Targeting the PI3K/AKT/mTOR pathway in ovarian cancer
Introduction
Ovarian cancer is the fifth most common cause of cancer related death in women despite its rare incidence (1). Recent evidence suggests that what is understood as ovarian cancer is actually a spectrum of cancer types possibly originating from the fallopian tubes, ovaries and/or the peritoneal lining (2-4). Ovarian cancer is a heterogeneous disease comprised of different histologic subtypes of adenocarcinomas including serous, mucinous, endometrioid, clear cell and transitional type carcinomas (which closely resemble serous carcinomas) (5). Not only are these subtypes distinct microscopically, but they also carry unique molecular changes, express different markers and not surprisingly, respond differently to therapy. The recent effort made public in The Cancer Genome Atlas (TCGA) highlights the complex genomic architecture of the most common histologic subtype of ovarian cancer, the high grade serous carcinomas. The project identifies numerous activating mutations, DNA copy number alterations and inactivating mutations further characterizing this single histologic entity into four additional molecular subtypes with differing prognoses (6). The complexity of ovarian cancer suggests that there will never be a single targeted therapy that will address this disease in a broad sense, but instead, with advances in personalized medicine, targeted therapy will need to be matched to the specific vulnerabilities of a particular tumor. The introduction of novel small molecule phosphoinositol-3 kinase/protein kinase B/mammalian target of rapamycin (PI3K/AKT/mTOR) pathway inhibitors to the anticancer armamentarium coupled with rational clinical trial design may alter the landscape of treatment for ovarian cancer in the near future. In this study we review the existing avenues to promote this change.
Overview of the pathway
The PI3K/AKT/mTOR pathway is a central regulator of normal cell physiology. Its main function is to integrate extracellular growth signals (including insulin, growth factors and amino acids) into an intracellular cascade culminating in increased cellular metabolism, growth and proliferation. This pathway is frequently altered in ovarian cancer, where it plays an important role in cancer cell proliferation, growth, migration, invasion and evasion of apoptosis (7-9). Data from the TCGA in high grade serous ovarian cancers suggests it is altered in up to 34% of samples analyzed, however, if the search is broadened to include downstream mTOR targets, the pathway seems to be altered in up to 63% of cases (6,10). The pathway is also particularly important for the rare subtypes of clear cell and endometrioid ovarian cancer, where it may offer a unique approach to circumvent chemotherapy resistance in these tumors (11-15).
Numerous molecular and somatic alterations in the PI3K/AKT/mTOR pathway have been documented in ovarian cancer cell lines and tumor specimens (Table 1). Figure 1 describes the frequency of molecular alterations in this pathway as per the cBioPortal of the TCGA including 580 ovarian cancer specimens (10). Despite the heterogeneity of the alterations seen, the overarching theme both in the preclinical data and in the TCGA data is that ovarian cancer relies on hyperactivated mTOR and is a formidable model to study these novel targeted therapies.
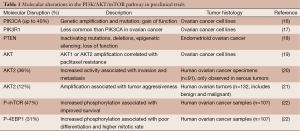
Full table
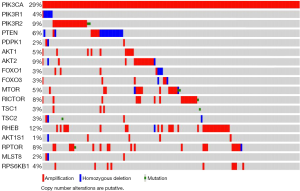
The mTOR kinase itself lies at the crossroads of several important oncogenic pathways in the cell, including the PI3K/AKT pathway and also the mitogen-activated protein kinase/extracellular signal-regulated kinases (MAPK/ERK) pathway, another important integrator of extracellular signals into protein transcription and translation. mTOR is an integral part of two complexes in the cell. Phosphorylation of mTOR complex 1 regulates messenger ribonucleic acid (mRNA) translation and oncogenic protein synthesis in the cell (23). Mounting data from our group and others suggest that hyperactivation of mTOR leads to selective translation of proteins responsible for survival factors (survivin, Mcl1, XIAP), angiogenesis (VEGF-A, FGF2) and the DNA repair response (BRCA1, 53BP1, γH2AX and others) by an increase in eIF4G1 mediated cap-dependent translation (24). mTOR complex 2 is less well understood, but plays an important role in the phosphorylation of AKT (25), a promiscuous oncogenic kinase that activates a plethora of proliferative signaling in the cell. Allosteric mTOR inhibitors such as sirolimus (rapamycin) analogs are capable of inhibiting mTOR complex 1 (25). However, without inhibition of mTOR complex 2, mTOR is available to phosphorylate AKT and the overwhelming proliferative signal may partially explain the therapeutic inefficacy of these drugs in human cancers.
Pathway inhibitors
mTOR inhibitors
There are two broad categories of available mTOR inhibitors: the allosteric inhibitors, which are derivatives of rapamycin and commonly referred to as “rapalogs”, and the novel small molecule mTOR inhibitors.
Rapamycin analogs
Rapamycin (sirolimus) was first discovered in a soil sample from the island of Rapa Nui in the 1970s. It is a product of the bacterium Streptomyces hygroscopicus and a macrolide antibiotic with antifungal and immunosuppressive properties (Figure 2) (26). The antitumoral effects were first noted in renal transplant patients who received mTOR inhibitors in clinical trials and had a lower incidence of tumors (27-29). Rapamycin binds to FK binding protein 12 (FKBP12) and the FKBP-rapamycin binding domain of the mTOR kinase forming a large ternary complex that is presumed to perturb protein function (30). The complex interferes primarily with mTOR complex 1 and only inhibits complex 2 in high doses associated with increased toxicity (9).
This class of drugs is comprised of three compounds with oral formulations: sirolimus (rapamune, Pfizer, formerly Wyeth, New York, NY, USA), everolimus (Affinitor, RAD 001, Novartis, Basel, Switzerland) and ridaforolimus (AP23573 or MK-8669, Merck and ARIAD Pharmaceuticals, New Jersey, NJ, USA); and one with a parenteral formulation, temsirolimus (Torisel, CCI779, Pfizer, New York, NY, USA). Class based toxicities include stomatitis, wound-healing problems and thrombocytopenia. Biomarkers of activity include decreased phosphorylation of ribosomal protein S6, which is associated with an antiproliferative effect and arrest at the G1 phase of the cell cycle, and to a lesser extent, 4EBP1.
Temsirolimus was investigated as a single agent in a Gynecologic Oncology Group (GOG) phase II trial for recurrent ovarian cancer with a reported an overall response rate (ORR) of 9.3% [five partial responses (PRs) in 54 evaluable patients], 6 months progression-free survival (PFS) rate of 24.1% and median PFS and overall survival (OS) of 3.2 and 11.6 months, respectively with no serious adverse events (SAEs) (NCT00429793) (31). The investigators concluded that the modest activity was insufficient to warrant further evaluation of temsirolimus in a phase III trial in an unselected population.
Temsirolimus was also evaluated in combination with topotecan in a phase I study of patients with advanced or recurrent gynecologic malignancies (NCT00523432). A starting dose of 1 mg/m2 of intravenous topotecan days 1, 8 and 15 were combined with 25 mg temsirolimus days 1, 8, 15 and 22 of a 28-day cycle. Seven patients with ovarian cancer entered this trial along with patients with uterine carcinosarcomas, endometrial and cervical cancers. Dose-limiting toxicity (DLT) in this cohort included asymptomatic neutropenia and thrombocytopenia. Four patients in this cohort had no prior pelvic radiation therapy (RT) and were successfully treated with 1 mg/m2 topotecan with 25 mg temsirolimus, days 1, 8, and 15 of a 28-day cycle. The combination was not tolerated in patients with a history of pelvic RT. Nine of eleven patients were found to have stable disease (SD) at 8 weeks. One woman with recurrent clear cell carcinoma of the ovary experienced SD for seven cycles of treatment (28 weeks). She was ultimately removed from the study due to disease progression (32).
Also in a phase I setting, the combination of bevacizumab, a monoclonal VEGF-A antibody, and temsirolimus showed promising results and attenuation of hypoxia-inducible factor 1 alpha (HIF-1α) levels. HIF-1α is part of the cellular response that permits growth in low oxygen concentrations including but not limited to angiogenesis, a crucial oncogenic pathway in ovarian cancer. Forty one patients with recurrent gynecologic cancers and a median of four prior systemic therapies were treated with bevacizumab 15 mg/kg IV every 3 weeks and temsirolimus 25 mg IV weekly without any DLTs. Grade 3 or 4 treatment-related toxicities included: thrombocytopenia (10%), mucositis (2%), hypertension (2%), hypercholesterolemia (2%), fatigue (7%), elevated aspartate aminotransferase (2%), and neutropenia (2%). Twenty-nine patients (71%) experienced no treatment-related toxicity greater than grade 2. Eight patients (20%) achieved SD >6 months and seven patients (17%) had a PR (total =15/41 patients (37%)). Eight of 13 patients (62%) with high-grade serous histology (ovarian or primary peritoneal) achieved more than 6 months of SD or a partial response (33).
Moroney et al. recently reported a SD ≥6 months/PR/CR rate of 38% in patients with advanced gynecologic and breast malignancies treated with liposomal doxorubicin, bevacizumab and temsirolimus (34). The patients were heavily pretreated with a median of four prior systemic therapies. Only a randomized study would be able to answer the question as to whether or not liposomal doxorubicin adds activity to the bevacizumab and temsirolimus regimen.
The combination of bevacizumab and temsirolimus was subsequently studied in a phase II study of women with recurrent ovarian cancer (up to two prior lines of chemotherapy). This study showed three PRs and 14 patients progression free at 6 months among the first 25 enrolled patients and was expanded into a second stagem which is ongoing (35).
Everolimus is being evaluated in a phase I trial in combination with carboplatin and liposomal doxorubicin in relapsed ovarian cancer (NCT01281514) (36). Additionally, in the phase II setting, the compound is being evaluated in combination with bevacizumab for patients with recurrent or persistent ovarian cancer (NCT00886691, NCT01031381) (37,38). Table 2 summarizes the registered clinical trials of mTOR complex 1 inhibitors for ovarian cancer.
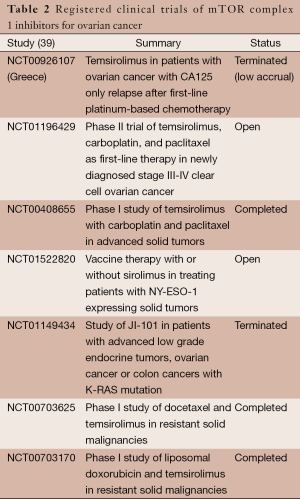
Full table
Dual mTOR inhibitors
Dual mTOR inhibitors are adenosine-5'-triphosphate (ATP)-competitive mTOR kinase inhibitors. As they compete with ATP in the catalytic site of the mTOR kinase itself, they inhibit both mTOR complex 1 and 2. mTOR complex 2 lies upstream from complex 1 and is responsible for the phosphorylation of AKT in a feedback activation loop presumably responsible for the relative ineffectiveness of complex 1 inhibitors in clinical trials (25). Preclinical data with these novel inhibitors demonstrate that similarly to rapalogs, they have antiproliferative effects in several human cancers (9,40-43). The mechanism of action is presumably via a decrease in protein translation, inhibition of cell cycle progression and an antiangiogenic effect. Interestingly, the dual inhibitors seem to be more effective complex 1 inhibitors, with near complete inhibition of AKT and 4EBP1 phosphorylation at much lower doses than achievable with rapalogs alone (9,43,44).
There are several dual mTOR kinase inhibitors currently undergoing development and a few in clinical trials. PP242 is the prototypical drug used in preclinical research and MLN 128 (Millenium Therapeutics, formerly Takeda) is a reformulation of PP242 with improved bioavailability. Other compounds include CC115 and CC223 (Celgene), as well as AZD8055, and AZD2014 (AstraZeneca).
A phase I trial of AZD8055 in solid tumors and lymphoma (n=49) demonstrated safety and tolerability of the drug. The most frequent adverse events assessed to be related to AZD8055 were increased alanine aminotransferase (22%), increased aspartate aminotransferase (22%) and fatigue (16%). Seven patients had SD for ≥4 months. Partial metabolic responses, assessed by fluorodeoxyglucose (FDG) positron emission tomography (PET), were observed at ≥40 mg BID (n=8 at day 35) but no Response Evaluation Criteria In Solid Tumors (RECIST) responses were seen in this unselected population (45). The results of the expanded phase I trial of CC-223 presented at American Society of Clinical Oncology (ASCO) 2013 meeting also confirm these findings. One hundred and one solid tumor subjects were treated (no gynecologic cancers enrolled). The most common related adverse events were fatigue, rash, stomatitis, hyperglycemia, anorexia, nausea, vomiting and diarrhea. Serious AEs included infection [1], pneumonitis [4], renal insufficiency [2] and pancreatitis [2]. CC-223 dose reduction was required in >50% of the subjects with non-small cell lung cancer (NSCLC) and hepatocellular carcinoma (HCC). Exposure-dependent TORC1 (p4EBP1) and TORC2 (pAKT) inhibition was observed across all cohorts. CC-223 was present in all resected glioblastoma multiforme tumors with plasma: tumor ratios of 16-77% confirming transit across the blood-brain barrier. Reduction in glucose uptake [>25% decrease in Standardized Uptake Value (SUV)] on PET imaging at day 15 was observed in 78% (14/18) of NSCLC and 69% (11/16) of HCC subjects. Disease control rate in the overall NSCLC cohort was 42% (11/26) and in the HCC cohort, 40% (10/25) (46).
The current focus of development appears to be in NSCLC, prostate cancer, glioblastoma, breast and hematologic malignancies. The opportunity for enrollment in gynecologic malignancies seems to be through phase I studies aimed at metastatic solid tumors at this time, but hopefully this will change as these drugs move into phase II development. As shown in Table 3, strategies to pair mTOR inhibitors with anti-angiogenic compounds, PI3K inhibitors and cytotoxic chemotherapy are being taken into clinical trials based on favorable preclinical research findings.
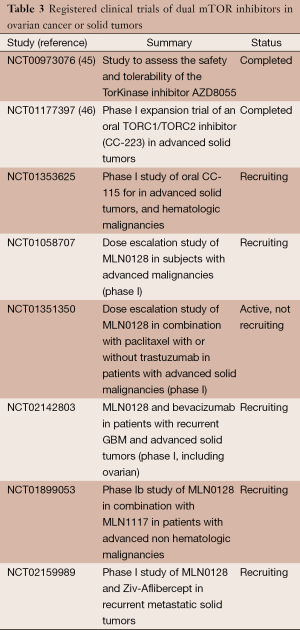
Full table
PI3K and PI3K/mTOR inhibitors
Not surprisingly, PI3K inhibitors have activity in preclinical models of ovarian cancer (47). Similarly to dual mTOR inhibitors, the new generations of PI3K inhibitors compete for ATP in catalytic domain of the protein kinase. As a consequence, some of these drugs are not specific for PI3K and block multiple kinases including mTOR and AKT (48). Because of these off target effects, the early development of PI3K inhibitors was negatively affected by high toxicity, which has been overcome by improved drug design and specificity.
PI3K inhibitors are broadly classified into three groups based on the selectivity to PI3K isoforms and mTOR: the pan-PI3K isoform inhibitors (e.g., XL147, BKM120, GDC-0941, BAY 80-9646, PX-866); the dual PI3K and mTOR inhibitors (e.g., XL765, BEZ235, GSK2126458, GDC-0980, SF-1126, PF-04691502, PF-05212384, BGT-226); and isoform-specific inhibitors (e.g., p110α-specific inhibitors, BYL719, INK-1114, GDC-0032) (Table 4).
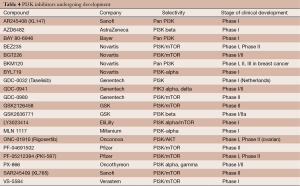
Full table
Recent data from phase I clinical trials suggest similar tolerability to other compounds in the class. For instance, in a phase I study of BAY 80-9646 in an expansion cohort of 23 patients with solid tumors, common grade 2-3 adverse events included hyperglycemia requiring insulin therapy, hypertension and interstitial pneumonitis (49). Table 5 reviews the ongoing clinical trials of PI3K inhibitors in ovarian cancer. Several compounds are being combined with MEK inhibitors in clinical trials and aimed at specific patient populations with pathway relevant mutations (preclinical data suggests PIK3CA mutations and phosphatase and tensin homolog (PTEN) inactivating mutations are seen with frequency in low grade tumors, so a strategy to block the MAPK pathway along with PI3K should achieve highest therapeutic activity in these tumors) (50).
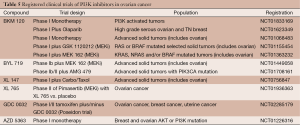
Full table
AKT inhibitors
AKT is an important driver of tumor regulation in ovarian cancer. It is a known oncogene encoding for a highly active protein kinase. Constitutive activation of AKT is achieved by PTEN deletion (an important regulator of AKT) and also through a hyperactive mTOR signaling cascade (mTOR complex 2 can also phosphorylate AKT). In turn, the phosphorylated AKT cross talks to several pathways and in turn activates a myriad of downstream targets responsible for cell growth and proliferation. AKT exists in three isoforms. Inhibitors may be specific to a particular isoform or active across the entire spectrum. Table 6 outlines the most important AKT inhibitors available.
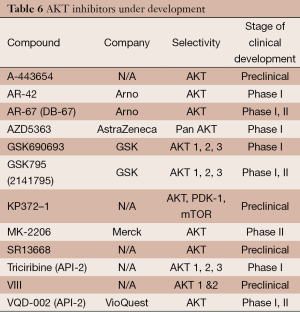
Full table
Preclinical data in ovarian cancer suggests AKT inhibition reverses chemotherapy resistance to cisplatin and has activity in this disease (51). These findings are confirmed by a phase I clinical trial of the AKT inhibitor, GSK795, in 12 patients with platinum resistant ovarian cancer. In this study, patients with Platinum Resistant ovarian cancer received 25, 50 or 75 mg of oral GSK795 daily. Paired tumor biopsies were compared to dynamic FDG-PET scans at 2 and 4 weeks post-treatment. The most common drug related AEs were decreased appetite (18%), and vomiting (18%), both grade 1 and 2. Eight out of the 12 patients had SD at week 4 by RECIST (4 had progressive disease). Clinical activity was seen in two patients with evidence of tumor regression by RECIST and decreased CA 125. Overall tumor FDG metabolism decreased in 71% of tumors with treatment, although inter and intra-patient variability was observed. There was no temporal or dose-response effect in FDG uptake (52). Table 7 outlines the ongoing clinical trials in ovarian cancer.
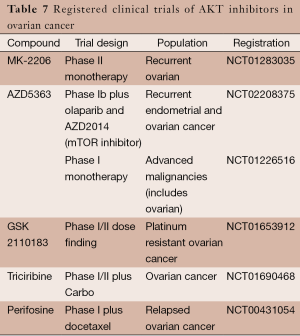
Full table
Conclusions
There is strong preclinical rationale to support the continued development of PI3K/AKT/mTOR inhibitors in ovarian cancer. This paper has thoroughly described the landscape of completed and ongoing clinical trials that are likely to improve our understanding of the role of this pathway in the treatment of ovarian cancer. Combination of mTOR inhibitors with cytotoxic chemotherapy and other biologic agents such as anti-angiogenic compounds, may capitalize on the central role of this pathway in regulating protein translation, cell growth, migration, metastasis and angiogenesis.
Rational clinical trial design with a focus in identifying a patient population most likely to benefit from this strategy is imperative to the success of this therapeutic strategy. There is some preclinical data suggesting this pathway is implicated in the reversal of platinum resistance in ovarian cancer, suggestive of an ideal patient population to study.
Further research into biomarkers that predict pathway activation (such as PIK3CA activating mutations, hyperactivated mTOR or AKT) is needed for rational patient recruitment. Improved biomarkers of drug activity are also needed for monitoring of drug activity in clinical trials (FDG avidity is variable and has not been reliable in the few phase I studies reported).
It is with great excitement that we anticipate the results of the ongoing trials of PI3K/AKT/mTOR inhibitors in ovarian cancer and that we look forward to offering our patients better and smarter options in the treatment of this disease that continues to take so many cherished lives.
Acknowledgments
F. Musa would like to thank Franco Muggia, Robert Schneider and the division of Gynecologic Oncology at NYU for allowing her the opportunity to perform ovarian cancer research. We also deeply acknowledge the effort and motivation of our patients with ovarian cancer who have bravely succumbed to this disease despite their very best efforts.
Funding: None.
Footnote
Provenance and Peer Review: This article was commissioned by the Guest Editors (Franco Muggia and Eleonora Teplinsky) for the series “Epithelial Ovarian Cancer Treatment: Integrating Molecular Targeting” published in Translational Cancer Research. The article has undergone external peer review.
Conflicts of Interest: Both authors have completed the ICMJE uniform disclosure form (available at http://dx.doi.org/10.3978/j.issn.2218-676X.2015.01.10). The series “Epithelial Ovarian Cancer Treatment: Integrating Molecular Targeting” was commissioned by the editorial office without any funding or sponsorship. The authors have no other conflicts of interest to declare.
Ethical Statement: The authors are accountable for all aspects of the work in ensuring that questions related to the accuracy or integrity of any part of the work are appropriately investigated and resolved.
Open Access Statement: This is an Open Access article distributed in accordance with the Creative Commons Attribution-NonCommercial-NoDerivs 4.0 International License (CC BY-NC-ND 4.0), which permits the non-commercial replication and distribution of the article with the strict proviso that no changes or edits are made and the original work is properly cited (including links to both the formal publication through the relevant DOI and the license). See: https://creativecommons.org/licenses/by-nc-nd/4.0/.
References
- Siegel R, Naishadham D, Jemal A. Cancer statistics, 2013. CA Cancer J Clin 2013;63:11-30. [PubMed]
- Erickson BK, Conner MG, Landen CN Jr. The role of the fallopian tube in the origin of ovarian cancer. Am J Obstet Gynecol 2013;209:409-14. [PubMed]
- Kim J, Coffey DM, Creighton CJ, et al. High-grade serous ovarian cancer arises from fallopian tube in a mouse model. Proc Natl Acad Sci U S A 2012;109:3921-6. [PubMed]
- Dubeau L. The cell of origin of ovarian epithelial tumours. Lancet Oncol 2008;9:1191-7. [PubMed]
- Soslow RA. Histologic subtypes of ovarian carcinoma: an overview. Int J Gynecol Pathol 2008;27:161-74. [PubMed]
- Cancer Genome Atlas Research Network. Integrated genomic analyses of ovarian carcinoma. Nature 2011;474:609-15. [PubMed]
- Vivanco I, Sawyers CL. The phosphatidylinositol 3-Kinase AKT pathway in human cancer. Nat Rev Cancer 2002;2:489-501. [PubMed]
- Altomare DA, Wang HQ, Skele KL, et al. AKT and mTOR phosphorylation is frequently detected in ovarian cancer and can be targeted to disrupt ovarian tumor cell growth. Oncogene 2004;23:5853-7. [PubMed]
- Musa F, Alard A, David-West G, et al. Abstract 4525: mTOR complex inhibition as a novel therapeutic strategy in high-grade papillary serous ovarian cancer. In: Proceedings of the 105th Annual Meeting of the American Association for Cancer Research; 2014 Apr 5-9; San Diego, CA. Philadelphia (PA): AACR; Cancer Res 2014;74:Abstract nr 4525.
- Cerami E, Gao J, Dogrusoz U, et al. The cBio cancer genomics portal: an open platform for exploring multidimensional cancer genomics data. Cancer Discov 2012;2:401-4. [PubMed]
- Kolasa IK, Rembiszewska A, Felisiak A, et al. PIK3CA amplification associates with resistance to chemotherapy in ovarian cancer patients. Cancer Biol Ther 2009;8:21-6. [PubMed]
- Romero I, Bast RC Jr. Minireview: human ovarian cancer: biology, current management, and paths to personalizing therapy. Endocrinology 2012;153:1593-602. [PubMed]
- Oishi T, Itamochi H, Kudoh A, et al. The PI3K/mTOR dual inhibitor NVP-BEZ235 reduces the growth of ovarian clear cell carcinoma. Oncol Rep 2014;32:553-8. [PubMed]
- Hirasawa T, Miyazawa M, Yasuda M, et al. Alterations of hypoxia-induced factor signaling pathway due to mammalian target of rapamycin (mTOR) suppression in ovarian clear cell adenocarcinoma: in vivo and in vitro explorations for clinical trial. Int J Gynecol Cancer 2013;23:1210-8. [PubMed]
- Miyazawa M, Yasuda M, Fujita M, et al. Therapeutic strategy targeting the mTOR-HIF-1alpha-VEGF pathway in ovarian clear cell adenocarcinoma. Pathol Int 2009;59:19-27. [PubMed]
- Shayesteh L, Lu Y, Kuo WL, et al. PIK3CA is implicated as an oncogene in ovarian cancer. Nat Genet 1999;21:99-102. [PubMed]
- Philp AJ, Campbell IG, Leet C, et al. The phosphatidylinositol 3'-kinase p85alpha gene is an oncogene in human ovarian and colon tumors. Cancer Res 2001;61:7426-9. [PubMed]
- Obata K, Morland SJ, Watson RH, et al. Frequent PTEN/MMAC mutations in endometrioid but not serous or mucinous epithelial ovarian tumors. Cancer Res 1998;58:2095-7. [PubMed]
- Page C, Lin HJ, Jin Y, et al. Overexpression of Akt/AKT can modulate chemotherapy-induced apoptosis. Anticancer Res 2000;20:407-16. [PubMed]
- Yuan ZQ, Sun M, Feldman RI, et al. Frequent activation of AKT2 and induction of apoptosis by inhibition of phosphoinositide-3-OH kinase/Akt pathway in human ovarian cancer. Oncogene 2000;19:2324-30. [PubMed]
- Bellacosa A, de Feo D, Godwin AK, et al. Molecular alterations of the AKT2 oncogene in ovarian and breast carcinomas. Int J Cancer 1995;64:280-5. [PubMed]
- Noske A, Lindenberg JL, Darb-Esfahani S, et al. Activation of mTOR in a subgroup of ovarian carcinomas: correlation with p-eIF-4E and prognosis. Oncol Rep 2008;20:1409-17. [PubMed]
- Silvera D, Formenti SC, Schneider RJ. Translational control in cancer. Nat Rev Cancer 2010;10:254-66. [PubMed]
- Badura M, Braunstein S, Zavadil J, et al. DNA damage and eIF4G1 in breast cancer cells reprogram translation for survival and DNA repair mRNAs. Proc Natl Acad Sci U S A 2012;109:18767-72. [PubMed]
- Sarbassov DD, Guertin DA, Ali SM, et al. Phosphorylation and regulation of Akt/PKB by the rictor-mTOR complex. Science 2005;307:1098-101. [PubMed]
- Vézina C, Kudelski A, Sehgal SN. Rapamycin (AY-22,989), a new antifungal antibiotic. I. Taxonomy of the producing streptomycete and isolation of the active principle. J Antibiot (Tokyo) 1975;28:721-6. [PubMed]
- Kahan BD, Yakupoglu YK, Schoenberg L, et al. Low incidence of malignancy among sirolimus/cyclosporine-treated renal transplant recipients. Transplantation 2005;80:749-58. [PubMed]
- Mathew T, Kreis H, Friend P. Two-year incidence of malignancy in sirolimus-treated renal transplant recipients: results from five multicenter studies. Clin Transplant 2004;18:446-9. [PubMed]
- Luan FL, Hojo M, Maluccio M, et al. Rapamycin blocks tumor progression: unlinking immunosuppression from antitumor efficacy. Transplantation 2002;73:1565-72. [PubMed]
- Edwards SR, Wandless TJ. The rapamycin-binding domain of the protein kinase mammalian target of rapamycin is a destabilizing domain. J Biol Chem 2007;282:13395-401. [PubMed]
- Behbakht K, Sill MW, Darcy KM, et al. Phase II trial of the mTOR inhibitor, temsirolimus and evaluation of circulating tumor cells and tumor biomarkers in persistent and recurrent epithelial ovarian and primary peritoneal malignancies: a Gynecologic Oncology Group study. Gynecol Oncol 2011;123:19-26. [PubMed]
- Temkin SM, Yamada SD, Fleming GF. A phase I study of weekly temsirolimus and topotecan in the treatment of advanced and/or recurrent gynecologic malignancies. Gynecol Oncol 2010;117:473-6. [PubMed]
- Piha-Paul SA, Wheler JJ, Fu S, et al. Advanced gynecologic malignancies treated with a combination of the VEGF inhibitor bevacizumab and the mTOR inhibitor temsirolimus. Oncotarget 2014;5:1846-55. [PubMed]
- Moroney JW, Schlumbrecht MP, Helgason T, et al. A phase I trial of liposomal doxorubicin, bevacizumab, and temsirolimus in patients with advanced gynecologic and breast malignancies. Clin Cancer Res 2011;17:6840-6. [PubMed]
- Morgan R, Oza AM, Qin R, et al. A phase II trial of temsirolimus and bevacizumab in patients with endometrial, ovarian, hepatocellular carcinoma, carcinoid, or islet cell cancer: Ovarian cancer (OC) subset—A study of the Princess Margaret, Mayo, Southeast phase II, and California Cancer (CCCP) N01 Consortia NCI#8233. J Clin Oncol 2011;29:abstr 5015.
- Martin LP. Carboplatin, Pegylated Liposomal Doxorubicin Hydrochloride, and Everolimus in Treating Patients With Relapsed Ovarian Epithelial, Fallopian Tube, or Peritoneal Cavity Cancer. Ongoing Clinical Trial at Fox Chase Cancer Center.
- Tew WP. Bevacizumab With or Without Everolimus in Treating Patients With Recurrent or Persistent Ovarian Epithelial Cancer, Fallopian Tube Cancer, or Primary Peritoneal Cancer. ngoing Clinical Trial at Memorial Sloan Kettering Cancer Center.
- Edwards R. Study of RAD001 and Bevacizumab in Recurrent Ovarian, Peritoneal, and Fallopian Tube Cancer (RADBEV). Ongoing Clinical Trial at University of Pittsburgh.
. Available online: http://www.clinicaltrials.govClinical Trials Website - Xing X, Zhang L, Wen X, et al. PP242 suppresses cell proliferation, metastasis, and angiogenesis of gastric cancer through inhibition of the PI3K/AKT/mTOR pathway. Anticancer Drugs 2014;25:1129-40. [PubMed]
- Ono A, Oike R, Okuhashi Y, et al. Comparative effects of PP242 and rapamycin on mTOR signalling and NOTCH signalling in leukemia cells. Anticancer Res 2013;33:809-13. [PubMed]
- Hoang B, Benavides A, Shi Y, et al. The PP242 mammalian target of rapamycin (mTOR) inhibitor activates extracellular signal-regulated kinase (ERK) in multiple myeloma cells via a target of rapamycin complex 1 (TORC1)/eukaryotic translation initiation factor 4E (eIF-4E)/RAF pathway and activation is a mechanism of resistance. J Biol Chem 2012;287:21796-805. [PubMed]
- Korets SB, Musa F, Curtin J, et al. Dual mTORC1/2 inhibition in a preclinical xenograft tumor model of endometrial cancer. Gynecol Oncol 2014;132:468-73. [PubMed]
- Vilar E, Perez-Garcia J, Tabernero J. Pushing the envelope in the mTOR pathway: the second generation of inhibitors. Mol Cancer Ther 2011;10:395-403. [PubMed]
- Naing A, Aghajanian C, Raymond E, et al. Safety, tolerability, pharmacokinetics and pharmacodynamics of AZD8055 in advanced solid tumours and lymphoma. Br J Cancer 2012;107:1093-9. [PubMed]
- Varga A, Mita MM, Wu JJ, et al. Phase I expansion trial of an oral TORC1/TORC2 inhibitor (CC-223) in advanced solid tumors. J Clin Oncol 2013;31:abstr 2606.
- Ihle NT, Williams R, Chow S, et al. Molecular pharmacology and antitumor activity of PX-866, a novel inhibitor of phosphoinositide-3-kinase signaling. Mol Cancer Ther 2004;3:763-72. [PubMed]
- Yuan TL, Cantley LC. PI3K pathway alterations in cancer: variations on a theme. Oncogene 2008;27:5497-510. [PubMed]
- Lotze MT, Appleman JL, Ramanathan RK, et al. Phase I study of intravenous PI3K inhibitor BAY 80-6946: Activity in patients (pts) with advanced solid tumors and non-Hodgkin lymphoma treated in MTD expansion cohorts. J Clin Oncol 2012;30:abstr 3019.
- Kalachand R, Hennessy BT, Markman M. Molecular targeted therapy in ovarian cancer: what is on the horizon? Drugs 2011;71:947-67. [PubMed]
- Engel JB, Schönhals T, Häusler S, et al. Induction of programmed cell death by inhibition of AKT with the alkylphosphocholine perifosine in in vitro models of platinum sensitive and resistant ovarian cancers. Arch Gynecol Obstet 2011;283:603-10. [PubMed]
- Gungor H, Saleem A, Agarwal R, et al. Pharmacokinetic (PK)/pharmacodynamic (PD) analysis of escalating repeat doses of the AKT inhibitor GSK2141795 (GSK795) in patients (pts) with ovarian cancer. J Clin Oncol 2011;29:abstr 5064.