Targeting DNA repair: poly (ADP-ribose) polymerase inhibitors
Introduction
Ovarian cancer is the fifth leading cause of death in women in the United states with an estimated 21,980 new cases and 14,270 deaths in 2014 (1). Most women are diagnosed at an advanced stage and standard treatment is surgical debulking combined with a platinum and taxane-based chemotherapy regimen. This multimodal approach results in complete clinical remission in up to 75% of patients. However, only 30% of patients will be cured by initial treatment and the remaining will relapse within 2-3 years, thus potentially benefitting from consolidation. Recurrent ovarian cancer generally does not exhibit the same level of chemo-sensitivity, and cure after relapse is unlikely (2,3). Rational therapies directed toward specific molecular targets may help overcome the problem of disease resistance and change the prognosis for ovarian cancer patients (4). Poly (ADP-ribose) polymerase (PARP) inhibitors are a promising new class of targeted agents currently in clinical trials for ovarian cancer (5).
Mechanism of action
PARP enzymes catalyze a reaction called poly(ADP-ribosyl)ation, consisting of the sequential attachment of ADP-ribose moieties from NAD+ to the side chains of polar residues of target proteins. Poly(ADP-ribosyl)ation was first described in the 1960s but recently has received increasing attention due to its discovered role in multiple diseases including stroke, cardiac ischemia, inflammation, diabetes and cancer. PARP-1, PARP-2, and PARP-3 are a group of PARPs that display nuclear localization and DNA-dependent activity (6). These PARPs are essential in the response to DNA damage and loss of their function can result in carcinogenesis. PARPs have been implicated in DNA repair via three mechanisms:
- Base excisional repair (BER): PARP binds to single-stranded DNA breaks (SSBs) and recruits DNA repair proteins including DNA polymerase β, DNA ligase III, and scaffolding proteins such as X-ray cross complementing protein 1 (XRCC1) (7,8);
- Homologous recombination (HR) double-stranded DNA repair: PARP facilitates HR by recruiting the factors ataxia telangiectasia-mutated (ATM), mitotic recombination 11 (Mre11), and Nijmegen breakage syndrome 1 (Nbs1) to sites of double-stranded DNA breaks (DSBs) (9);
- Non-homologous end-joining (NHEJ): NHEJ is a pathway that repairs DSBs by directly ligating the break ends without a homologous template. NHEJ is less precise and more error prone that HR. PARP interacts with the DNA protein kinase complex involved in NHEJ (10).
Each day thousands of spontaneous SSBs occur in the genome of a normal cell and are repaired by PARP via BER. When PARP activity is compromised these SSBs persist and are converted into DSBs at DNA replication forks. In a normal cell, DSBs are repaired by HR, a mechanism that is separate from BER and therefore, even without PARP activity and the resulting loss of BER, DNA damage repair can occur effectively through the HR pathway. However, cells can have a double-hit whereby both BER and HR are compromised. These cells rely on NHEJ for damage repair but this pathway is less accurate and results in errors that cause DNA instability, chromosomal aberrations and eventually cell death (5,11). The double-hit of HR and BER dysfunction results in “synthetic lethality” or the potent and lethal synergy between two otherwise nonlethal events (12). The concept of synthetic lethality emerged from Drosophila studies whereby a combination of mutations in two or more separate genes led to cell death. This is in contrast to cell viability, which occurs when only one of the genes is mutated or deleted. The concept gave rise to a unique and exciting method of targeting cancer. Synthetically lethal therapeutic approaches to cancer exploit the inherent differences between cancer cells, which may already have one hit or mutation, and normal cells in a way that is often not feasible with conventional chemotherapeutic agents (13).
Preclinical data and rationale in BRCA mutated cells
The BRCA1 and BRCA2 genes were first cloned in the 1990s. They encode tumor-suppressor proteins that are essential components in HR. Dysfunction of BRCA1 and BRCA2 is associated with an elevated risk of developing multiple cancers, among which breast and ovarian are most common. BRCA1/2 mutations were initially considered uncommon, however, research over the past 2 decades contests this assumption. Germline BRCA1/2 mutations are now thought to contribute to 15% of epithelial ovarian cancers and 23% of high grade serous carcinomas (HGSC) (14,15). The concept of synthetic lethality was the rationale for initially investigating PARP inhibitors in BRCA1/2 mutated cell lines. In patients with BRCA mutations, every cell carries a heterozygous loss-of-function BRCA mutation, but cells in this heterozygous state have a functional HR system. Tumor cells, however, have lost the remaining wild type BRCA allele and therefore HR becomes dysfunctional. When exposed to PARP inhibitors, normal cells can repair DNA damage through HR and survive but tumor cells, which are missing HR and BER, must rely on the more error-prone NHEJ. NHEJ mediated repair causes DNA instability and eventual cell death in the BRCA mutated cells (5,11). PARP inhibitors utilize this tumor-specific defect, causing selective tumor toxicity and sparing normal cells (12).
Synthetic lethality with PARP inhibitors was first reported in two landmark studies published in 2005 (16,17). Farmer et al. (16) found that cells deficient in BRCA1 or BRCA2 function showed a greater than 1,000-fold enhanced sensitivity to PARP inhibitors when compared to wild-type cells. PARP inhibition elicited a profound arrest in the G2 or M phase of the cell cycle for these BRCA-deficient cells. The efficacy of PARP inhibition was reproduced in vivo with BRCA2-deficient mouse models. Bryant et al. (17) demonstrated that HR-deficient cell lines were sensitive to all PARP inhibitors and correction of the HR defect reversed this sensitivity. Furthermore, by comparing BRCA2-deficient cell lines to controls, they showed that sensitivity to the PARP inhibitor is a direct consequence of the BRCA2 defect.
Three years later Evers et al. (18) established a BRCA2-deficient mammary tumor cell line to further study PARP inhibition. This group showed that the PARP inhibitor olaparib caused the strongest differential growth inhibition in BRCA2-deficient vs. BRCA2-proficient mammary tumor cells. They performed drug combination studies and showed a synergistic cytotoxicity of olaparib and cisplatin in BRCA2-deficient cells but not in BRCA2-proficient controls. Xenograft models have been established for other types of solid tumors including cervix, colon, lung, glioblastoma, and squamous cell carcinoma of the head and neck and have shown that PARP inhibitors given hours prior to radiation delayed tumor growth or reduced tumor volume (19-24). Additive or synergistic antitumor effects have been shown with multiple drugs in addition to cisplatin including doxorubicin and topotecan (25,26).
Beyond BRCA mutation carriers
While PARP inhibition is usually thought of in the context of BRCA germline mutations, synthetic lethality requires only that the HR system be compromised and there are ways to achieve this outcome besides BRCA1/2 germline mutations. Alternative mechanisms of defective HR, sometimes referred to as conferring cells with “BRCAness,” result from somatic BRCA1/2 mutations, BRCA1 promoter methylation, and other genetic or epigenetic abnormalities of HR pathway genes. Somatic mutations in BRCA1/2 have been identified in up to 7% of ovarian cancers. BRCA1 inactivation via epigenetic silencing has been identified in 35% of sporadic serous ovarian cancers (14,15,27-29). Inherited mutations in genes that work in concert with BRCA1/2 including ATM, PTEN, MRE-11, EMSY and Fanconi anemia (FA) proteins are affected in 17% to 60% of patients with ovarian cancer (30-33). Finally, a molecular characterization study of HGSC discovered that approximately 50% of HGSCs harbor a genetic or epigenetic alteration in the HR pathway (34). These alternative mechanisms of BRCA dysfunction render cells sensitive to PARP inhibition. This explains why PARP inhibitor activity has been found in patients without germline BRCA1/2 mutations and supports the study of PARP inhibitors in sporadic ovarian cancers that display BRCAness (35). Currently, many phase III PARP inhibitor trials include patients without germline BRCA1/2 mutations.
Specific drugs and clinical trials
Table 1 outlines the sentinel completed phase II studies of PARP inhibitors in ovarian cancer. Areas currently being explored include using PARP inhibitors as induction agents in front line treatment, consolidation agents following front line treatment or following platinum sensitive recurrence that has responded to treatment with a platinum. PARP inhibitors have been used as single agents and in combination with cytotoxic drugs or other targeted therapies.
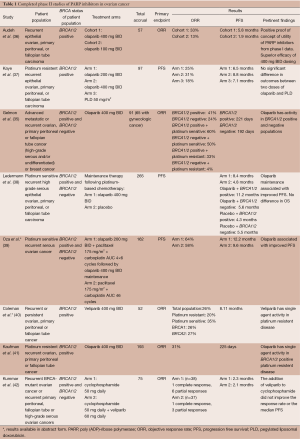
Full table
Olaparib (AZD2281)
Olaparib is an oral PARP-1 and PARP-2 inhibitor. Olaparib has been the most extensively studied among the drugs in this class. In 2009, Fong et al. (12) published a phase I trial of olaparib in 60 patients with refractory solid tumors. The study was enriched for patients carrying BRCA1/2 mutations. The maximum tolerated dose of olaparib was established at 400 mg BID with only minimal adverse effects observed (mainly fatigue and gastrointestinal side effects). BRCA1/2 mutation carriers had a significant objective tumor response. Eight of nine BRCA-associated ovarian cancer patients exhibited a partial response (PR) and 63% of these patients derived clinical benefit, defined either as a Response Evaluation Criteria in Solid Tumors (RECIST) radiologic response or tumor marker response or stable disease (SD) of four months or greater. In an expanded cohort of 50 ovarian cancer patients with germline BRCA1/2 mutations, 40% of patients demonstrated a radiologic or CA125 response (43). The expansion study supported the role of olaparib in BRCA-mutated ovarian cancer and revealed that PARP inhibition is closely related to platinum sensitivity. The clinical benefit from olaparib was highest in the platinum sensitive group (69%), followed by the platinum resistant group (46%) and lowest in the patients with platinum refractory disease (23%). This study also found an association between overall platinum-free interval and response to PARP inhibition. These findings are not surprising given that a dysfunctional HR system confers sensitivity to both platinum drugs and PARP inhibitors (44).
In 2010, Audeh et al. (36) published an international, multicenter phase II trial of olaparib in two sequential cohorts of patients with BRCA1/2 mutations and recurrent ovarian cancer. Fifty seven patients were enrolled: 33 in the first cohort receiving olaparib at 400 mg BID and 24 in the second cohort receiving olaparib at 100 mg BID. The primary endpoint was objective response rate (ORR). The ORR in the first cohort was 33% and in the second cohort 13%. The drug was well tolerated and the results confirmed phase I findings, providing positive proof of concept of the efficacy and tolerability of targeted treatment with olaparib in BRCA-mutated advanced ovarian cancer, with superior efficacy in the 400 mg BID group.
Following the initial olaparib studies, Kaye et al. (37) published a multicenter, randomized phase II trial designed to assess the safety and efficacy of olaparib at two doses (200 mg BID and 400 mg BID) vs. pegylated liposomal doxorubicin (PLD). The study enrolled 97 patients with platinum resistant ovarian cancer and a confirmed BRCA1/2 mutation. The study found comparable responses between olaparib (at both doses) and PLD. Interestingly the efficacy of PLD was greater than expected which may be explained by greater sensitivity among BRCA1/2 mutated patients to both PARP inhibitors and PLD. This study shows that the activity of an oral PARP inhibitor is essentially equivalent to an intravenous chemotherapeutic drug.
Olaparib has also been investigated in non-BRCA mutation ovarian cancers. Gelmon et al. (35) published a phase II study examining olaparib at 400 mg BID in HGSC or poorly differentiated ovarian carcinoma and triple-negative breast cancer. This study enrolled 91 patients and included a mix of BRCA1/2 positive, negative and unknown genotypes. A response rate of 24% was found in HGSC and 41% in patients with BRCA1/2 mutations. Importantly, this study demonstrated that PARP inhibition had utility in both BRCA1/2 mutated and non-mutated ovarian cancer, thus expanding the population of patients who could receive clinical benefit from PARP inhibitors.
Ledermann et al. (38) performed a randomized, double-blind, placebo-controlled phase II study of olaparib in platinum-sensitive relapsed ovarian cancer. In contrast to prior studies, this study investigated olaparib in the maintenance setting. Two hundred and sixty five patients were enrolled, including patients with and without known BRCA1/2 mutations, and randomized to olaparib 400 mg BID vs. placebo. This study found a statistically significant improvement in the primary endpoint progression free survival (PFS) in the olaparib maintenance group; however there was no difference in overall survival (OS). A preplanned retrospective subgroup analysis found that regardless of BRCA mutation status, the olaparib cohort had a decreased risk for progression. This study reaffirmed the role of PARP inhibitors in patients with ovarian cancer, regardless of BRCA mutational status (45).
In December 2014 the U.S. Food and Drug Administration (FDA) granted olaparib accelerated approval for monotherapy in patients with germline BRCA1/2 mutations and recurrent ovarian cancer, making olaparib the first of the PARP inhibitors to receive FDA approval. The approval was based on the results of the recently published phase II study by Kaufman et al. (41) of olaparib 400 mg BID for patients with a germline BRCA1/2 mutation and recurrent cancer. This study included patients who had received prior platinum and were considered to be platinum resistant or not suitable for further platinum therapy. The final results of this trial included 193 ovarian cancer patients and found an overall response rate of 31% and a median duration of response of 225 days. The safety data for this approval was a compilation of data from the Kaufman et al. (41) phase II study and the randomized, double-blind, placebo-controlled phase II study by Ledermann et al. (38). Conversion of the accelerated approval into full approval is contingent on a full review of data from two ongoing phase III olaparib trials for BRCA mutated patients in the maintenance setting, one following frontline chemotherapy (NCT01844986) and the other following treatment of a platinum sensitive recurrence (NCT01874353).
In addition to monotherapy, olaparib has been studied in combination with other anti-cancer drugs. There are multiple phase I and phase II trials of PARP inhibitors combined with conventional cytotoxic chemotherapies based on preclinical evidence that PARP inhibitors potentiate the antineoplastic effects of chemotherapy (26). The damage induced by chemotherapy is often repaired by BER, which is disrupted by PARP inhibition (46). Preliminary published results from a phase II trial comparing carboplatin and paclitaxel plus olaparib for six cycles followed by olaparib maintenance vs. carboplatin and paclitaxel alone demonstrate an improved PFS for the olaparib arm (12.2 vs. 9.6 months) (39). Olaparib has been studied in combination with other chemotherapies including cisplatin, cisplatin and gemcitabine, carboplatin, and PLD (47-50). Overall, the combination studies support an improved PFS but also reveal that due to overlapping hematologic toxicities, PARP inhibitors make it difficult for patients to achieve the full dose of the standard chemotherapy (46). Perhaps this information, along with the improvement in PFS in the maintenance studies supports the notion that the ideal use of PARP inhibitors may be in the maintenance setting or as monotherapy in the recurrent setting. Olaparib is currently being studied in combination with carboplatin in a phase I trial for patients with refractory or recurrent gynecologic cancer (NCT01237067).
Finally, olaparib is being investigated in combination with other targeted biologic agents. Angiogenesis inhibitors have proven activity in ovarian cancer with an underlying mechanism involving inhibition of vascular endothelial growth factor receptor (VEGFR). VEGFR inhibition can propagate DNA damage through down regulation of DNA repair proteins. Preclinical data support a relationship between PARP inhibition and the VEGF pathway and suggest a synergistic relationship when drugs from each class are combined (51-53). Liu et al. (54) recently published the results of a phase I trial combining olaparib and cediranib, an anti-angiogenic agent with activity against multiple VEGFRs, and found that the combination improves PFS in women with recurrent platinum sensitive HGSC. Given the promising activity, NRG is planning a phase II study to further investigate the efficacy of this combination. Dean et al. (55) examined the use of olaparib with bevacizumab, a recombinant antibody that prevents VEGF receptor binding, in 12 patients with advanced solid tumors and found the combination to be generally well tolerated. Of note, the majority of included patients had colorectal and breast cancer and there were no patients with ovarian cancer. Olaparib has also been combined with phosphoinositide-3-kinase (PI3K) inhibitors based on the finding that the PI3K pathway is associated with genomic instability and is activated in up to 70% of ovarian cancers. Animal experiments provide rationale for the combination of PI3K inhibition and olaparib and there is currently an ongoing phase I study of olaparib and the PI3K inhibitor BKM120 (NCT01623349) (56,57). As the use of targeted biologic agents in ovarian cancer continues to rise, future studies will likely look more closely at the combination of these drugs with PARP inhibitors. Preliminary data combining anti-angiogenenic agents and PI3K inhibitors with PARP inhibitors appears promising, and we eagerly await further confirmatory data.
Veliparib (ABT888)
Veliparib is another oral PARP-1 and PARP-2 inhibitor. Veliparib has been studied in combination with multiple standard chemotherapeutic agents including topotecan, irinotecan, doxorubicin and cyclophosphamide (58-61). A phase I trial by Kummar et al. (60) demonstrated that the combination of veliparib with metronomic cyclophosphamide was well tolerated with promising activity in a subset of patients with BRCA mutations. However, Kummar et al. (42) published the results of a randomized phase II trial of oral cyclophosphamide with and without veliparib 60 mg daily in patients with pretreated BRCA-mutant ovarian cancer or high-grade serous ovarian, primary peritoneal and fallopian tube cancers. They found that the addition of veliparib did not improve the response rate or the median PFS.
Reiss et al. (62) recently reported the results of a phase I study of veliparib in combination with low-dose whole abdominal radiation therapy in patients with advanced solid malignancies and peritoneal carcinomatosis. Eight of the 22 patients included in the study had ovarian and fallopian tube cancer and this subset of patients had better PFS and OS than other cancer types and better quality life. The authors concluded that the combination of veliparib and radiation therapy is well tolerated and results in prolonged disease stability in the ovarian and fallopian tube cancer subpopulation.
Preliminary results from a trial of veliparib monotherapy provide the first phase II evidence that single-agent veliparib has activity in treating platinum resistant, BRCA-mutated ovarian cancers (NCT01540565/GOG-0280). This study included 52 patients (50 of them evaluable for efficacy and toxicity), 78% harboring a BRCA1 mutation and 22% a BRCA2 mutation. Veliparib administered at 400 mg BID resulted in an overall response rate of 26%, with two complete responses and 11 PRs. The response rate was 20% in platinum resistant patients and 35% in platinum sensitive patients. Veliparib had a reported PFS of 8.11 months and OS of 19.7 months (40).
Ongoing studies include a phase I trial combining veliparib with bevacizumab, paclitaxel and carboplatin in frontline ovarian cancer (NCT00989651) and a study combining veliparib and PLD (NCT01145430). Ongoing phase II trials include single agent veliparib administered at 300 mg BID in relapsed BRCA-associated ovarian cancer (NCT01472783) and a study of veliparib in combination with temozolomide vs. PLD (NCT01113957).
Niraparib (MK4827)
Niraparib is an oral PARP-1 and PARP-2 inhibitor, found to inhibit tumor growth in models with loss of BRCA and PTEN function. In a phase I study, Sandhu et al. (63) administered niraparib to a small cohort of patients enriched for BRCA-deficient and sporadic cancers associated with defects in HR repair. Thirty-nine patients were treated, 11 of which had germline BRCA1/2 mutations. Eight of the BRCA1/2 mutation carriers with ovarian cancer had a PR and antitumor activity was also found in sporadic HGSC. There is currently a phase III study of niraparib vs. placebo maintenance therapy for patients with platinum sensitive recurrent HGSC (NCT01847274).
Rucaparib (CO338, formerly known as AGO14699 and PFO1367338)
Rucaparib, also an oral inhibitor of PARP-1 and PARP-2, has shown antiproliferative activity in ovarian cancer cell lines (64). The phase I study of rucaparib demonstrated anti-cancer responses in the platinum resistant and platinum sensitive ovarian and primary peritoneal subgroups. Phase I studies have explored rucaparib in combination with temozolomide, carboplatin, carboplatin and paclitaxel and cisplatin and pemetrexed (65-67). The preliminary results of a phase II study of rucaparib monotherapy in 41 patients with either breast or ovarian cancer and known BRCA1/2 mutations found a clinical benefit rate of 32% but an ORR of only 5%. Twenty-six percent of patients achieved SD for at least 4 months (68). Rucaparib currently is being studied as part of three ongoing clinical trials. A phase I/II study of rucaparib will evaluate drug toxicity and pharmacokinetics in patients with germline BRCA1/2 mutations and ovarian cancer or other solid tumors (NCT01482715). ARIEL2 (NCT01891344) is an accruing phase II trial of rucaparib in platinum sensitive, relapsed, high-grade epithelial ovarian cancer. Finally ARIEL3 (NCT01968213) is exploring rucaparib maintenance therapy following a platinum treatment in relapsed HGSC and endometrioid ovarian cancer. These two studies hope to define a molecular signature of HR dysfunction in ovarian cancer that correlates with response to rucaparib and enables selection of appropriate ovarian cancer patients for treatment with rucaparib.
Other PARP inhibitors
There are multiple other PARP inhibitors that have not yet been included in late phase ovarian cancer trials. BMN 673 is an oral PARP-1 and PARP-2 inhibitor that has received attention because in addition to inhibiting PARP activity, it has demonstrated 100-fold more potent trapping of PARP-DNA complexes than other PARP inhibitors. While PARP inhibitors were initially developed as catalytic inhibitors to block PARP-associated repair of DNA single-stranded breaks, recently an additional mechanism of action, the trapping of PARP-DNA complexes, has emerged as a second important instrument of anti-cancer activity. BMN 673 has undergone phase I testing in patients with ovarian cancer and is currently being evaluated in a phase I/II pilot study in patients with advanced solid tumors and BRCA1/2 mutations (NCT01989546) (69). E7449, another PARP inhibitor, is being evaluated in a phase I/II trial in patients with advanced solid tumors or B-cell malignancies alone and in combination with temozolomide or carboplatin and paclitaxel (NCT01618136). Other PARP inhibitors that have been clinically tested but not yet in ovarian cancer include AZD2461, CEP9722, E7016, and INO-1001 (46).
Iniparib is a compound that was initially developed as a PARP inhibitor and received attention when phase II data demonstrated that in patients with triple-negative breast cancer, the addition of iniparib to gemcitabine and carboplatin extended PFS and OS with minimal side effects (70). However the follow-up phase III trial did not show a significant improvement in either outcome (71). Many proposed poor trial design as an explanation for the discordance in results between the phase II and phase III trials. However, recent studies have raised the concern that iniparib is not a true PARP inhibitor due to in vitro data to suggest that iniparib is structurally distinct from other PARP inhibitors and poorly inhibits PARP activity (72-74).
Challenges in ovarian cancer
Despite promising results from the initial olaparib trials, PARP inhibitors have not shown an improvement in OS. The recent FDA approval of olaparib represents an important step forward for this class of drugs, however, there remain several challenges for the clinical development of PARP inhibitors. To begin, PARP inhibitors have demonstrated maximal effect in germline BRCA-associated tumors and sporadic cases with deficient HR. While testing for germline BRCA mutations is available, there currently is not a validated biomarker for HR-deficient ovarian cancer that can predict response to PARP inhibition. This limits the use of PARP inhibitors in patients who lack a known BRCA mutation and this population compromises the majority of ovarian cancer cases. However, with the growing availability and scope of multiplex-gene testing and massive parallel sequencing, patients with mutations in other HR related genes are being identified and may prove to be good PARP inhibitor candidates. Furthermore, the role of tumor BRCA mutations and response to PARP inhibitors needs to be further elucidated.
In addition to difficulties identifying appropriate patient candidates, there also are patients with HR deficient tumors who do not respond or develop resistance to PARP inhibition. This suggests that tumors can have both de novo and acquired resistance to PARP inhibition. Multiple mechanisms of acquired PARP inhibitor resistance have been proposed. There is data to suggest that exposure to DNA damaging agents can lead to re-expression of BRCA1 by genetic reversion. This causes a partial restoration of HR-mediated DNA repair and renders cells less sensitive to PARP inhibition. Another mechanism of resistance involves increased expression of the Mdr1a/b genes. These genes encode the drug efflux transporter P-glycoprotein. Elevated expression of this target results in the need for increasing drug concentrations required for effective inhibition. Finally, loss of 53BP1 has been shown to cause suppression of NHEJ which also leads to PARP inhibitor resistance (46,75,76).
Another barrier to clarifying the role for PARP inhibitors in ovarian cancer is that trials for these drugs have largely been in combination with other chemotherapeutic agents at multiple chronological points in the disease course. As a result, the ideal method and timing of PARP inhibitor delivery remains unclear. Finally, the differences between different PARP inhibitors have yet to be fully defined. Preclinical data suggest that the potency in trapping PARP differs markedly among niraparib, olaparib and veliparib, and the patterns of trapping were not correlated with the catalytic inhibitory properties for each drug (77). Further studies will be needed to clarify the differences in toxicity, pharmacokinetics and efficacy between these related but unique drugs.
Future directions
PARP inhibitors are undoubtedly a promising new class of anti-cancer agents with proven activity in ovarian cancer. However, the full potential of these drugs has yet to be realized. Questions remain regarding the ideal use of PARP inhibitors. Does administration of PARP inhibitors at a specific time, recurrent vs. adjuvant vs. maintenance, procure a greater benefit? Do side effect profiles make monotherapy more favorable than combination with standard chemotherapeutic agents? Is there a role of PARP inhibitors as chemoprevention? Perhaps women who are at high risk for developing ovarian cancer but are not ready to accept risk-reducing bilateral salpingo-oophorectomy and surgical menopause could benefit from prophylaxis with these drugs. Furthermore, even after risk-reducing salpingo-oophoretomy women remain at risk for primary peritoneal cancer and perhaps there is a role for PARP inhibitor chemoprevention in this setting. Hopefully many of these questions will be answered by ongoing clinical trials.
The combination of PARP inhibitors with non-cytotoxic agents is another exciting area under investigation. As described previously, olaparib is being studied in combination with inhibitors of the VEGFR pathway and PI3K inhibitors. The combination of PARP inhibitors with ionizing radiation is another logical combination based on the role of PARP in DNA repair. Preclinical studies confirm that PARP inhibitors can sensitize malignant cells to radiation and experiments with PARP-1 knockout mice demonstrate increased sensitivity to gamma-radiation (20,78-80). Although veliparib was previously studied in combination with low-dose whole abdominal radiation therapy in patients with ovarian and fallopian tube cancer, there currently are no active trials investigating PARP inhibitors with radiation in gynecologic malignancies. Inclusion of patients with gynecologic cancer in trials that combine PARP inhibitors with radiation and other non-cytotoxic anti-cancer agents is imperative to fully grasp the potential of PARP inhibition in this patient population.
While the chronology and setting of PARP administration remains an important area of study, the more pressing issue is identifying the patients who will benefit from PARP inhibitor therapy. By understanding the pathways of PARP inhibition, synthetic lethality and NHEJ, we have begun to uncover the anti-cancer mechanism of this class of drugs. However, there is not yet a validated predictive biomarker to distinguish the ideal candidates and early pharmacokinetic and pharmacodynamics data were unable to correlate PAR levels with PARP activity. Several attempts have been made to discover a biomarker useful for PARP inhibition. Duan et al. (81) utilized immunofluorescence assays of tumors to evaluate the functional capacity of the FA pathway, which is important in BRCA mediated DNA repair. Fifteen different complementation groups for FA have been defined, including FANCD2. When FANCD2 is ubiqinated it can activate DNA repair proteins in the downstream FA/BRCA pathway and repair DNA damage (82,83). There is a currently accruing phase I clinical trial that will measure FANCD2 foci formation in peripheral blood mononuclear cells in patients with metastatic, unresectable or recurrent solid tumors being treated with veliparib and mitomycin C (NCT01017640). Mukhopadhyay et al. (84) used immunofluorescence assays to quantify RAD51 foci, a marker for repair of DSB, from ascites and utilized this as a surrogate for HR function. This measurement was then found to correlate with the in vitro cytotoxicity of rucaparib (85). The RAD51 assay could allow clinicians to identify patients with dysfunctional HR who will respond to PARP inhibitors.
Finally, the increasing use of multi-gene panels may also help define groups of patients for PARP inhibitor administration. Multi-gene panels rely on massive parallel sequencing analysis and can detect highly penetrant genes known to cause hereditary cancers but also the less common deleterious mutations and variants of uncertain significance in known carcinogenic pathways like HR. The potential clinical implications of this technology coupled with falling prices of sequencing could make single gene testing an obsolete practice (86). However, the use of massive parallel sequencing to determine PARP inhibitor utility has yet to be studied in a prospective fashion. A trial addressing this question will be important to help clinicians better utilize genetic testing results and allow them to capture a larger percentage of patients likely to benefit from PARP inhibition (87).
In conclusion, PARP inhibitors have demonstrated exciting potential in the treatment of ovarian cancer. Completed phase II trials reveal a role for PARP inhibitors as monotherapy for BRCA1/2 mutated and non-mutated platinum sensitive and platinum resistant ovarian cancer. PARP inhibitors have also been successfully combined with other cytotoxic chemotherapies and targeted biologics. Finally ongoing studies are evaluating the role of PARP inhibition as consolidation, following completion of either front line treatment or following treatment for recurrent disease. The recent FDA approval of olaparib in BRCA-mutated recurrent ovarian cancer further highlights the promise of PARP inhibitors in the treatment of women with ovarian cancer. Hopefully ongoing and future clinical trials that better define strategies of use and the ideal patient populations will make these drugs even more successful in the treatment of gynecologic malignancies.
Acknowledgments
Funding: None.
Footnote
Provenance and Peer Review: This article was commissioned by the Guest Editors (Franco Muggia and Eleonora Teplinsky) for the series “Epithelial Ovarian Cancer Treatment: Integrating Molecular Targeting” published in Translational Cancer Research. The article has undergone external peer review.
Conflicts of Interest: Both authors have completed the ICMJE uniform disclosure form (available at http://dx.doi.org/10.3978/j.issn.2218-676X.2015.01.09). The series “Epithelial Ovarian Cancer Treatment: Integrating Molecular Targeting” was commissioned by the editorial office without any funding or sponsorship. The authors have no other conflicts of interest to declare.
Ethical Statement: The authors are accountable for all aspects of the work in ensuring that questions related to the accuracy or integrity of any part of the work are appropriately investigated and resolved.
Open Access Statement: This is an Open Access article distributed in accordance with the Creative Commons Attribution-NonCommercial-NoDerivs 4.0 International License (CC BY-NC-ND 4.0), which permits the non-commercial replication and distribution of the article with the strict proviso that no changes or edits are made and the original work is properly cited (including links to both the formal publication through the relevant DOI and the license). See: https://creativecommons.org/licenses/by-nc-nd/4.0/.
References
- Siegel R, Ma J, Zou Z, et al. Cancer statistics, 2014. CA Cancer J Clin 2014;64:9-29. [PubMed]
- Covens A, Carey M, Bryson P, et al. Systematic review of first-line chemotherapy for newly diagnosed postoperative patients with stage II, III, or IV epithelial ovarian cancer. Gynecol Oncol 2002;85:71-80. [PubMed]
- Gadducci A, Sartori E, Maggino T, et al. Analysis of failures after negative second-look in patients with advanced ovarian cancer: an Italian multicenter study. Gynecol Oncol 1998;68:150-5. [PubMed]
- Pothuri B. The circuitous path of PARP inhibitor development in epithelial ovarian cancer. Oncology (Williston Park) 2012;26:144-6. [PubMed]
- Do K, Chen AP. Molecular pathways: targeting PARP in cancer treatment. Clin Cancer Res 2013;19:977-84. [PubMed]
- Liscio P, Camaioni E, Carotti A, et al. From polypharmacology to target specificity: the case of PARP inhibitors. Curr Top Med Chem 2013;13:2939-54. [PubMed]
- Houtgraaf JH, Versmissen J, van der Giessen WJ. A concise review of DNA damage checkpoints and repair in mammalian cells. Cardiovasc Revasc Med 2006;7:165-72. [PubMed]
- El-Khamisy SF, Masutani M, Suzuki H, et al. A requirement for PARP-1 for the assembly or stability of XRCC1 nuclear foci at sites of oxidative DNA damage. Nucleic Acids Res 2003;31:5526-33. [PubMed]
- Haince JF, McDonald D, Rodrigue A, et al. PARP1-dependent kinetics of recruitment of MRE11 and NBS1 proteins to multiple DNA damage sites. J Biol Chem 2008;283:1197-208. [PubMed]
- Wang M, Wu W, Wu W, et al. PARP-1 and Ku compete for repair of DNA double strand breaks by distinct NHEJ pathways. Nucleic Acids Res 2006;34:6170-82. [PubMed]
- Patel AG, Sarkaria JN, Kaufmann SH. Nonhomologous end joining drives poly(ADP-ribose) polymerase (PARP) inhibitor lethality in homologous recombination-deficient cells. Proc Natl Acad Sci U S A 2011;108:3406-11. [PubMed]
- Fong PC, Boss DS, Yap TA, et al. Inhibition of poly(ADP-ribose) polymerase in tumors from BRCA mutation carriers. N Engl J Med 2009;361:123-34. [PubMed]
- McLornan DP, List A, Mufti GJ. Applying synthetic lethality for the selective targeting of cancer. N Engl J Med 2014;371:1725-35. [PubMed]
- Pal T, Permuth-Wey J, Betts JA, et al. BRCA1 and BRCA2 mutations account for a large proportion of ovarian carcinoma cases. Cancer 2005;104:2807-16. [PubMed]
- Hennessy BT, Timms KM, Carey MS, et al. Somatic mutations in BRCA1 and BRCA2 could expand the number of patients that benefit from poly (ADP ribose) polymerase inhibitors in ovarian cancer. J Clin Oncol 2010;28:3570-6. [PubMed]
- Farmer H, McCabe N, Lord CJ, et al. Targeting the DNA repair defect in BRCA mutant cells as a therapeutic strategy. Nature 2005;434:917-21. [PubMed]
- Bryant HE, Schultz N, Thomas HD, et al. Specific killing of BRCA2-deficient tumours with inhibitors of poly(ADP-ribose) polymerase. Nature 2005;434:913-7. [PubMed]
- Evers B, Drost R, Schut E, et al. Selective inhibition of BRCA2-deficient mammary tumor cell growth by AZD2281 and cisplatin. Clin Cancer Res 2008;14:3916-25. [PubMed]
- Kelland LR, Tonkin KS. The effect of 3-aminobenzamide in the radiation response of three human cervix carcinoma xenografts. Radiother Oncol 1989;15:363-9. [PubMed]
- Calabrese CR, Almassy R, Barton S, et al. Anticancer chemosensitization and radiosensitization by the novel poly(ADP-ribose) polymerase-1 inhibitor AG14361. J Natl Cancer Inst 2004;96:56-67. [PubMed]
- Albert JM, Cao C, Kim KW, et al. Inhibition of poly(ADP-ribose) polymerase enhances cell death and improves tumor growth delay in irradiated lung cancer models. Clin Cancer Res 2007;13:3033-42. [PubMed]
- Khan K, Araki K, Wang D, et al. Head and neck cancer radiosensitization by the novel poly(ADP-ribose) polymerase inhibitor GPI-15427. Head Neck 2010;32:381-91. [PubMed]
- Russo AL, Kwon HC, Burgan WE, et al. In vitro and in vivo radiosensitization of glioblastoma cells by the poly (ADP-ribose) polymerase inhibitor E7016. Clin Cancer Res 2009;15:607-12. [PubMed]
- Donawho CK, Luo Y, Luo Y, et al. ABT-888, an orally active poly(ADP-ribose) polymerase inhibitor that potentiates DNA-damaging agents in preclinical tumor models. Clin Cancer Res 2007;13:2728-37. [PubMed]
- Penning TD, Zhu GD, Gandhi VB, et al. Discovery of the Poly(ADP-ribose) polymerase (PARP) inhibitor 2-[(R)-2-methylpyrrolidin-2-yl]-1H-benzimidazole-4-carboxamide (ABT-888) for the treatment of cancer. J Med Chem 2009;52:514-23. [PubMed]
- Muñoz-Gámez JA, Martín-Oliva D, Aguilar-Quesada R, et al. PARP inhibition sensitizes p53-deficient breast cancer cells to doxorubicin-induced apoptosis. Biochem J 2005;386:119-25. [PubMed]
- Zhang S, Royer R, Li S, et al. Frequencies of BRCA1 and BRCA2 mutations among 1,342 unselected patients with invasive ovarian cancer. Gynecol Oncol 2011;121:353-7. [PubMed]
- Baldwin RL, Nemeth E, Tran H, et al. BRCA1 promoter region hypermethylation in ovarian carcinoma: a population-based study. Cancer Res 2000;60:5329-33. [PubMed]
- Geisler JP, Hatterman-Zogg MA, Rathe JA, et al. Frequency of BRCA1 dysfunction in ovarian cancer. J Natl Cancer Inst 2002;94:61-7. [PubMed]
- Walsh T, Casadei S, Lee MK, et al. Mutations in 12 genes for inherited ovarian, fallopian tube, and peritoneal carcinoma identified by massively parallel sequencing. Proc Natl Acad Sci U S A 2011;108:18032-7. [PubMed]
- Taniguchi T, Tischkowitz M, Ameziane N, et al. Disruption of the Fanconi anemia-BRCA pathway in cisplatin-sensitive ovarian tumors. Nat Med 2003;9:568-74. [PubMed]
- Hughes-Davies L, Huntsman D, Ruas M, et al. EMSY links the BRCA2 pathway to sporadic breast and ovarian cancer. Cell 2003;115:523-35. [PubMed]
- Parikh RA, White JS, Huang X, et al. Loss of distal 11q is associated with DNA repair deficiency and reduced sensitivity to ionizing radiation in head and neck squamous cell carcinoma. Genes Chromosomes Cancer 2007;46:761-75. [PubMed]
- Cancer Genome Atlas Research Network. Integrated genomic analyses of ovarian carcinoma. Nature 2011;474:609-15. [PubMed]
- Gelmon KA, Tischkowitz M, Mackay H, et al. Olaparib in patients with recurrent high-grade serous or poorly differentiated ovarian carcinoma or triple-negative breast cancer: a phase 2, multicentre, open-label, non-randomised study. Lancet Oncol 2011;12:852-61. [PubMed]
- Audeh MW, Carmichael J, Penson RT, et al. Oral poly(ADP-ribose) polymerase inhibitor olaparib in patients with BRCA1 or BRCA2 mutations and recurrent ovarian cancer: a proof-of-concept trial. Lancet 2010;376:245-51. [PubMed]
- Kaye SB, Lubinski J, Matulonis U, et al. Phase II, open-label, randomized, multicenter study comparing the efficacy and safety of olaparib, a poly (ADP-ribose) polymerase inhibitor, and pegylated liposomal doxorubicin in patients with BRCA1 or BRCA2 mutations and recurrent ovarian cancer. J Clin Oncol 2012;30:372-9. [PubMed]
- Ledermann J, Harter P, Gourley C, et al. Olaparib maintenance therapy in platinum-sensitive relapsed ovarian cancer. N Engl J Med 2012;366:1382-92. [PubMed]
- Oza AM, Cibula D, Oaknin A, et al. Olaparib plus paclitaxel plus carboplatin (P/C) followed by olaparib maintenance treatment in patients (pts) with platinum-sensitive recurrent serous ovarian cancer (PSR SOC): A randomized, open-label phase II study. J Clin Oncol 2012;30:abstr 5001.
- Coleman R, Sill M, Aghajanian C. A phase II evaluation of the potent, highly selective PARP inhibitor veliparib in the treatment of persistent or recurrent epithelial ovarian, fallopian tube, or primary peritoneal cancer in patients who carry a germline BRCA1 or BRCA2 mutation. a Gynecologic Oncology Group study. Society of Gynecologic Oncology Annual Meeting on Women's Cancer. Los Angeles, CA, 2013.
- Kaufman B, Shapira-Frommer R, Schmutzler RK, et al. Olaparib Monotherapy in Patients With Advanced Cancer and a Germline BRCA1/2 Mutation. J Clin Oncol 2015;33:244-50. [PubMed]
- Kummar S, Fleming GF, Oza AM, et al. Randomized trial of oral cyclophosphamide and veliparib in high-grade serous ovarian, primary peritoneal, or fallopian tube cancers, or BRCA-mutant ovarian cancer. Clin Cancer Res 2015; [Epub ahead of print]. [PubMed]
- Fong PC, Yap TA, Boss DS, et al. Poly(ADP)-ribose polymerase inhibition: frequent durable responses in BRCA carrier ovarian cancer correlating with platinum-free interval. J Clin Oncol 2010;28:2512-9. [PubMed]
- Lord CJ, Ashworth A. Mechanisms of resistance to therapies targeting BRCA-mutant cancers. Nat Med 2013;19:1381-8. [PubMed]
- Ledermann J, Harter P, Gourley C, et al. Olaparib maintenance therapy in patients with platinum-sensitive relapsed serous ovarian cancer: a preplanned retrospective analysis of outcomes by BRCA status in a randomised phase 2 trial. Lancet Oncol 2014;15:852-61. [PubMed]
- Liu JF, Konstantinopoulos PA, Matulonis UA. PARP inhibitors in ovarian cancer: current status and future promise. Gynecol Oncol 2014;133:362-9. [PubMed]
- Balmaña J, Tung NM, Isakoff SJ, et al. Phase I, open-label study of olaparib plus cisplatin in patients with advanced solid tumors. J Clin Oncol 2012;30:abstr 1009.
- Rajan A, Carter CA, Kelly RJ, et al. A phase I combination study of olaparib with cisplatin and gemcitabine in adults with solid tumors. Clin Cancer Res 2012;18:2344-51. [PubMed]
- Lee JM, Hays JL, Annunziata CM, et al. Phase I/Ib study of olaparib and carboplatin in BRCA1 or BRCA2 mutation-associated breast or ovarian cancer with biomarker analyses. J Natl Cancer Inst 2014;106:dju089 [PubMed]
- Del Conte G, Sessa C, von Moos R, et al. Phase I study of olaparib in combination with liposomal doxorubicin in patients with advanced solid tumours. Br J Cancer 2014;111:651-9. [PubMed]
- Rajesh M, Mukhopadhyay P, Bátkai S, et al. Pharmacological inhibition of poly(ADP-ribose) polymerase inhibits angiogenesis. Biochem Biophys Res Commun 2006;350:352-7. [PubMed]
- Pyriochou A, Olah G, Deitch EA, et al. Inhibition of angiogenesis by the poly(ADP-ribose) polymerase inhibitor PJ-34. Int J Mol Med 2008;22:113-8. [PubMed]
- Tentori L, Lacal PM, Muzi A, et al. Poly(ADP-ribose) polymerase (PARP) inhibition or PARP-1 gene deletion reduces angiogenesis. Eur J Cancer 2007;43:2124-33. [PubMed]
- Liu JF, Barry WT, Birrer M, et al. Combination cediranib and olaparib versus olaparib alone for women with recurrent platinum-sensitive ovarian cancer: a randomised phase 2 study. Lancet Oncol 2014;15:1207-14. [PubMed]
- Dean E, Middleton MR, Pwint T, et al. Phase I study to assess the safety and tolerability of olaparib in combination with bevacizumab in patients with advanced solid tumours. Br J Cancer 2012;106:468-74. [PubMed]
- Juvekar A, Burga LN, Hu H, et al. Combining a PI3K inhibitor with a PARP inhibitor provides an effective therapy for BRCA1-related breast cancer. Cancer Discov 2012;2:1048-63. [PubMed]
- Ibrahim YH, García-García C, Serra V, et al. PI3K inhibition impairs BRCA1/2 expression and sensitizes BRCA-proficient triple-negative breast cancer to PARP inhibition. Cancer Discov 2012;2:1036-47. [PubMed]
- Kummar S, Chen A, Ji J, et al. Phase I study of PARP inhibitor ABT-888 in combination with topotecan in adults with refractory solid tumors and lymphomas. Cancer Res 2011;71:5626-34. [PubMed]
- Tan AR, Toppmeyer D, Stein MN, et al. Phase I trial of veliparib, (ABT-888), a poly(ADP-ribose) polymerase (PARP) inhibitor, in combination with doxorubicin and cyclophosphamide in breast cancer and other solid tumors. J Clin Oncol 2011;29:abstr 3041.
- Kummar S, Ji J, Morgan R, et al. A phase I study of veliparib in combination with metronomic cyclophosphamide in adults with refractory solid tumors and lymphomas. Clin Cancer Res 2012;18:1726-34. [PubMed]
- LoRusso P, Ji J, Li J, et al. Phase I study of the safety, pharmacokinetics and pharmacodynamics of the poly(ADP-ribose)polymerase (PARP) inhibitor veliparib (ABT-888) in combination with irinotecan (CPT-111) in patients with advanced solid tumors. J Clin Oncol 2011;29:abstr 3000.
- Reiss KA, Herman JM, Zahurak M, et al. A Phase I Study of Veliparib (ABT-888) in Combination with Low-Dose Fractionated Whole Abdominal Radiation Therapy in Patients with Advanced Solid Malignancies and Peritoneal Carcinomatosis. Clin Cancer Res 2015;21:68-76. [PubMed]
- Sandhu SK, Schelman WR, Wilding G, et al. The poly(ADP-ribose) polymerase inhibitor niraparib (MK4827) in BRCA mutation carriers and patients with sporadic cancer: a phase 1 dose-escalation trial. Lancet Oncol 2013;14:882-92. [PubMed]
- Ihnen M, zu Eulenburg C, Kolarova T, et al. Therapeutic potential of the poly(ADP-ribose) polymerase inhibitor rucaparib for the treatment of sporadic human ovarian cancer. Mol Cancer Ther 2013;12:1002-15. [PubMed]
- Plummer R, Jones C, Middleton M, et al. Phase I study of the poly(ADP-ribose) polymerase inhibitor, AG014699, in combination with temozolomide in patients with advanced solid tumors. Clin Cancer Res 2008;14:7917-23. [PubMed]
- Coupe N, Roxburgh P, Drew Y, et al. Phase I study of oral or intravenous rucaparib in combination with platinum-containing chemotherapeutic regimens: analysis of patients with ovarian or peritoneal cancers. Eur Soc of Gyn Onc Proceedings 2013:abstr 155.
- Roxburgh P, Molife LR, Gupta A, et al. phase 1 study of oral rucaparib in combination with carboplatin. Proceedings from the European Cancer Congress 2013:abstr 824.
- Drew Y, Ledermann JA, Jones A, et al. Phase II trial of the poly(ADP-ribose) polymerase (PARP) inhibitor AG-014699 in BRCA 1 and 2-mutated, advanced ovarian and/or locally advanced or metastatic breast cancer. J Clin Oncol 2011;29:abstr 3104.
- De Bono JS, Mina LA, Gonzalez M, et al. First-in-human trial of the novel PARP inhibitor BMN 673 in patients with solid tumors. J Clin Oncol 2013;31:abstr 2580.
- O'Shaughnessy J, Osborne C, Pippen JE, et al. Iniparib plus chemotherapy in metastatic triple-negative breast cancer. N Engl J Med 2011;364:205-14. [PubMed]
- O'Shaughnessy J, Schwartzberg L, Danso MA, et al. Phase III study of iniparib plus gemcitabine and carboplatin versus gemcitabine and carboplatin in patients with metastatic triple-negative breast cancer. J Clin Oncol 2014;32:3840-7. [PubMed]
- Patel AG, De Lorenzo SB, Flatten KS, et al. Failure of iniparib to inhibit poly(ADP-Ribose) polymerase in vitro. Clin Cancer Res 2012;18:1655-62. [PubMed]
- Chuang HC, Kapuriya N, Kulp SK, et al. Differential anti-proliferative activities of poly(ADP-ribose) polymerase (PARP) inhibitors in triple-negative breast cancer cells. Breast Cancer Res Treat 2012;134:649-59. [PubMed]
- Mateo J, Ong M, Tan DS, et al. Appraising iniparib, the PARP inhibitor that never was--what must we learn? Nat Rev Clin Oncol 2013;10:688-96. [PubMed]
- Gilabert M, Launay S, Ginestier C, et al. Poly(ADP-ribose) polymerase 1 (PARP1) overexpression in human breast cancer stem cells and resistance to olaparib. PLoS One 2014;9:e104302 [PubMed]
- Jaspers JE, Kersbergen A, Boon U, et al. Loss of 53BP1 causes PARP inhibitor resistance in Brca1-mutated mouse mammary tumors. Cancer Discov 2013;3:68-81. [PubMed]
- Murai J, Huang SY, Das BB, et al. Trapping of PARP1 and PARP2 by Clinical PARP Inhibitors. Cancer Res 2012;72:5588-99. [PubMed]
- Veuger SJ, Curtin NJ, Richardson CJ, et al. Radiosensitization and DNA repair inhibition by the combined use of novel inhibitors of DNA-dependent protein kinase and poly(ADP-ribose) polymerase-1. Cancer Res 2003;63:6008-15. [PubMed]
- de Murcia JM, Niedergang C, Trucco C, et al. Requirement of poly(ADP-ribose) polymerase in recovery from DNA damage in mice and in cells. Proc Natl Acad Sci U S A 1997;94:7303-7. [PubMed]
- Masutani M, Nozaki T, Nishiyama E, et al. Function of poly(ADP-ribose) polymerase in response to DNA damage: gene-disruption study in mice. Mol Cell Biochem 1999;193:149-52. [PubMed]
- Duan W, Gao L, Zhao W, et al. Assessment of FANCD2 nuclear foci formation in paraffin-embedded tumors: a potential patient-enrichment strategy for treatment with DNA interstrand crosslinking agents. Transl Res 2013;161:156-64. [PubMed]
- de Winter JP. The Fanconi anemia protein FANCF forms a nuclear complex with FANCA, FANCC and FANCG. Hum Mol Genet 2000;9:2665-74. [PubMed]
- Yao C, Du W, Chen H, et al. The Fanconi anemia/BRCA pathway is involved in DNA interstrand cross-link repair of adriamycin-resistant leukemia cells. Leuk Lymphoma 2014;1-8. [Epub ahead of print]. [PubMed]
- Mukhopadhyay A, Elattar A, Cerbinskaite A, et al. Development of a functional assay for homologous recombination status in primary cultures of epithelial ovarian tumor and correlation with sensitivity to poly(ADP-ribose) polymerase inhibitors. Clin Cancer Res 2010;16:2344-51. [PubMed]
- Mukhopadhyay A, Plummer ER, Elattar A, et al. Clinicopathological features of homologous recombination-deficient epithelial ovarian cancers: sensitivity to PARP inhibitors, platinum, and survival. Cancer Res 2012;72:5675-82. [PubMed]
- Pennington KP, Swisher EM. Hereditary ovarian cancer: beyond the usual suspects. Gynecol Oncol 2012;124:347-53. [PubMed]
- Reinbolt RE, Hays JL. The Role of PARP Inhibitors in the Treatment of Gynecologic Malignancies. Front Oncol 2013;3:237. [PubMed]