Circular RNA circZFR promotes tumorigenic capacity of lung cancer via CCND1
Introduction
Lung cancer (LC) is the third human malignant tumor and the leading cause of cancer-associated mortality around the world (1,2). Surprisingly, survival from a diagnosis of LC is longer for those who experienced a previous cancer than for those without previous cancer (3). LC is a serious cancer which can be cured if it is diagnosed at early stages, but its early diagnosis is not good (4). Thus, it is very critical to understand the molecular mechanism of LC progression and to discover novel therapeutic targets. Circular RNAs (circRNAs), which are discovered as a class of endogenous non-coding RNAs, have recently shown huge capabilities to regulate gene expression including viruses, plants, and animals (5). As a novel class of noncoding RNAs, circRNAs was reported to play important roles in various biological processes (6). CircRNAs have the potential in application of individualized cancer medicine (7). Microarray analysis of circRNAs identifies hsa_circ_0014130 as a new biomarker in non-small cell lung cancer (NSCLC) (8). Many studies showed that circRNAs were potential biomarkers for tumor diagnosis (9-11). In this study, circRNA circZFR (circBase ID: hsa_circ_0072088) located at chromosome 5p13.3. CircZFR was highly expressed in LC tissues compared with adjacent normal tissues. The deficiency and overexpression of circRNA circZFR in LC cell lines were performed to study its effects on tumor propagation, and its potential mechanism was explored.
Methods
Patients and sample collection
Thirty LC patients were recruited from Department of Respiratory Medicine, Beijing Aerospace General Hospital (Beijing, China) in this study. Pathological diagnosis was made according to the histology of tumor specimens or biopsy and examined by experienced pathologists. All tissue samples were obtained from consenting patients and this study was approved by Ethics committee of Beijing Aerospace General Hospital (Ethical approval number: KS-2016-01). The clinical data for the above patients were summarized in Table 1.
Table 1
Characteristics | Lung cancer (n=30)* |
---|---|
Sex | |
Male | 18 [60] |
Female | 12 [40] |
Age (yr) | 55.2±10.2 |
≤55 | 16 [53] |
>55 | 14 [47] |
Tumor size (cm) | |
≤5 | 17 [57] |
>5 | 13 [43] |
Differentiation | |
Low | 12 [40] |
Medium | 15 [50] |
High | 3 [10] |
*, data are shown as means ± standard deviation (SD) or numbers [%].
Cell lines and reagents
LC cell lines were from ATCC and maintained in DMEM supplemented with 10% fetal bovine serum (FBS) (Invitrogen, NY, USA), 100 µg/mL penicillin G, and 100 U/Ml streptomycin (Invitrogen, NY, USA).
ShRNA-mediated interference
ShRNAs against circRNA were designed with MIT's siRNA designer (http://sirna.wi.mit.edu/home.php). At least four quadruplexs were designed for each gene, and the most effective shRNAs were used for subsequent studies. The sequences of the effective shRNAs were provided as follows: Sh 1#: 5'-TACCTCGAGTGTAGCTACG-3', 2# 5'-CGCATAACTCGCATCGACC-3'. shRNAs and control hairpins were cloned into pSICO R vector. Production of lentiviral particles and transduction of cells was performed according to protocols from the RNAi consortium (http://www.broadinstitute.org/rnai/trc). Cells were transfected with lentiviral constructs expressing shRNA or shCtrl as described above for 24 hours. Positive cells were selected with puromycin (MCE, NJ, USA) for 5 days. Then, cells were collected for protein and RNA analysis.
CircRNA overexpression
Full-length circular cDNA was cloned into pCDNA3.1 vector, and transfected cells were generated as described above. Stable clones were obtained by selection with G418 (Thermo Fisher Scientific, USA). All constructs were confirmed by DNA sequencing.
Immunoblotting assay
Cells were lysed in RIPA lysis buffer supplemented with cocktail protease inhibitor (Roche, UK). Cytoplasamic or nuclear proteins were separated by SDS-PAGE and transferred onto polyvinylidene difluoride (PVDF) membrane (GE Healthcare, USA). The PVDF membranes were incubated with primary antibodies, followed by incubation with secondary antibodies coupled to horseradish peroxidase (R&D systems, USA).
Quantitative real time PCR (qRT-PCR) assay
Total RNA was isolated using TRIzol (Invitrogen, USA) and an RNeasy kit (QIAGEN, USA) with DNase I digestion according to the manufacturers’ instructions. cDNA was synthesized from total RNA using M-MLV reverse transcriptase (Promega) and random primers (Promega, USA). qRT-PCR was performed on an ABI7300 Real-Time PCR System (ABI 7300, Applied Biosystems). Data were normalized to 18S rRNA or β-actin or to control samples. Primers sequences for the detected genes were as follows: Circ-F: 5'-TTATCCCTACCTACTGCTGTGCC-3'; Circ -R: 5'-CCTTACTCTCCTGTTGTTGC-3'; CCND1-F: 5'-CAATCACTCCGCTCATGCTC-3', CCND1-R: 5'-CATGTGCAGCCTACCGGTT-3'; CCND2-F: 5'-TTACTCCTACTGCACTGCATC-3’, CCND2-R: 5'-ATTCGGCATACATGAATG-3'; 18S-F: 5'-AATCCGTTGAACCCCATT-3', 18S-R: 5'-CCATCCAATCGGTAGTAGCG-3'; ACTB-F: 5'-TCCCAACAGCTT-3', ACTB-R: 5'-ATGACGTCTGGGCCTGTCTA-3'.
In situ hybridization (ISH) assay
Digoxigenin-conjugated circRNA probes (targeting junction sequence of circRNA, #1: 5'-ATTCCATAGTTCTCGGCTCG-3', #2: 5'-AGCTTGCAATTATCGAGAGC-3', #3: 5'-GAACTTGTGCGTTCCAAT-3') were designed according to protocols of Biosearch Technologies (https://www.biosearchtech.com/). Indicated samples treated in non-denaturing conditions were hybridized with probe sets overnight, then incubated with HRP-conjugated secondary antibodies. After rinsing with RNA-free PBS, the sections were incubated with DAB, counterstained with hematoxylin, dehydrated and mounted. All experiments were performed according to manuals of Biosearch Technologies.
Northern blot
Total RNA was extracted from indicated samples using Trizol, then subjected to electrophoresis on a formaldehyde denaturing agarose gel for 1.5 h. Samples were transferred to positively charged NC membranes (GE Healthcare, USA) with 20× SSC (Invitrogen, USA) buffer for 12−16 hours. After UV cross-linking and prehybridization, the with secondary antibodies coupled to horseradish peroxidase (R&D systems, USA). Membrane was incubated with biotin-labeled probes at 65 °C for 16−20 hours. After washed with washing buffer, biotin signals were detected with Chemiluminescent Nucleic Acid Detection Module according to the manufacturer’s instructions. For detecting circRNAs only, junction sequences were used for probes. For detecting both circRNAs and linear RNAs, exon sequences were used for probes.
Cell proliferation assay
About 2,000−5,000 indicated cells were planted to a well of a 6-well plate and incubated for 2−3 weeks until growing to 80% in a CO2 incubator at 37 °C with 5% CO2. After washing three times with PBS, the cells were fixed with 4% PFA (Sigma, USA) for 10 min and incubated with 0.1% crystal violet for 30 min at room temperature. Plates were washed gently with distilled water until the background is clear, then subsequent to air-dry. After photographed, 33% acetic acid was added to each well to decolorize, then subsequent to measuring their absorbance value at 570 nm after sufficient shaking.
RNA fluorescence in situ hybridization (FISH)
Fluorescence-conjugated CircRNA probes were used for RNA FISH. RNA FISH was performed as previously described. Hybridization was carried out using DNA probe sets (Biosearch Technologies) according to the protocol of Biosearch Technologies. Oncosphere and control cells were observed with a FV1000 confocal laser microscopy (Olympus, Japan).
Xenograft growth in nude mice
For subcutaneous injection models, different dilutions of control and treated cells were implanted into mice (male BALB/c nude mice), aged 4 to 6 weeks, with a matrigel scaffold (BD matrigel matrix, BD biosciences) into two sides of the same nude mouse at the posterior dorsal flank region (n=4 to 6 per group). Tumors were measured every other day. Mouse experiments were approved by the Institutional Animal Care and Use Committees at Peking Union Medical College Hospital. The mice were maintained under standard conditions according to the institutional guidelines for animal care.
Statistical analysis
Statistical analysis was performed with SPSS 17.0 software (IBM, Chicago, IL). Data were shown as mean ± SD. The significance of the differences was determined by Student’s t-test. P<0.05 was considered significant.
Results
CircZFR is highly expressed in LC tumor tissues
Based on circRNA sequencing and circBase (http://www.circbase.org/) analysis, circZFR transcript was transcribed from the ZFR gene due to variable cyclizations (Figure 1A). CircZFR was an exon circRNA consisting of 13th to 17th exons of ZFR gene (Figure 1A). The circRNA was further validated by RNase R digestion (Figure 1B) and actinomycin D treatment (Figure 1C). In addition, circZFR was highly expressed in tumor tissues than peri-tumor tissues (Figure 1D). These observations were further validated by qRT-PCR analysis (Figure 1E). Furthermore, circZFR was mainly distributed in the nucleus of LC cells by RNA FISH (Figure 1F). Collectively, a conserved circRNA circZFR was highly expressed in LC tumors.
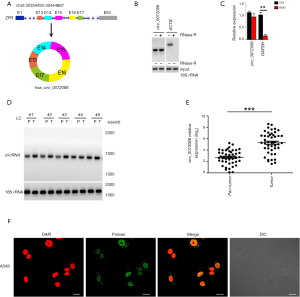
circZFR deficiency inhibits LC tumor propagation
To further test the role of circZFR in LC, we depleted circZFR in LC cells and examined its ability of tumor growth in vitro and in vivo. Given that intronic complementary sequences flanking exons are required for formation of exonic circRNAs, the exonic circRNAs do not generate if either of intronic complementary sequences is missing. The circZFR was silenced in two LC cell lines using lentivirus-mediated short hairpins RNAs (shRNAs) (Figure 2A). CircZFR deficiency significantly abrogated clone formation (Figure 2B). The circZFR deficient or wild type (WT) cells were subcutaneously injected into BALB/c nude mice. We observed that circZFR depletion substantially decreased tumor propagation compared to WT control cells (Figure 2C,D). Collectively, circZFR depletion impaired the lung tumor growth in vitro and in vivo.
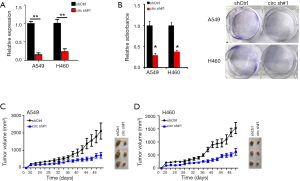
circZFR overexpression promotes LC tumorigenic capacity
CircZFR was overexpressed in LC cell lines (Figure 3A). Results indicated that circZFR overexpression did not affect the expression of its parental gene ZFR (data not shown). CircZFR overexpression was dramatically increased the capacity of cell growth in LC cell lines (Figure 3B). Consequently, circZFR overexpression substantially promoted tumor propagation (Figure 3C). Taken together, circZFR overexpression enhances tumorigenic capacity of LC.
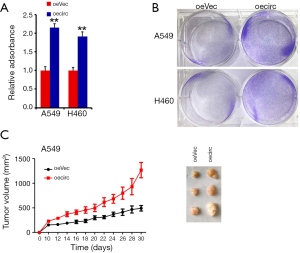
CircZFR promotes tumor propagation via CCND1 mediated cell cycle activation
To further determine target genes of circZFR, transcriptome microarray analysis was performed for circZFR deficiency and WT control LC cells. We noticed that the circZFR was positively correlated with cell cycle signaling (Figure 4A). These observations were validated in LC cell lines. Consistently, circZFR deficiency reduced the expression of CCND1 and other cell cycle genes in LC cell lines (Figure 4B,C). In contrast, circZFR depletion did not alter the expression of ZFR. Consequently, circZFR deficiency dramatically decreased H3K4me3 levels on the CCND1 promoter at ‒1,100 to ‒900 bp segment of CCND1 promoter (Figure 4D,E). Taken together, circZFR-mediated CCND1 transcription activates cell cycle signaling in LC.
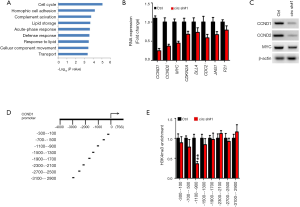
Discussion
CircRNA, a class of non-coding RNAs, is a new group of RNAs that are related to tumorigenesis, and the role of circRNAs in various diseases has been already highlighted (12). CircRNAs shape a covalently closed continuous loop which have no 5'-3' polarity and contain no polyA tail (13). There is increasing evidence that circRNAs are involved in cancer development (14-16). Our results that compared with adjacent normal tissues, circZFR was highly expressed in LC tissues. CircZFR depletion impaired the lung tumor growth in vitro and in vivo, while its overexpression enhanced tumorigenic capacity of LC. CircRNA circZFR (circBase ID: hsa_circ_0072088) was first reported by Wei et al. (17). They found that circRNA circZFR was significantly upregulated in papillary thyroid carcinoma tissues compared with adjacent normal tissues, and circZFR expression level was negatively correlated with clinical severity, demonstrating that circRNA circZFR exerted oncogenic roles via regulating miR-1261/C8orf4 axis in papillary thyroid carcinoma. In another study, circZFR knockdown significantly suppressed cell proliferation and epithelial-mesenchymal transition in hepatocellular carcinoma, and may play carcinogenic role in hepatocellular carcinoma through regulating miR-3619–5p/CTNNB1 axis and activating Wnt/β-catenin pathway (18). CircZFR could significantly distinguish the cancer samples, with an AUC of 0.7069 to distinguish live cancer cases and normal controls (19). However, there are still few studies to explore circRNA circZFR in cancer research. Through many articles have showed the relationship of circRNAs with LC (8,11,20), to our knowledge, our study firstly reported that circRNA circZFR exerted carcinogenic role on LC. And possible mechanism is that circZFR-mediated CCND1 transcription activates cell cycle signaling in LC. CCND1 gene encodes Cyclin Dl, a cyclin involved in cell cycle regulation at the Gi-S transition (21). CCND1 G870A polymorphism may be a risk factor for LC (22). Study found that miR-326 played a pivotal role on NSCLC through inhibiting cell proliferation, migration, invasion, and promoting apoptosis by targeting oncogenic CCND1 (23). The results were consistent our results, circRNA circZFR deficiency dramatically decreased H3K4me3 levels on the CCND1 promoter at ‒2,700 to ‒2,500 bp segment of CTNNB1 promoter and suppressed chromatin accessibility of the CTNNB1 locus, indicating that circZFR played important role in cell cycle signaling of LC by targeting CCND1. In conclusion, circZFR as a new circRNA was highly expressed in LC tumors, its depletion impaired the LC growth in vitro and in vivo and overexpression enhanced tumorigenic capacity of LC, and possible mechanism was related with expression of CCND1. Our results suggested the circZFR/CCND1 signaling might be a promising therapeutic target for LC treatment.
Acknowledgments
Funding: None.
Footnote
Conflicts of Interest: All authors have completed the ICMJE uniform disclosure form (available at http://dx.doi.org/10.21037/tcr.2020.04.24). The authors have no conflicts of interest to declare.
Ethical Statement: The authors are accountable for all aspects of the work in ensuring that questions related to the accuracy or integrity of any part of the work are appropriately investigated and resolved. All tissue samples were obtained from consenting patients and this study was approved by Ethics committee of Beijing Aerospace General Hospital (Ethical approval number: KS-2016-01).
Open Access Statement: This is an Open Access article distributed in accordance with the Creative Commons Attribution-NonCommercial-NoDerivs 4.0 International License (CC BY-NC-ND 4.0), which permits the non-commercial replication and distribution of the article with the strict proviso that no changes or edits are made and the original work is properly cited (including links to both the formal publication through the relevant DOI and the license). See: https://creativecommons.org/licenses/by-nc-nd/4.0/.
References
- Zhang Y, Wang Y, Wang J. MicroRNA-584 inhibits cell proliferation and invasion in non-small cell lung cancer by directly targeting MTDH. Exp Ther Med 2018;15:2203-11. [PubMed]
- Li X, Dong X, Lian J, et al. Knockoff filter-based feature selection for discrimination of non-small cell lung cancer in CT image. IET Image Processing 2019;13:543-8. [Crossref]
- Ge Z, Heitjan DF, Gerber DE, et al. Estimating lead-time bias in lung cancer diagnosis of patients with previous cancers. Stat Med 2018;37:2516-29. [Crossref] [PubMed]
- Wang Y, Song G, Wang Y, et al. Elevated serum levels of circulating immunoinflammation-related protein complexes are associated with cancer. J Proteome Res 2014;13:710-9. [Crossref] [PubMed]
- Lux S, Bullinger L. Circular RNAs in Cancer. Adv Exp Med Biol 2018;1087:215-30. [Crossref] [PubMed]
- Guo L, Zheng L, Zhao Y, et al. Profiling and Bioinformatic Analyses Indicate Differential circRNA and miRNA/isomiR Expression and Interactions. Biomed Res Int 2018;2018:8518563.
- Zhang M, Xin Y. Circular RNAs: a new frontier for cancer diagnosis and therapy. J Hematol Oncol 2018;11:21. [Crossref] [PubMed]
- Zhang S, Zeng X, Ding T, et al. Microarray profile of circular RNAs identifies hsa_circ_0014130 as a new circular RNA biomarker in non-small cell lung cancer. Sci Rep 2018;8:2878. [Crossref] [PubMed]
- Hu W, Bi ZY, Chen ZL, et al. Emerging landscape of circular RNAs in lung cancer. Cancer Lett 2018;427:18-27. [Crossref] [PubMed]
- Chen Y, Wei S, Wang X, et al. Progress in research on the role of circular RNAs in lung cancer. World J Surg Oncol 2018;16:215. [Crossref] [PubMed]
- Zong L, Sun Q, Zhang H, et al. Increased expression of circRNA_102231 in lung cancer and its clinical significance. Biomed Pharmacother 2018;102:639-44. [Crossref] [PubMed]
- Zhu Z, Li Y, Liu W, et al. Comprehensive circRNA expression profile and construction of circRNA-associated ceRNA network in fur skin. Exp Dermatol 2018;27:251-7. [Crossref] [PubMed]
- Zhang HD, Jiang LH, et al. CircRNA: a novel type of biomarker for cancer. Breast Cancer 2018;25:1-7. [Crossref] [PubMed]
- Meng S, Zhou H, Feng Z, et al. CircRNA: functions and properties of a novel potential biomarker for cancer. Mol Cancer 2017;16:94. [Crossref] [PubMed]
- Dang Y, Ouyang X, Zhang F, et al. Circular RNAs expression profiles in human gastric cancer. Sci Rep 2017;7:9060. [Crossref] [PubMed]
- Hansen TB, Kjems J, Damgaard CK. Circular RNA and miR-7 in cancer. Cancer Res 2013;73:5609-12. [Crossref] [PubMed]
- Wei H, Pan L, Tao D, et al. Circular RNA circZFR contributes to papillary thyroid cancer cell proliferation and invasion by sponging miR-1261 and facilitating C8orf4 expression. Biochem Biophys Res Commun 2018;503:56-61. [Crossref] [PubMed]
- Tan A, Li Q, Chen L. CircZFR promotes hepatocellular carcinoma progression through regulating miR-3619–5p/CTNNB1 axis and activating Wnt/β-catenin pathway. Arch Biochem Biophys 2019;661:196-202. [Crossref] [PubMed]
- Ren S, Xin Z, Xu Y, et al. Construction and analysis of circular RNA molecular regulatory networks In liver cancer. Cell Cycle 2017;16:2204. [Crossref] [PubMed]
- Yao JT, Zhao SH, Liu QP, et al. Over-expression of CircRNA_100876 in non-small cell lung cancer and its prognostic value. Pathol Res Pract 2017;213:453-6. [Crossref] [PubMed]
- Bosch F, Campo E, Jares P, et al. Increased expression of the PRAD-1/CCND1 gene in hairy cell leukaemia. Br J Haematol 1995;91:1025-30. [Crossref] [PubMed]
- Zhou C, An H, Hu M, et al. The cyclin D1 (CCND1) G870A polymorphism and lung cancer susceptibility: a meta-analysis. Tumour Biol 2013;34:3831-7. [Crossref] [PubMed]
- Sun C, Huang C, Li S, et al. Hsa-miR-326 targets CCND1 and inhibits non-small cell lung cancer development. Oncotarget 2016;7:8341-59. [Crossref] [PubMed]