights from experimental animals
Chodankar et al. (61) sought to test the cell non-autonomous hypothesis in an animal model by investigating the consequences of disrupting communications between cells that control the estrous cycle and Müllerian epithelium. Given the central role of ovarian granulosa cells in regulating progression through the normal menstrual cycle, plus the fact that these cells secrete a variety of hormones such as estradiol, Müllerian inhibiting substance, and others that are known to influence EUMET cell growth in vitro, these authors used the Cre-lox system driven by a truncated form of the Fshr promoter to specifically inactivate the Brca1 gene in mouse granulosa cells. The mice indeed developed benign tumors that were clearly of epithelial (as opposed to granulosa cell) origin in strong support of a cell non-autonomous mechanism. These results raise the possibility that EUMET predisposition in human BRCA1 mutation carriers is due, at least in part, to decreased BRCA1 expression in ovarian granulosa cells, thereby disrupting control mechanisms that these cells exert on serous extra-uterine Müllerian epithelium. The findings by Hu et al. (62) that down-regulation of BRCA1 in primary cultures of human granulosa cells results in increased expression of aromatase, the rate-limiting enzyme in estradiol biosynthesis, is well in line with this hypothesis. It is not clear whether the same mechanism is also responsible for breast cancer predisposition in BRCA1 mutation carriers. The fact that ovulatory activity, which is largely controlled by ovarian granulosa cells, has a strong influence on predisposition to breast cancer in addition to EUMET suggests that the mechanisms of predisposition to breast cancer in mutation carriers could indeed be similar. This idea is further strengthened by the demonstration that oophorectomy can protect against breast cancer in BRCA1 mutation carriers (63).
Hormonal mediators of a cell non-autonomous mechanism
Hong et al. (64) sought further insights into the mechanisms whereby a BRCA1 mutation could contribute to cancer development via a cell non-autonomous mechanism. These authors specifically looked at the consequences of a Brca1 mutation in ovarian granulosa cells on the relative lengths of different phases of the estrus cycle and on circulating levels of specific hormones important for the regulation of this cycle. The estrus cycle is equivalent to the human menstrual cycle, the most important risk factor for the sporadic form of serous extra-uterine Müllerian carcinomas. Indeed, the average length of the proestrus phase, which corresponds to the follicular phase of the human menstrual cycle, was longer in mutant mice than in wild type littermates relative to the metestrus phase (corresponding to the luteal phase of the menstrual cycle). Mutant mice also had higher circulating levels of estradiol. They concluded that mice carrying a Brca1 mutation had both increased and prolonged estrogen stimulation unopposed by progesterone (64). The physiological relevance of these findings is underscored by a follow up study showing evidence of increased steroid hormone stimulation in end-organs targeted by estrogens such as the endometrium and long bones in Brca1-deficient mice (65). Mutant mice showed increased endometrial thickness and increased bone length and density, providing strong support for the idea that the presence of a Brca1 mutation in ovarian granulosa cells leads to increased estrogen stimulation. Although the authors used mice carrying homozygous Brca1 mutations in these studies in order to magnify the measurable consequences of such mutations, they also showed that mice carrying heterozygous mutations, such as present in human BRCA1 mutation carriers, showed effects similar to those present in homozygous mutants on sex steroid hormone biosynthesis, albeit of lesser magnitude (65).
Evidence for a cell non-autonomous mechanism in human
Although direct evidence for a cell non-autonomous effect of BRCA1 mutations in human is still lacking, observations with the UK Familial Ovarian Cancer Screening Study cohort (UKFOCSS), which showed that human BRCA1 mutation carriers have a sex hormone dysregulation and altered end-organ hormone-sensitivity during the menstrual cycle, provide strong support for this notion (66). Endometrial thickness was higher during the follicular phase [OR 1.11, 95% confidence interval (CI), 1.03-1.20; P=0.006) and lesser in the luteal phase (OR 0.90, 95% CI, 0.83-0.98; P=0.027) of BRCA1 mutation carriers compared to non-carriers while median luteal phase progesterone and estradiol levels were respectively 121% (P<0.001) and 33% (P=0.007) higher in mutant compared to controls. These results were not due to differences in oral contraceptive use in carriers (66).
Insights from The Cancer Genome Atlas (TCGA) dataset and other molecular profiling studies
Extensive data about molecular changes associated with high-grade serous extra-uterine Müllerian carcinomas are now readily available through TCGA (67), which includes a dataset of mRNA expression, miRNA expression, promoter methylation, and DNA copy number abnormalities in hundreds of such tumors, most of which were also subjected to whole exome sequencing analyses. Gene expression profiling studies that had preceded this project had already uncovered significant differences in the expression profile of each major extra-uterine Müllerian epithelial tumor subtype (68-71), leading the way to the development of potentially useful biomarker panels for distinguishing between subtypes associated with different clinical outcomes or with different therapeutic responses independent of classical histological parameters (72-74). Additional data from the Cancer Genome project led to the separation of high-grade serous extra-uterine Müllerian carcinomas into four different subgroups, respectively named differentiated, immunoreactive, mesenchymal, and proliferative, based on their expression profile of mRNA and miRNA (67). Separation into these four subtypes correlated well with independent findings by other investigators (74,75) as well as with three molecular subtypes identified based on miRNA expression. In addition, significant differences in overall survival were found based on expression of 108 genes selected from the TCGA dataset, 85 associated with good survival, as well as based on miRNA profiling subtypes.
Another contribution of TCGA that is of potential importance to our understanding of serous extra-uterine Müllerian epithelial tumor development is the observation that abnormalities in BRCA1 and BRCA2 appear to be mutually exclusive of amplification of CCNE1 in these tumors. Indeed, although CCNE1 amplification is present in 26% of tumors associated with wild type BRCA1 or BRCA2, it is seen in only 8% of those with mutations in either one of these two genes (67). This observation led to the idea that these cancers can be divided into two molecular classes, one driven by abnormalities in cellular pathways controlled by BRCA gene products and the other by CCNE1 amplification. This leaves approximately two thirds of these cancers with no characteristic abnormality except for P53 mutations and aneuploidy. Indeed, no individual gene or pathway other than P53 and those previously associated with familial predisposition to these tumors is unraveled from the Cancer Genome dataset. Although several mutations in potentially targetable pathways were found, none are frequent enough to provide potential therapeutic targets applicable to a significant proportion of these cancers. Severe numerical chromosomal abnormalities leading to aneuploidy is the only consistent molecular alteration associated with these tumors in addition to P53 mutations, the latter being presumably needed to overcome cell cycle checkpoints triggered by those ploidy changes. This has led to the notion that high-grade serous extra-uterine Müllerian carcinomas are primarily a disease of chromosomes, implying an important role for mitotic errors in their development.
Insights from in vitro studies
Cell culture models mimicking the genetic background of cancer precursor lesions, such as the model developed by Luo et al. (76), can provide clues about the cellular mechanisms leading to the near polyploid state that typically characterizes serous high-grade extra-uterine Müllerian carcinomas. These authors used cultures of cystadenomas, the benign counterparts of extra-uterine Müllerian carcinomas, in which SV40 large T antigen was forced-expressed. These genetic manipulations not only allow extension of in vitro life span by overcoming senescence, but also provide a genetic background not unlike that present in cancer precursor lesions because this antigen, by binding to RB and P53, leads to a constitutively active cell cycle in cells deprived of a functional P53 similarly to the situation in serous EUMET precursor lesions. Further characterization of this cell culture model suggested that accumulation of chromosomal abnormalities that are initially left unchecked due to the absence of a normal P53 function eventually overwhelms ability to overcome the spindle assembly checkpoint complex, resulting in a prolonged mitotic arrest. Recovery from such arrest, which is facilitated by lowering BRCA1 expression levels, results in resumption of cell cycle activity without cytokinesis, leading to tetraploidy and, if the process repeats itself, even higher degrees of polyploidy with ensuing aneuploidy (77,78). This scenario is a central element of the model of cancer development proposed in the next section.
It is interesting that cultures of extra-uterine Müllerian tumors of low malignant potential derived similarly and in parallel to those of cystadenomas, in spite of absence of normal P53 function due to forced expression of SV40 large T antigen, do not undergo a similar mitotic arrest and that their ploidy status remains substantially more stable than that of cystadenomas (79). It is tempting to relate such chromosome stability in culture to the fact that these tumors are typically diploid and genetically stable in vivo (80,81). In fact, aneuploid tumors of low malignant potential are associated with a more aggressive clinical course and their response to chemotherapeutic agents may be more typical of carcinomas, raising the possibility that at least some of the those tumors are carcinomas incorrectly diagnosed as LMP tumors (81-84). Indeed, the possibility of using ploidy status as a diagnostic tool to help distinguish tumors of low malignant potential from carcinomas has been suggested (80). Further understanding of the mechanisms underlying the apparent protection against chromosomal instability in cultures of tumors low malignant potential should further increase our understanding of the development of aneuploidy, one of the hallmarks of cancer.
A model for serous extra-uterine Müllerian carcinoma development
The model illustrated in Figure 1 is based on published data and arguments reviewed in this chapter. It is well established that cancer, in general, arises in a background of cell proliferation. It is probable that the main proliferation signal for serous extra-uterine Müllerian carcinoma precursors comes from the hormonal changes associated with the menstrual cycle that act cell non-autonomously as predicted by the aforementioned cell non-autonomous hypothesis. This cell non-autonomous scenario is magnified either by increased ovulatory activity (incessant ovulation) in individuals affected by sporadic cancers, or by the consequences of decreased BRCA1 or BRCA2 gene dosage in BRCA1/2 mutation carriers.
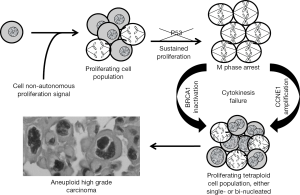
It is also well-established that extra-uterine Müllerian serous tumors almost always carry P53 mutations and that such mutations are present not only after acquisition of invasive and metastatic ability, but also in precursor lesions (85). The presence of such mutations in cells subjected to growth stimulation (via the cell non-autonomous pathway driven by the ovulatory cycle) inevitably leads to accumulation of chromosomal abnormalities due to absence of important cell cycle checkpoints controlled by P53, as observed in the in vitro model described by Luo et al. (76). Eventually, chromosomal abnormalities become overwhelming, resulting in a cell cycle arrest at the spindle assembly checkpoint (77,78). As illustrated in Figure 1, two different mechanisms can lead to recovery from this mitotic arrest, both leading to aneuploidy from a polyploid intermediate. In the absence of reduced or absent BRCA1 function either due to a germline mutation or promoter methylation, cells overcome this checkpoint and return to interphase without completing cytokinesis as described by Yu et al. (78). The resulting bi-nucleated cells may eventually show a single, enlarged nucleus following subsequent mitoses as depicted in Figure 1.
In the presence of normal BRCA1/2 function, cells arrested at the spindle assembly checkpoint might overcome this checkpoint via an alternate mechanism entailing CCNE1 amplification, as suggested by the Cancer Genome Atlas dataset, which shows frequent amplification of this cyclin in the absence of BRCA1/2 mutations (see above). The proposed mechanism is that centrosome duplication, one of the consequences of CCNE1 over-expression (86), leads to increased microtubule formation and anchoring (87), resulting in disruption of the spindle assembly checkpoint.
Thus, the combination of cell non-autonomous factors driven by hormonal factors or BRCA mutations, and of cell autonomous factors entailing recovery from a mitotic arrest at the spindle assembly checkpoint, leads to polyploid cell populations that invariably become aneuploid due to instability of the polyploid state. The combination of chromosomal imbalances, superimposed on accumulation of additional genetic defects provides an excellent soil for malignant transformation. The cell non-autonomous effects influence proliferation of serous extra-uterine Müllerian epithelium and account for the site specificity of the tumors associated with the BRCA mutation carrier state while the cell autonomous effects lead to the characteristic near polyploid state of these cancers. Relevance to human tumors is illustrated in Figure 2, which shows examples of either bi-nucleated cells (Figure 2A) or multipolar mitoses (Figure 2B) in histological photographs of representative high-grade serous extra-uterine Müllerian carcinomas.
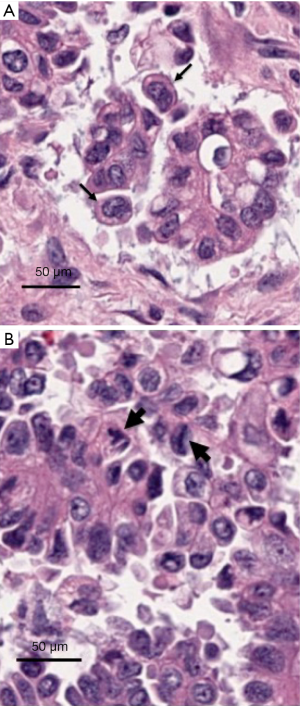
Concluding remarks
The cell non-autonomous mechanism of cancer predisposition discussed in this article has important implication for prevention of serous extra-uterine Müllerian carcinomas because it is based on circulating mediators of cancer risk that should be readily targetable with pharmacologic agents or even, perhaps, life style modifications. This underscores the importance of fully elucidating the nature of these mediators and of understanding their consequences on the biology of serous extra-uterine Müllerian epithelium. Better understanding of the cell autonomous mechanisms involved in serous extra-uterine Müllerian carcinoma development, on the other hand, should lead to strategies to reduce the likelihood of recovery from a cell cycle arrest thought to play an important role in the development of these cancers, both as cancer preventive and therapeutic measures. It is hoped that progress in both of these areas will have a significant impact on the morbidity and mortality associated with these important cancers of women.
Acknowledgments
Funding: This work was aided by grant (No. R01 CA133117) from the US National Institutes of Health and by a gift from the Ovarian Cancer Coalition of Greater California.
Footnote
Provenance and Peer Review: This article was commissioned by the Guest Editors (Franco Muggia and Eleonora Teplinsky) for the series “Epithelial Ovarian Cancer Treatment: Integrating Molecular Targeting” published in Translational Cancer Research. The article has undergone external peer review.
Conflicts of Interest: The author has completed the ICMJE uniform disclosure form (available at http://dx.doi.org/10.3978/j.issn.2218-676X.2015.01.06). The series “Epithelial Ovarian Cancer Treatment: Integrating Molecular Targeting” was commissioned by the editorial office without any funding or sponsorship. The author has no other conflicts of interest to declare.
Ethical Statement: The author is accountable for all aspects of the work in ensuring that questions related to the accuracy or integrity of any part of the work are appropriately investigated and resolved.
Open Access Statement: This is an Open Access article distributed in accordance with the Creative Commons Attribution-NonCommercial-NoDerivs 4.0 International License (CC BY-NC-ND 4.0), which permits the non-commercial replication and distribution of the article with the strict proviso that no changes or edits are made and the original work is properly cited (including links to both the formal publication through the relevant DOI and the license). See: https://creativecommons.org/licenses/by-nc-nd/4.0/.
References
- Dubeau L. The cell of origin of ovarian epithelial tumours. Lancet Oncol 2008;9:1191-7. [PubMed]
- Pike MC, Pearce CL, Peters R, et al. Hormonal factors and the risk of invasive ovarian cancer: a population-based case-control study. Fertil Steril 2004;82:186-95. [PubMed]
- Whittemore AS, Harris R, Itnyre J. Characteristics relating to ovarian cancer risk: collaborative analysis of 12 US case-control studies. II. Invasive epithelial ovarian cancers in white women. Collaborative Ovarian Cancer Group. Am J Epidemiol 1992;136:1184-203. [PubMed]
- Pike MC. Age-related factors in cancers of the breast, ovary, and endometrium. J Chronic Dis 1987;40:59S-69S. [PubMed]
- Fathalla MF. Factors in the causation and incidence of ovarian cancer. Obstet Gynecol Surv 1972;27:751-68. [PubMed]
- Dubeau L, Drapkin R. Coming into focus: the nonovarian origins of ovarian cancer. Ann Oncol 2013;24 Suppl 8:viii28-viii35.
- Zhou H, Luo MP, Schönthal AH, et al. Effect of reproductive hormones on ovarian epithelial tumors: I. Effect on cell cycle activity. Cancer Biol Ther 2002;1:300-6. [PubMed]
- Pearce CL, Chung K, Pike MC, et al. Increased ovarian cancer risk associated with menopausal estrogen therapy is reduced by adding a progestin. Cancer 2009;115:531-9. [PubMed]
- Rodriguez GC, Nagarsheth NP, Lee KL, et al. Progestin-induced apoptosis in the Macaque ovarian epithelium: differential regulation of transforming growth factor-beta. J Natl Cancer Inst 2002;94:50-60. [PubMed]
- Cramer DW, Barbieri RL, Fraer AR, et al. Determinants of early follicular phase gonadotrophin and estradiol concentrations in women of late reproductive age. Hum Reprod 2002;17:221-7. [PubMed]
- Risch HA. Hormonal etiology of epithelial ovarian cancer, with a hypothesis concerning the role of androgens and progesterone. J Natl Cancer Inst 1998;90:1774-86. [PubMed]
- Helzlsouer KJ, Alberg AJ, Gordon GB, et al. Serum gonadotropins and steroid hormones and the development of ovarian cancer. JAMA 1995;274:1926-30. [PubMed]
- Ness RB, Cottreau C. Possible role of ovarian epithelial inflammation in ovarian cancer. J Natl Cancer Inst 1999;91:1459-67. [PubMed]
- Terry KL, Karageorgi S, Shvetsov YB, et al. Genital powder use and risk of ovarian cancer: a pooled analysis of 8,525 cases and 9,859 controls. Cancer Prev Res (Phila) 2013;6:811-21. [PubMed]
- Cook LS, Kamb ML, Weiss NS. Perineal powder exposure and the risk of ovarian cancer. Am J Epidemiol 1997;145:459-65. [PubMed]
- Cramer DW, Liberman RF, Titus-Ernstoff L, et al. Genital talc exposure and risk of ovarian cancer. Int J Cancer 1999;81:351-6. [PubMed]
- Gertig DM, Hunter DJ, Cramer DW, et al. Prospective study of talc use and ovarian cancer. J Natl Cancer Inst 2000;92:249-52. [PubMed]
- Ness RB, Grisso JA, Cottreau C, et al. Factors related to inflammation of the ovarian epithelium and risk of ovarian cancer. Epidemiology 2000;11:111-7. [PubMed]
- Whittemore AS, Wu ML, Paffenbarger RS Jr, et al. Personal and environmental characteristics related to epithelial ovarian cancer. II. Exposures to talcum powder, tobacco, alcohol, and coffee. Am J Epidemiol 1988;128:1228-40. [PubMed]
- Wong C, Hempling RE, Piver MS, et al. Perineal talc exposure and subsequent epithelial ovarian cancer: a case-control study. Obstet Gynecol 1999;93:372-6. [PubMed]
- Brinton LA, Gridley G, Persson I, et al. Cancer risk after a hospital discharge diagnosis of endometriosis. Am J Obstet Gynecol 1997;176:572-9. [PubMed]
- Heaps JM, Nieberg RK, Berek JS. Malignant neoplasms arising in endometriosis. Obstet Gynecol 1990;75:1023-8. [PubMed]
- Ness RB. Endometriosis and ovarian cancer: thoughts on shared pathophysiology. Am J Obstet Gynecol 2003;189:280-94. [PubMed]
- Prefumo F, Todeschini F, Fulcheri E, et al. Epithelial abnormalities in cystic ovarian endometriosis. Gynecol Oncol 2002;84:280-4. [PubMed]
- Prowse AH, Manek S, Varma R, et al. Molecular genetic evidence that endometriosis is a precursor of ovarian cancer. Int J Cancer 2006;119:556-62. [PubMed]
- Vignali M, Infantino M, Matrone R, et al. Endometriosis: novel etiopathogenetic concepts and clinical perspectives. Fertil Steril 2002;78:665-78. [PubMed]
- Pearce CL, Templeman C, Rossing MA, et al. Association between endometriosis and risk of histological subtypes of ovarian cancer: a pooled analysis of case-control studies. Lancet Oncol 2012;13:385-94. [PubMed]
- Risch HA, Howe GR. Pelvic inflammatory disease and the risk of epithelial ovarian cancer. Cancer Epidemiol Biomarkers Prev 1995;4:447-51. [PubMed]
- Shu XO, Brinton LA, Gao YT, et al. Population-based case-control study of ovarian cancer in Shanghai. Cancer Res 1989;49:3670-4. [PubMed]
- Akhmedkhanov A, Toniolo P, Zeleniuch-Jacquotte A, et al. Aspirin and epithelial ovarian cancer. Prev Med 2001;33:682-7. [PubMed]
- Cramer DW, Harlow BL, Titus-Ernstoff L, et al. Over-the-counter analgesics and risk of ovarian cancer. Lancet 1998;351:104-7. [PubMed]
- Moysich KB, Mettlin C, Piver MS, et al. Regular use of analgesic drugs and ovarian cancer risk. Cancer Epidemiol Biomarkers Prev 2001;10:903-6. [PubMed]
- Tavani A, Gallus S, La Vecchia C, et al. Aspirin and ovarian cancer: an Italian case-control study. Ann Oncol 2000;11:1171-3. [PubMed]
- Risch HA, Marrett LD, Jain M, et al. Differences in risk factors for epithelial ovarian cancer by histologic type. Results of a case-control study. Am J Epidemiol 1996;144:363-72. [PubMed]
- Genkinger JM, Hunter DJ, Spiegelman D, et al. A pooled analysis of 12 cohort studies of dietary fat, cholesterol and egg intake and ovarian cancer. Cancer Causes Control 2006;17:273-85. [PubMed]
- Cramer DW, Greenberg ER, Titus-Ernstoff L, et al. A case-control study of galactose consumption and metabolism in relation to ovarian cancer. Cancer Epidemiol Biomarkers Prev 2000;9:95-101. [PubMed]
- Fung WL, Risch H, McLaughlin J, et al. The N314D polymorphism of galactose-1-phosphate uridyl transferase does not modify the risk of ovarian cancer. Cancer Epidemiol Biomarkers Prev 2003;12:678-80. [PubMed]
- Qin LQ, Xu JY, Wang PY, et al. Milk/dairy products consumption, galactose metabolism and ovarian cancer: meta-analysis of epidemiological studies. Eur J Cancer Prev 2005;14:13-9. [PubMed]
- Jordan SJ, Whiteman DC, Purdie DM, et al. Does smoking increase risk of ovarian cancer? A systematic review. Gynecol Oncol 2006;103:1122-9. [PubMed]
- Pan SY, Ugnat AM, Mao Y, et al. Association of cigarette smoking with the risk of ovarian cancer. Int J Cancer 2004;111:124-30. [PubMed]
- Purdie DM, Webb PM, Siskind V, et al. The different etiologies of mucinous and nonmucinous epithelial ovarian cancers. Gynecol Oncol 2003;88:S145-8. [PubMed]
- Claus EB, Schildkraut JM, Thompson WD, et al. The genetic attributable risk of breast and ovarian cancer. Cancer 1996;77:2318-24. [PubMed]
- Antoniou A, Pharoah PD, Narod S, et al. Average risks of breast and ovarian cancer associated with BRCA1 or BRCA2 mutations detected in case Series unselected for family history: a combined analysis of 22 studies. Am J Hum Genet 2003;72:1117-30. [PubMed]
- Brose MS, Rebbeck TR, Calzone KA, et al. Cancer risk estimates for BRCA1 mutation carriers identified in a risk evaluation program. J Natl Cancer Inst 2002;94:1365-72. [PubMed]
- Ford D, Easton DF, Bishop DT, et al. Risks of cancer in BRCA1-mutation carriers. Breast Cancer Linkage Consortium. Lancet 1994;343:692-5. [PubMed]
- Ford D, Easton DF, Stratton M, et al. Genetic heterogeneity and penetrance analysis of the BRCA1 and BRCA2 genes in breast cancer families. The Breast Cancer Linkage Consortium. Am J Hum Genet 1998;62:676-89. [PubMed]
- King MC, Marks JH, Mandell JB, et al. Breast and ovarian cancer risks due to inherited mutations in BRCA1 and BRCA2. Science 2003;302:643-6. [PubMed]
- Risch HA, McLaughlin JR, Cole DE, et al. Prevalence and penetrance of germline BRCA1 and BRCA2 mutations in a population series of 649 women with ovarian cancer. Am J Hum Genet 2001;68:700-10. [PubMed]
- Thompson D, Easton DBreast Cancer Linkage Consortium. Variation in cancer risks, by mutation position, in BRCA2 mutation carriers. Am J Hum Genet 2001;68:410-9. [PubMed]
- Miki Y, Swensen J, Shattuck-Eidens D, et al. A strong candidate for the breast and ovarian cancer susceptibility gene BRCA1. Science 1994;266:66-71. [PubMed]
- Deng CX, Scott F. Role of the tumor suppressor gene Brca1 in genetic stability and mammary gland tumor formation. Oncogene 2000;19:1059-64. [PubMed]
- Scully R, Livingston DM. In search of the tumour-suppressor functions of BRCA1 and BRCA2. Nature 2000;408:429-32. [PubMed]
- Starita LM, Parvin JD. The multiple nuclear functions of BRCA1: transcription, ubiquitination and DNA repair. Curr Opin Cell Biol 2003;15:345-50. [PubMed]
- Venkitaraman AR. Cancer susceptibility and the functions of BRCA1 and BRCA2. Cell 2002;108:171-82. [PubMed]
- Zheng L, Li S, Boyer TG, et al. Lessons learned from BRCA1 and BRCA2. Oncogene 2000;19:6159-75. [PubMed]
- McLaughlin JR, Risch HA, Lubinski J, et al. Reproductive risk factors for ovarian cancer in carriers of BRCA1 or BRCA2 mutations: a case-control study. Lancet Oncol 2007;8:26-34. [PubMed]
- Narod SA, Risch H, Moslehi R, et al. Oral contraceptives and the risk of hereditary ovarian cancer. Hereditary Ovarian Cancer Clinical Study Group. N Engl J Med 1998;339:424-8. [PubMed]
- Tone AA, Begley H, Sharma M, et al. Gene expression profiles of luteal phase fallopian tube epithelium from BRCA mutation carriers resemble high-grade serous carcinoma. Clin Cancer Res 2008;14:4067-78. [PubMed]
- Swisher EM, Sakai W, Karlan BY, et al. Secondary BRCA1 mutations in BRCA1-mutated ovarian carcinomas with platinum resistance. Cancer Res 2008;68:2581-6. [PubMed]
- Sakai W, Swisher EM, Karlan BY, et al. Secondary mutations as a mechanism of cisplatin resistance in BRCA2-mutated cancers. Nature 2008;451:1116-20. [PubMed]
- Chodankar R, Kwang S, Sangiorgi F, et al. Cell-nonautonomous induction of ovarian and uterine serous cystadenomas in mice lacking a functional Brca1 in ovarian granulosa cells. Curr Biol 2005;15:561-5. [PubMed]
- Hu Y, Ghosh S, Amleh A, et al. Modulation of aromatase expression by BRCA1: a possible link to tissue-specific tumor suppression. Oncogene 2005;24:8343-8. [PubMed]
- Kramer JL, Velazquez IA, Chen BE, et al. Prophylactic oophorectomy reduces breast cancer penetrance during prospective, long-term follow-up of BRCA1 mutation carriers. J Clin Oncol 2005;23:8629-35. [PubMed]
- Hong H, Yen HY, Brockmeyer A, et al. Changes in the mouse estrus cycle in response to BRCA1 inactivation suggest a potential link between risk factors for familial and sporadic ovarian cancer. Cancer Res 2010;70:221-8. [PubMed]
- Yen HY, Gabet Y, Liu Y, et al. Alterations in Brca1 expression in mouse ovarian granulosa cells have short-term and long-term consequences on estrogen-responsive organs. Lab Invest 2012;92:802-11. [PubMed]
- Widschwendter M, Rosenthal AN, Philpott S, et al. The sex hormone system in carriers of BRCA1/2 mutations: a case-control study. Lancet Oncol 2013;14:1226-32. [PubMed]
- Cancer Genome Atlas Research Network. Integrated genomic analyses of ovarian carcinoma. Nature 2011;474:609-15. [PubMed]
- Bonome T, Lee JY, Park DC, et al. Expression profiling of serous low malignant potential, low-grade, and high-grade tumors of the ovary. Cancer Res 2005;65:10602-12. [PubMed]
- Tsuda H, Ito YM, Ohashi Y, et al. Identification of overexpression and amplification of ABCF2 in clear cell ovarian adenocarcinomas by cDNA microarray analyses. Clin Cancer Res 2005;11:6880-8. [PubMed]
- Wamunyokoli FW, Bonome T, Lee JY, et al. Expression profiling of mucinous tumors of the ovary identifies genes of clinicopathologic importance. Clin Cancer Res 2006;12:690-700. [PubMed]
- Zorn KK, Bonome T, Gangi L, et al. Gene expression profiles of serous, endometrioid, and clear cell subtypes of ovarian and endometrial cancer. Clin Cancer Res 2005;11:6422-30. [PubMed]
- Jazaeri AA, Awtrey CS, Chandramouli GV, et al. Gene expression profiles associated with response to chemotherapy in epithelial ovarian cancers. Clin Cancer Res 2005;11:6300-10. [PubMed]
- Ouellet V, Guyot MC, Le Page C, et al. Tissue array analysis of expression microarray candidates identifies markers associated with tumor grade and outcome in serous epithelial ovarian cancer. Int J Cancer 2006;119:599-607. [PubMed]
- Tothill RW, Tinker AV, George J, et al. Novel molecular subtypes of serous and endometrioid ovarian cancer linked to clinical outcome. Clin Cancer Res 2008;14:5198-208. [PubMed]
- Bonome T, Levine DA, Shih J, et al. A gene signature predicting for survival in suboptimally debulked patients with ovarian cancer. Cancer Res 2008;68:5478-86. [PubMed]
- Luo MP, Gomperts B, Imren S, et al. Establishment of long-term in vitro cultures of human ovarian cystadenomas and LMP tumors and examination of their spectrum of expression of matrix-degrading proteinases. Gynecol Oncol 1997;67:277-84. [PubMed]
- Velicescu M, Yu J, Herbert BS, et al. Aneuploidy and telomere attrition are independent determinants of crisis in SV40-transformed epithelial cells. Cancer Res 2003;63:5813-20. [PubMed]
- Yu VM, Marion CM, Austria TM, et al. Role of BRCA1 in controlling mitotic arrest in ovarian cystadenoma cells. Int J Cancer 2012;130:2495-504. [PubMed]
- Yu J, Roy D, Brockmeyer AD, et al. Increased chromosomal stability in cultures of ovarian tumours of low malignant potential compared to cystadenomas. Br J Cancer 2007;96:1908-13. [PubMed]
- Friedlander ML, Russell P, Taylor IW, et al. Flow cytometric analysis of cellular DNA content as an adjunct to the diagnosis of ovarian tumours of borderline malignancy. Pathology 1984;16:301-6. [PubMed]
- Lai CH, Hsueh S, Chang TC, et al. The role of DNA flow cytometry in borderline malignant ovarian tumors. Cancer 1996;78:794-802. [PubMed]
- Burger CW, Prinssen HM, Baak JP, et al. The management of borderline epithelial tumors of the ovary. Int J Gynecol Cancer 2000;10:181-197. [PubMed]
- Kaern J, Trope C, Kjorstad KE, et al. Cellular DNA content as a new prognostic tool in patients with borderline tumors of the ovary. Gynecol Oncol 1990;38:452-7. [PubMed]
- Sykes PH, Quinn MA, Rome RM. Ovarian tumors of low malignant potential: a retrospective study of 234 patients. Int J Gynecol Cancer 1997;7:218-26.
- Lee Y, Miron A, Drapkin R, et al. A candidate precursor to serous carcinoma that originates in the distal fallopian tube. J Pathol 2007;211:26-35. [PubMed]
- Okuda M, Horn HF, Tarapore P, et al. Nucleophosmin/B23 is a target of CDK2/cyclin E in centrosome duplication. Cell 2000;103:127-40. [PubMed]
- Ogden A, Rida PC, Aneja R. Heading off with the herd: how cancer cells might maneuver supernumerary centrosomes for directional migration. Cancer Metastasis Rev 2013;32:269-87. [PubMed]