Preliminary study on the relationship among stem cell markers, drug resistance and PI3K signaling pathway in multiple myeloma (MM) cell
Introduction
Multiple myeloma (MM) could cause multi-organ damage, such as the hemopoietic system, bones, and kidneys. The reasons are uncontrollable monoclonal plasma cell proliferation and the secretion of monoclonal immunoglobulin (1). Although there are many different treatment options, most patients will have a good outcome in case of receiving an early treatment. However, after the continuous treatment of either chemotherapy, or radiation therapy, some patients will relapse due to acquired drug resistance. Hence, MM remains as an incurable disease (1,2). Therefore, the study of its mechanism of resistance has great significance in the research of new drugs, prolonging the survival time of patients, and improving the prognosis.
Cancer is a chronic disease that is widespread worldwide. Mostly, the high mortality from cancer correlates with the lack of clear symptoms, which results in late diagnosis for patients, and consequently, advanced tumor disease with poor probabilities for cure, because many patients will exhibit chemo- and radio-resistance. Several mechanisms have been studied to explain chemo- and radio-resistance to anti-tumor therapies, including cell signaling pathways, anti-apoptotic mechanisms, stemness, metabolism, and cellular phenotypes. Interestingly, the presence of cancer stem cells, which are a subset of cells within the tumors, has been correlated to therapy resistance (3-8).
In our study, we aim to explore the mechanism of drug resistance in multiple myeloma (MM).
Methods
Cells culture
The Roswell Park Memorial Institute (RPMI) 1640 medium (Gibco BRL) and 10% fetal calf serum (Gibco BRL) was used to culture cells (37 °C in a 5% CO2 atmosphere). The RPMI-8226 cell lines were acquired from the Chinese Academy of Sciences. Cells were observed, and received radiation doses of 6 Gy in the logarithmic growth phase. For the Cell Counting Kit 8 (CCK-8) CCK-8 assay, 2×104 cells that received radiation or did not receive radiation were separately seeded per well in a 96-well plate. Each plate was added with 100 µL of cell culture medium and bortezomib at different concentrations (Xian Janssen). At 48 hours later, these cells were incubated with 10 µL of Cell Counting Kit (CCK) solution (Dojindo) for 1–4 hours. The absorbance was measured by microplate autoreader (BIO-RAD).
Flow cytometry
For the cell cycle distribution assay, cells were fixed with 70% ethanol (Sigma) at −20 °C overnight, and washed with phosphate buffered saline. Then, these cells were resuspended in phosphate buffer saline (PBS), concomitantly treated with Rnase A (Sigma), and stained with 50 µg/mL of propidium iodide (PI; KeyGEN BioTECH) for 15 minutes. Afterwards, cell apoptosis was assessed using an Annexin V staining Kit (KeyGEN BioTECH). Next, cells were washed twice with PBS and resuspended in binding buffer before incubation with Annexin V and PI for 15 minutes at room temperature. The cell cycle distribution and apoptosis were analyzed using BD FACSCanto.
Western blotting
Cells were lysed in Radio Immunoprecipitation Assay (RIPA) buffer, 1 mM salt of sodium (NaF), 10 mM Na3VO4, 1 mM Phenylmethylsulfonyl fluoride (PMSF), and a protease inhibitor cocktail (Roche, Indianapolis, IN, USA). After incubation at 4 °C for 30 minutes, the lysate was centrifuged at 15,000 g for 10 minutes at 4 °C. The protein concentration was determined using a bicinchoninic acid (BCA) assay (Pierce, Rockford, IL, USA). The samples were denatured for five minutes at 95 °C and subjected to 10% SDS⁄PAGE. Then, the separated proteins were transferred onto a polyvinylidene fluoride (PVDF) membrane (Millipore, Bedford, MA, USA). Afterwards, the membrane was blocked in 5% (w⁄v) skim milk-Tris-Buffered Saline Tween-20 (TBST) (10 mM Tris, 150 mM NaCl, and 0.05% Tween 20, pH 8.3) solution, followed by incubation with the primary antibodies diluted in skim milk-TBST solution overnight at 4°C. Subsequently, the membrane was incubated with the corresponding horseradish peroxidase-conjugated secondary antibody (Cell Signaling) for one hour at room temperature, and the immunoreactive protein bands were visualized by enhanced chemiluminescence reagents (Millipore).
Results
The bortezomib 48-hour IC50 of RPMI-8226
MM cell RPMI-8226 exhibited a significant change in morphology and drug sensitivity after radiation treatment. As shown in Figure 1, cells without radiation treatment grew suspended and exhibited normal morphology (Figure 1A). Cells with radiation treatment had a partly adherent growth, irregular membrane shape, and larger nucleolus (Figure 1B). The bortezomib 48-hour IC50 of cells without radiation treatment was 29.9 nmol/L, while the bortezomib 48-hour IC50 of cells after radiation treatment was 89.5 nmol/L. The NVP-BEZ235 for the 48-hour IC50 of RPMI-8226 was 8.3×106 mol/L, and cells were finally treated with 1/2 48-hour IC50 (4×106 mol/L).
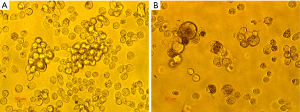
Cell cycle
The cell cycle analysis of RPMI-8226 by flow cytometry revealed that 16.74% of cells without irradiation or bortezomib treatment were in the G0/G1 phase (Figure 2A), 36.10% cells were in the G0/G1 phase after bortezomib was added at 48 hours before irradiation (Figure 2B), and 24.11% cells were in the G0/G1 phase after bortezomib was added at 48 hours after irradiation (Figure 2C). These indicate that the induction of the G0/G1 cell cycle arrest by bortezomib on radiation-treated cells was significantly attenuated.
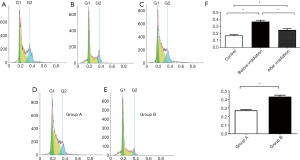
Radiation-treated cells were divided into group A and group B. Group A was added with 1/2 48-hour IC50 of bortezomib, and the cell cycle was tested after 48 hours. The ratio of the G0/G1 phase was 26.59% (Figure 2D). Group B was added with 1/2 48-hour IC50 of bortezomib and 1/2 48-hour IC50 of NVP-BEZ235, and the cell cycle was tested after 48 hours. The ratio of the G0 phase cells to G1 phase cells was 43.54% (Figure 2E). These suggest that NVP-BEZ235 could contribute in significantly improving the effect of inducing the G0/G1 cell cycle arrest by bortezomib on radiation-treated cells (Figure 2F).
Apoptosis of cells
The apoptosis analysis by flow cytometry revealed that after adding bortezomib at 48 hours before irradiation, early apoptosis cells + late apoptosis cells accounted for 53.73% (Figure 3A), while after radiation-treated cells were added with bortezomib at 48 hours, early apoptosis cells + late apoptosis cells accounted for 31.25% (Figure 3B). These indicate that the apoptotic induction effect of bortezomib on irradiated cells was significantly attenuated.
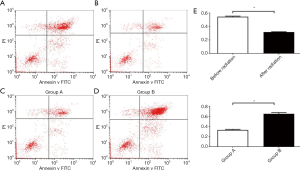
Group A was added with 1/2 48-hour IC50 of bortezomib, and apoptosis was tested after 48 hours. The rate of apoptosis was 33.13% (Figure 3C). Group B was added with 1/2 48-hour IC50 of bortezomib and 1/2 48-hour IC50 of NVP-BEZ235, and apoptosis was tested after 48 hours. The rate of apoptosis was 66.14% (Figure 3D). These indicate that NVP-BEZ235 could significantly increase the apoptotic induction of bortezomib on cells (Figure 3E).
Stem cell markers and the PI3K pathway
The protein expression of cells with radiation treatment was detected by Western blotting. It was revealed that the expression levels of stem cell markers (CD44, Oct4, and Sox2) were higher after radiation treatment (Figure 4A). However, when cells with radiation treatment were added with NVP-BEZ235, the expression levels of thse stem cell markers (CD44, Oct4, and Sox2) decreased (Figure 4B,C).
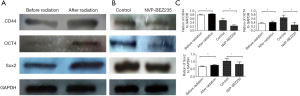
The results of Western blotting revealed that the expression levels of p110, p85, p-AKT and p-mTOR were higher after radiation treatment, indicating that radiation treatment may activate the PI3K/AKT/mTOR signaling pathway (Figure 5A). When cells with radiation treatment were added with NVP-BEZ235, the expression of p110, p85, p-AKT and p-mTOR decreased (Figure 5B,C).
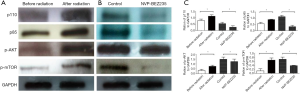
Discussion
MM stem cell has become a popular topic (9-11). This study aims to investigate the relationship among stem cell markers, drug resistance and the PI3K signaling pathway in MM cell line RPMI-8226.
A previous study presented that the combined evaluation of Nanog, Oct4, Sox2, PCNA, Ki67 and E-cadherin was valuable for patients with gastric cancer (12). The high level of stem cell markers Sox2 and Oct4 was associated with poor prognosis: later stage, poorer disease-free survival, and overall survival. Evidence has indicated that most tumors contain a small population of cells, and these are called “cancer stem cells”. Because these share several markers of normal stem cells. These cells display chemotherapy resistance, thereby causing the relapse of cancer (13). One of the characteristics is the overexpression of stem cell-specific transcription factors, such as Nanog, Oct4 and Sox2. These factors could sustain pluripotency of embryonic stem cells (14). The clinical data demonstrated that gene expression signatures associated with the “stemness” state of a cell are informative as molecular predictors of cancer therapy outcome, and can help to identify cancer patients with therapy-resistant tumors. Furthermore, Nanog, Oct4 and Sox2 can stimulate cancer cell growth and anti-apoptosis, thereby making it critical in carcinogenesis (15,16). Nanog, Sox2, or Oct4 has been reported to lead to the activation of growth factors, promoting cellular proliferation and metastasis in several types of malignancies.
The present study mainly investigated MM cell line RPMI-8226. After receiving radiation dose of 6 Gy in the logarithmic growth phase, these RPMI-8226 cells exhibited changes, in terms of cell morphology, cell cycle, apoptosis, and stem cell markers, and changes in signaling pathways. It was considered that these MM cells that survived the radiotherapy, might have obtained some stemness, and had the ability to resist bortezomib. Compared with untreated cells, RPMI-8226 cells had higher levels of PI3K pathway protein which activated the PI3K pathway.
The PI3K/AKT/mTOR signaling pathway plays a role in the resistance to several existing therapies (17). Hsu et al. (18) reported that AKT phosphorylation was significantly higher in D-S III stage MM patients (49%) than D-S phase (9%) patients and asymptomatic MM patients (6%). Besides, AKT phosphorylation could independently contribute to the survival of MM cell.
Drug resistance and relapse remain as the major factors that limit the survival of MM patients. However, the mechanism remains unknown which correlated to P53 mutation, and the activation of NF-kB, IL-6, CD44 and MM cell differentiation. It has been reported that AKT phosphorylation can inhibit MM cell apoptosis (17,19,20). NVP-BEZ235 can inhibit all PI3K isomers and mutant PI3K, as well as mTOR. This phenomenon was observed when the PI3K/AKT/mTOR signaling pathway was blocked by NVP-BEZ235. The combination of NVP-BEZ235 and bortezomib can increase the apoptotic rate of RPMI-8226 cells that survive the radiotherapy. When the PI3K/AKT/mTOR signaling pathway was blocked, the expression of stem cell markers CD44, Oct4 and Sox2 is downregulated. In addition, the expression of p85, p110, p-AKT and p-mTOR are also downregulated.
Therefore, to some extent, the present study revealed that the stemness of RPMI-8226 cells that survive the radiotherapy decreased, and the sensitivity of cells to bortezomib partially recovered after blocking the PI3K/AKT/mTOR signaling pathway by NVP-BEZ235. In the future, there is a need to use more kinds of MM cells, especially in clinical practice, in order to further verify these above results and assumptions.
Acknowledgements
Funding: This study was supported by grants from
Footnote
Conflicts of Interest: All authors have completed the ICMJE uniform disclosure form (available at http://dx.doi.org/10.21037/tcr-19-2116). The authors have no conflicts of interest to declare.
Ethical Statement: The authors are accountable for all aspects of the work in ensuring that questions related to the accuracy or integrity of any part of the work are appropriately investigated and resolved.
Open Access Statement: This is an Open Access article distributed in accordance with the Creative Commons Attribution-NonCommercial-NoDerivs 4.0 International License (CC BY-NC-ND 4.0), which permits the non-commercial replication and distribution of the article with the strict proviso that no changes or edits are made and the original work is properly cited (including links to both the formal publication through the relevant DOI and the license). See: https://creativecommons.org/licenses/by-nc-nd/4.0/.
References
- Palumbo A, Anderson K. Multiple myeloma. N Engl J Med 2011;364:1046-60. [Crossref] [PubMed]
- Richardson PG, San Miguel JF, Moreau P, et al. Interpreting clinical trial data in multiple myeloma: translating findings to the real-world setting. Blood Cancer J 2018;8:109. [Crossref] [PubMed]
- Toledo-Guzmán ME, Bigoni-Ordóñez GD, Ibáñez Hernández M, et al. Cancer stem cell impact on clinical oncology. World J Stem Cells 2018;10:183-95. [Crossref] [PubMed]
- Chang HL, Chen HA, Bamodu OA, et al. Ovatodiolide suppresses yes-associated protein 1-modulated cancer stem cell phenotypes in highly malignant hepatocellular carcinoma and sensitizes cancer cells to chemotherapy in vitro. Toxicol In Vitro 2018;51:74-82. [Crossref] [PubMed]
- Su YK, Shih PH, Lee WH, et al. Antrodia cinnamomea sensitizes radio-/chemo-therapy of cancer stem-like cells by modulating microRNA expression. J Ethnopharmacol 2017;207:47-56. [Crossref] [PubMed]
- Wang A, Qu L, Wang L. At the crossroads of cancer stem cells and targeted therapy resistance. Cancer Lett 2017;385:87-96. [Crossref] [PubMed]
- Jin X, Jin X, Kim H. Cancer stem cells and differentiation therapy. Tumour Biol 2017;39:1010428317729933. [Crossref] [PubMed]
- Leon G, MacDonagh L, Finn SP, et al. Cancer stem cells in drug resistant lung cancer: Targeting cell surface markers and signaling pathways. Pharmacol Ther 2016;158:71-90. [Crossref] [PubMed]
- Ware WR. Natural Cancer Therapy and Prevention Targeted on Cancer Cells and Cancer Stem Cells Based on the Cytochrome P45O Enzyme CYP1B1 A Commentary. Altern Ther Health Med 2017;23:50-8. [PubMed]
- Wang N, Liang X, Yu W, et al. Differential Expression of MicroRNA-19b Promotes Proliferation of Cancer Stem Cells by Regulating the TSC1/mTOR Signaling Pathway in Multiple Myeloma. Cell Physiol Biochem 2018;50:1804-14. [Crossref] [PubMed]
- Martins LNGF, Morita AA, Broto GE, et al. Interferon-gamma in mobilized stem cells: A possible prognostic marker in early post-transplant management in multiple myeloma. Cytokine 2018;108:127. [Crossref] [PubMed]
- Li N, Deng W, Ma J, et al. Prognostic evaluation of Nanog, Oct4, Sox2, PCNA, Ki67 and E-cadherin expression in gastric cancer. Med Oncol 2015;32:433. [Crossref] [PubMed]
- Vidal SJ, Rodriguez-Bravo V, Galsky M, et al. Targeting cancer stem cells to suppress acquired chemotherapy resistance. Oncogene 2014;33:4451-63. [Crossref] [PubMed]
- Jeong HC, Park SJ, Choi JJ, et al. PRMT8 Controls the Pluripotency and Mesodermal Fate of Human Embryonic Stem Cells by Enhancing the PI3K/AKT/SOX2 axis. Stem Cells 2017;35:2037-49. [Crossref] [PubMed]
- Li C, Zhu M, Lou X, et al. Transcriptional factor OCT4 promotes esophageal cancer metastasis by inducing epithelial-mesenchymal transition through VEGF-C/ VEGFR-3 signaling pathway. Oncotarget 2017;8:71933-45. [Crossref] [PubMed]
- Wang H, Liu B, Wang J, et al. Reduction of NANOG Mediates the Inhibitory Effect of Aspirin on Tumor Growth and Stemness in Colorectal Cancer. Cell Physiol Biochem 2017;44:1051-63. [Crossref] [PubMed]
- Ramakrishnan V, Kumar S. PI3K/AKT/mTOR pathway in multiple myeloma: from basic biology to clinical promise. Leuk Lymphoma 2018;59:2524-34. [Crossref] [PubMed]
- Hsu JH, Shi Y, Hu L, et al. Role of the AKT kinase in expansion of multiple myeloma clones: effects on cytokine-dependent proliferative and survival responses. Oncogene 2002;21:1391-400. [Crossref] [PubMed]
- Manni S, Carrino M, Manzoni M, et al. Inactivation of CK1α in multiple myeloma empowers drug cytotoxicity by affecting AKT and β-catenin survival signaling pathways. Oncotarget 2017;8:14604-19. [Crossref] [PubMed]
- Kinoshita S, Ri M, Kanamori T, et al. Potent antitumor effect of combination therapy with sub-optimal doses of Akt inhibitors and pomalidomide plus dexamethasone in multiple myeloma. Oncol Lett 2018;15:9450-6. [PubMed]