The diagnostic value of extracellular protein kinase A (ECPKA) in serum for gastric and colorectal cancer
Introduction
Gastrointestinal cancer, comprising a diverse group of tumors with varying clinical course and prognosis, is one of the most common cancer-related death worldwide due to the high incidence and overall poor outcome. For example, gastric cancer (GC) represents the fifth most common malignancy and remains the third leading cause of cancer mortality with widely varying incidence worldwide, while colorectal cancer (CRC) is the third most common cancer in men and the second most common in women (1). Advances over the last decade in molecular diagnostics in GC and CRC have a benefit to improve our understanding of the complex mechanisms in the development and progression (2). However, the low rate of early diagnosis means that most patients have the advanced-stage disease at diagnosis and so the best surgical window is missed, leading that the 5-year survival rate of patients is extremely low. So, early prediction/detection plays a vital role in improving their prognosis and optimal treatment. Although there is much progress in the cancer diagnosis due to endoscopic ultrasonography application, the early detection of serum biomarkers still has its advantages in patient diagnosis, monitoring, and therapeutic management, compared with some other screening programs (3). It is essential to discover new biomarkers of gastric cancer that could give predictive information about the response to therapy and finally improve the therapeutic outcomes (4). Currently, some serum biomarkers have been identified, including vascular endothelial growth factor, aberrant DNA or RNA, carcinoembryonic antigen (CEA), alpha-fetoprotein (AFP), cancer antigens (i.e., CA125), most of them have already used in the clinic, such as CEA, CA125, and AFP. Unfortunately, the ideal specific biomarker for the prediction of gastrointestinal cancer in the early period remains devoid. Protein Kinase A (PKA), also known as a cAMP-dependent protein kinase, is a serine/threonine kinase that is responsible for phosphorylating a wide range of downstream substrates, the extent of which depends on cellular localization (5-7). PKA is inactive heterotetramers of four regulatory (R) subunits, including RIα, RIβ, RIIα and RIIβ, and three catalytic (C) subunits, including Cα, Cβ, and Cγ (8). Each R subunit contains a flexible inhibitory segment that limits catalytic activity by blocking access to the active site of the C subunit (8,9). The binding of cAMP to the regulatory subunit leads to the dissociation and activation of the catalytic subunits and thus releases the C subunits to phosphorylate downstream cytoplasmic and nuclear targets (10,11). One main target in nuclear is the transcription factor cAMP response binding protein (CREB) (12).
PKA is considered as an essential regulator of several physiological processes (13,14), including ion channels, enzymes catalyzing biosynthesis, metabolism, transmembrane receptors, and other signaling mediator proteins (9,15). More importantly, although PKA is not traditionally considered as an oncogene, dysregulation of PKA signaling is implicated in tumorigenesis and progression of numerous cancers (16). For example, an extracellular form of PKA has been detected in the conditioned medium and serum from several cancer patients, including prostate, renal, bladder, breast, lung, melanoma, lymphomas, and adrenal carcinomas (11,16-19). More recently, it was reported that upregulation transcripts of PKA catalytic subunit alpha (PKACα) have also been detected in breast cancer, specifically in patients resistant to trastuzumab (20); elevated PKACα transcript levels in tissues can also lead to fibrolamellar hepatocellular carcinoma (21). Many studies have indicated that PKA is expressed in various types of cancers; however, the relationship between its expression pattern and disease prognosis remains unclear. Besides, recently, some reports showed that free catalytic subunit of PKA (PKAC) was found in the peripheral blood of cancer patients and secreted by some cancer cells (11,17). This form PKA is active, designated as extracellular protein kinase A (ECPKA) (22). About the role of ECPKA in cancer, there are some different views. Some reports showed that the released PKAC from conditioned media of cultured cancer cells could inhibit angiogenesis (23). And some others found that the ECPKA expression in serum of cancer patients is up-regulated as compared with normal serum (11). So, it is imperative to further elucidate the role of ECPKA in cancer. In this study, we examined the level of PKACα in serum and tissue of gastric and colon cancer people and investigated the relationship between PKACα expression and clinicopathological factors in the diagnosis and prediction of gastric and colon cancer patients.
We present the following article in accordance with the REMARK reporting checklist (available at http://dx.doi.org/10.21037/tcr-20-764).
Methods
Reagents
PKACα ELISA kit was obtained from Cloud-Clone-Corp (Katy, TX, USA). RIPA buffer was from Proteintech (Rosemont, IL, USA). Antibodies against PKACα and β-actin were purchased from Cell Signaling Technology (Danvers, MA, USA). Electrochemiluminescence (ECL) reagents were purchased from Millipore (Billerica, MA, USA).
Serum samples
Serum samples were collected from regular healthy volunteers (n=24), patients with gastric cancer (n=42), and colorectal cancer (n=20) from the Affiliated Hospital of Jiangsu University in Zhenjiang of China. Serum was obtained by centrifugation at 2,000 rpm for 20 min at 4 °C and stored at −80 °C until use. The diagnosis of cancer for each patient was confirmed by pathology analysis. All the patients didn’t receive any treatment. Healthy volunteers, without evidence of surgical history, chronic/acute diseases, or current use of any medication, were randomly recruited into this study. All patients provided informed consent. The study involving human subjects was approved by the Human Ethics Committee of School of Medicine, Jiangsu University.
Resected specimens
The surgically resected specimens from patients who underwent curative surgery (24 gastric cancer and 13 colon cancer) were obtained from the Affiliated Hospital of Jiangsu University in Zhenjiang of China. All patients were consent informed. Resected specimens were stored in liquid nitrogen until analysis. The study involving human subjects was approved by the Medical Ethics Committee of Jiangsu University.
ELISA assay
The serum samples were detected according to the PKACα ELISA kit manufacturer’s specifications and read at 450 nm immediately. And the BCA Protein Quantification Kit (Sigma-Aldrich, MO, USA) was applied for determining the total concentration of proteins in the tissue lysis. The data were obtained from three independent experiments.
Tissue lysis
The cancer tissue and adjacent mucosa were cut into smaller pieces while keeping them on ice and transferred into a homogenizer and then homogenized thoroughly with RIPA buffer plus protease inhibitors on ice. Centrifuged at 12,000 ×g for 20 minutes at 4 °C to pellet cell debris, and then transferred the supernatant to a fresh microfuge tube. Samples were frozen at −80 °C until used.
Western blotting
Equal amounts of protein lysis were separated by 8–10% SDS-PAGE gels electrophoresis and then transferred to polyvinylidene difluoride (PVDF) membranes. After blockading by 5% non-fat dried milk, the membranes were incubated with specific primary antibodies against PKACα or β-actin overnight at 4 °C. After washing 3 times with TBS/T, the horseradish peroxidase-labeled (HRP) secondary antibodies were incubated for 1 h at room temperature. The bands were detected with ECL reagents.
Statistical analysis
The population expression of PKACα was analyzed with age, gender, metastasis, and some tumor makers. Two groups were compared by t-test for normal distribution and Wilcoxon Rank Sum Test for non-normal distribution. Differences among normally distributed data of three or more groups were analyzed by one-way ANOVA, following by the Bonferroni post hoc test. The relationship of serum PKACα, gender, liver metastasis, and lymphatic metastasis was analyzed by the Mann-Whitney test. The rank correlation of serum PKACα and T stage, age, and tumor markers were analyzed by Spearman rank correlation and Pearson correlation. SPSS 24.0 software and GraphPad Prism 8.0 were applied to assay all the above data, and P<0.05 was considered significant for all tests.
Results
PKACα in serum of the cancer patient is higher than healthy people
To investigate whether there are differences in the serum levels of PKACα between patients with gastric, colorectal cancers and volunteers, the serum of patients was collected and detected by ELISA. The data were first analyzed by Kolmogorov-Smirnov (K-S) test, and the results showed that Z=0.127, P=0.002, indicating that level of PKACα in serum and were abnormal distributions (Table 1), so the data were further analyzed by Kruskal-Wallis H test, and multiple comparisons of PKACα in serum between the three groups were analyzed by Bonferroni correction. The results showed that the levels of serum PKACα were higher in patients with colon and gastric cancer (258.75±90.20 and 288.31±177.67 pg/mL, respectively) compared with the volunteers (191.08±73.44 pg/mL) (Figure 1A, Tables 1,2). There were significant differences between the volunteers and the gastric cancers (P<0.01), but no difference between the volunteers and the colorectal cancers (P>0.05). ROC analyses were performed to further evaluate the utility of PKACα as a potential cancer biomarker (Figure 1B,C). For gastric cancer, the sensitivities and specificities of PKACα was 61.90% and 87.50%, at the cutoff value of 253.92 pg/mL, with an AUC value of 0.727 (Z=3.637, P<0.001). The above results implied that specific PKACα levels might be a potential biomarker for gastric cancer diagnosis while it is not suitable for the diagnosis of colorectal cancer.
Table 1
Index | Z | P |
---|---|---|
PKACα in serum | 0.127 | 0.002 |
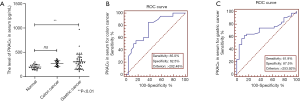
Table 2
Index | Normal | Colon cancer | Gastric cancer | H | P |
---|---|---|---|---|---|
PKACα, M (Q) | 191.08 (73.44) | 258.75 (90.20) | 288.31 (177.67) | 11.786 | 0.002 |
M, median; Q, inter-quartile range.
Association between serum PKACα and clinicopathological factors
To investigate whether there is a relationship between serum PKACα and clinicopathological factors. Mann-Whitney test was applied to analyze the patients’ characteristics and serum PKACα. Also, the results in Table 3 showed that the differences of gender, liver metastasis, and lymphatic metastasis were not significantly co-related with the difference of the levels of serum PKACα. Besides, the analysis of Spearman rank correlation was applied to analyze the relationship of PKACα in serum with T stage, age, and there was no association between PKACα and T stage (r=−0.231, P=0.094) or age (r=0.129, P=0.322). And the Pearson Correlation analysis was applied for the relationship of PKACα and tumor markers (including CA50, CA199, AFP, CEA, CA125, CA242, CA724, and Ferritin). The result in Figure 2 showed that PKACα in serum was positively-correlated with CA50 (Pearson r=0.482, P=0.042), but was not associated with other factors or tumor markers, including AFP, CA199, CEA, CA125, CA242, CA724, and Ferritin.
Table 3
Characteristic | PKACα, M (Q) | Z | P |
---|---|---|---|
Gender | −1.593 | 0.111 | |
Male | 257.48 (232.65) | ||
Female | 293.98 (195.83) | ||
Liver metastasis | −1.818 | 0.069 | |
None | 270.16 (168.74) | ||
Yes | 166.91 (63.51) | ||
Lymphatic metastasis | −0.615 | 0.539 | |
None | 236.75 (134.38) | ||
Yes | 302.63 (139.64) |
M, median; Q, inter-quartile range.
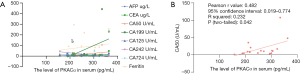
The PKACα expression in cancer tissue and the adjacent mucosa
The expression of PKACα in colon cancer tissue (n=13), gastric cancer (n=24) tissue and the adjacent mucosa were also detected. The western blotting and ELISA results (Figure 3 and Table 4) showed that in the colon cancer patients (n=13), there were 5 cases, the PKACα expression in cancer tissue is higher than that in the adjacent noncancerous tissues, while 8 cases, no higher. But there was no significant difference of PKACα expression between the colon cancer tissue and the adjacent mucosa (P>0.05). In gastric cancer patients (n=24), there are 8 cases, the PKACα expression in cancer tissue is higher than that in the adjacent noncancerous tissues, while 16 cases, no higher. Similarly, PKACα expressed in the gastric cancer tissue and the adjacent mucosa with no significant difference (P>0.05).
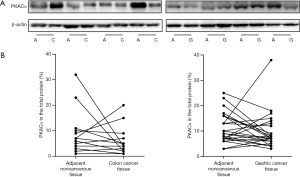
Table 4
Groups | PKACα in the total protein (%) (mean ± SEM) | t value | P value | |
---|---|---|---|---|
The adjacent mucosa | The cancer tissue | |||
PKACα in gastric cancer | 11.29±01.22 | 9.75±1.49 | 0.9027 | 0.3760 |
PKACα in colon cancer | 9.23±2.45 | 6.00±1.58 | 1.0940 | 0.2954 |
SEM, standard error of mean.
Discussion
Malignancies of the gastrointestinal tract, such as CRC, GC, and pancreatic carcinoma, are among the most common human cancers (1,24), and each kind of them has specific clinical features (24,25). Usually, the conventional therapies for these malignancies include radiotherapy, chemotherapy, and target therapies. For example, a standard first-line therapy for advanced CRC is the combination of chemotherapy and EGFR inhibitor or VGFR inhibitor (26). Although many chemotherapeutic agents and combinations are available, the overall prognosis of patients with advanced gastric and colorectal cancer remains poor (27), and the clinical outcomes of the patients are still not encouraging with a low rate of 5 years survival (28). Some of the reason is due to the concealed early symptoms and lack of sensitive diagnostic biomarkers. So, the prognosis of gastric and colorectal cancer patients can be greatly improved by early detection or prediction of the metastatic potential of the primary tumor. Although there is much progress in the cancer diagnosis due to endoscopic ultrasonography application, the early detection of serum biomarkers still has its advantages in patient diagnosis, monitoring, and therapeutic management, compared with some other clinical screening examination (3). Currently, some serum biomarkers have been used in the clinic, while the ideal biomarker for gastric and colorectal cancer in the early period is not yet identified.
Recent molecular studies showed that some kinase inhibitors were taken as targeted therapies in gastric or colon tumors (25). So far, there are 518 kinases confirmed in human (25). The elucidation of the first kinase signaling cascade involving PKA in the 1960s marked a milestone (29), followed by the breakthrough discovery that protein kinases can act as oncogenes, which won the 1989 Nobel Prize in Physiology (30). The function of the intracellular PKA in cell survival and migration has been widely studied (31). Recently, there was indeed an intriguing phenomenon (11,16,17,19,23) that the release of PKACα into the extracellular environment, including the serum of cancer patients. This form of PKA does not require activation by cAMP, which is distinct from the intracellular tetrameric holoenzyme, and the release of ECPKA into the extracellular milieu is not due to cell lysis (16,17,22). Though the function and mechanism of the secreted kinase are poorly known, it is clear that there are marked differences between ECPKA and intracellular PKA (23).
In our study, there were significant differences between the volunteers and the gastric cancers, and the ROC analyses also implied that specific PKACα levels might be a potential biomarker for the gastric cancer diagnosis. Moreover, the release of ECPKA in peripheral blood has been further confirmed in other reports from various cancer patients, including breast, colon, cervical, lung, prostate, pancreatic, ovarian, and rectal carcinomas, and surgical removal of tumors led to a decrease in ECPKA levels in patients (11,16,19,22). More recently, there is increasing evidence that patients with cancer produce autoantibodies against antigens in their tumors, such as c-myc, p53, and ECPKA (22,32,33). The autoantibody against ECPKA has been detected in the serum of many patients by an autoantibody enzyme immunoassay (34). These results indicated that extracellular PKA might serve as a cancer antigen for diagnosis and prediction of cancer. However, in the gastric and colon cancer tissue, the expression of PKACα is not always higher or lower than the adjacent mucosa, and there was no significant difference in this research. One reason is that the number of patients is insufficient and the pathological stage is inconsistent. The second, PKA is a heterotetramer. No data show whether a complete or partial tetramer is secreted into the extracellular milieu, however, in the present work, only the catalytic α subunit was detected. In mammalian cells, there are two types of PKA (PKA-I and PKA-II). It is known that there are four R isoforms of PKA, with this common catalytic (C) subunit (22), and this PKA-RI and RII showed a different function on the tumor (16). The RII subunits, expressed preferentially in healthy tissues, play a growth-inhibitory role; the RI subunits, promote growth and have been implicated in cancer progression (16). And, the ratios of the intracellular PKA-I to PKA-II could regulate the secretion of ECPKA, a decrease in PKA-I by a mutant would down-regulate the ECPKA (11), while overexpression of PKA-I leads to the secretion of ECPKA (35). So, the oncogenic potential of PKA depends on which isoform is expressed, and it is difficult to confirm the relationship of PKACα in tumor tissues and its relation with the tumor stage. Of course, there are more questions needed to address, for example, the discrepancy of PKACα between cancer patients and patients with benign disease, such as polyps and ulcers should be evaluated. Besides, more attention should pay to the phenomenon and mechanism of PKACα secretion.
Conclusions
The ECPKA in the serum of gastric cancers was higher than the healthy people. ROC analyses evaluated the utility of PKACα for colorectal cancer and gastric cancer with higher sensitivities and specificities. Collectively, ECPKA may prove to be of great significance when used either alone or in combination with other biomarkers (such as CEA, CA199, etc.) or technologies for diagnosing and monitoring cancer. And targeting PKA, a kinase regulating cell metabolism, may provide a new way for tumor treatment.
Acknowledgments
Funding: This work was supported by
Footnote
Reporting Checklist: The authors have completed the REMARK reporting checklist. Available at http://dx.doi.org/10.21037/tcr-20-764
Data Sharing Statement: Available at http://dx.doi.org/10.21037/tcr-20-764
Conflicts of Interest: All authors have completed the ICMJE uniform disclosure form (available at http://dx.doi.org/10.21037/tcr-20-764). The authors have no conflicts of interest to declare.
Ethical Statement: The authors are accountable for all aspects of the work in ensuring that questions related to the accuracy or integrity of any part of the work are appropriately investigated and resolved. The study involving human subjects was approved by the Human Ethics Committee of School of Medicine, Jiangsu University. All patients provided informed consent. The study was conducted in accordance with the Declaration of Helsinki (as revised in 2013).
Open Access Statement: This is an Open Access article distributed in accordance with the Creative Commons Attribution-NonCommercial-NoDerivs 4.0 International License (CC BY-NC-ND 4.0), which permits the non-commercial replication and distribution of the article with the strict proviso that no changes or edits are made and the original work is properly cited (including links to both the formal publication through the relevant DOI and the license). See: https://creativecommons.org/licenses/by-nc-nd/4.0/.
References
- Harada K, Mizrak Kaya D, Shimodaira Y, et al. Translating genomic profiling to gastrointestinal cancer treatment. Future Oncol 2017;13:919-34. [Crossref] [PubMed]
- Athauda A, Segelov E, Ali Z, et al. Integrative molecular analysis of colorectal cancer and gastric cancer: What have we learnt? Cancer Treat Rev 2019;73:31-40. [Crossref] [PubMed]
- Tian SB, Yu JC, Kang WM, et al. Combined detection of CEA, CA 19-9, CA 242 and CA 50 in the diagnosis and prognosis of resectable gastric cancer. Asian Pac J Cancer Prev 2014;15:6295-300. [Crossref] [PubMed]
- Durães C, Almeida GM, Seruca R, et al. Biomarkers for gastric cancer: prognostic, predictive or targets of therapy? Virchows Arch 2014;464:367-78. [Crossref] [PubMed]
- Taskén K, Aandahl EM. Localized effects of cAMP mediated by distinct routes of protein kinase A. Physiol Rev 2004;84:137-67. [Crossref] [PubMed]
- Turnham RE, Scott JD. Protein kinase A catalytic subunit isoform PRKACA; History, function and physiology. Gene 2016;577:101-8. [Crossref] [PubMed]
- Sapio L, Di Maiolo F, Illiano M, et al. Targeting protein kinase A in cancer therapy: an update. EXCLI J 2014;13:843-55. [PubMed]
- Caretta A, Mucignat-Caretta C. Protein kinase a in cancer. Cancers (Basel) 2011;3:913-26. [Crossref] [PubMed]
- Cheung J, Ginter C, Cassidy M, et al. Structural insights into mis-regulation of protein kinase A in human tumors. Proc Natl Acad Sci U S A 2015;112:1374-9. [Crossref] [PubMed]
- Hussain M, Tang F, Liu J, et al. Dichotomous role of protein kinase A type I (PKAI) in the tumor microenvironment: a potential target for 'two-in-one' cancer chemoimmunotherapeutics. Cancer Lett 2015;369:9-19. [Crossref] [PubMed]
- Cho YS, Park YG, Lee YN, et al. Extracellular protein kinase A as a cancer biomarker: its expression by tumor cells and reversal by a myristate-lacking Calpha and RIIbeta subunit overexpression. Proc Natl Acad Sci U S A 2000;97:835-40. [Crossref] [PubMed]
- Espiard S, Ragazzon B, Bertherat J. Protein kinase A alterations in adrenocortical tumors. Horm Metab Res 2014;46:869-75. [Crossref] [PubMed]
- Skalhegg BS, Tasken K. Specificity in the cAMP/PKA signaling pathway. Differential expression,regulation, and subcellular localization of subunits of PKA. Front Biosci 2000;5:D678-93. [PubMed]
- Sands WA, Palmer TM. Regulating gene transcription in response to cyclic AMP elevation. Cell Signal 2008;20:460-6. [Crossref] [PubMed]
- Clegg RA, Gardner RA, Sumathipala RN, et al. Targeting of PKA in mammary epithelial cells. Mechanisms and functional consequences. Adv Exp Med Biol 2000;480:99-105. [Crossref] [PubMed]
- Wang H, Li M, Lin W, et al. Extracellular activity of cyclic AMP-dependent protein kinase as a biomarker for human cancer detection: distribution characteristics in a normal population and cancer patients. Cancer Epidemiol Biomarkers Prev 2007;16:789-95. [Crossref] [PubMed]
- Cvijic ME, Kita T, Shih W, et al. Extracellular catalytic subunit activity of the cAMP-dependent protein kinase in prostate cancer. Clin Cancer Res 2000;6:2309-17. [PubMed]
- Cho YS, Lee YN, Cho-Chung YS. Biochemical characterization of extracellular cAMP-dependent protein kinase as a tumor marker. Biochem Biophys Res Commun 2000;278:679-84. [Crossref] [PubMed]
- Kita T, Goydos J, Reitman E, et al. Extracellular cAMP-dependent protein kinase (ECPKA) in melanoma. Cancer Lett 2004;208:187-91. [Crossref] [PubMed]
- Moody SE, Schinzel AC, Singh S, et al. PRKACA mediates resistance to HER2-targeted therapy in breast cancer cells and restores anti-apoptotic signaling. Oncogene 2015;34:2061-71. [Crossref] [PubMed]
- Honeyman JN, Simon EP, Robine N, et al. Detection of a recurrent DNAJB1-PRKACA chimeric transcript in fibrolamellar hepatocellular carcinoma. Science 2014;343:1010-4. [Crossref] [PubMed]
- Nesterova MV, Johnson N, Cheadle C, et al. Autoantibody cancer biomarker: extracellular protein kinase A. Cancer Res 2006;66:8971-4. [Crossref] [PubMed]
- Szkudlarek M, Bosio RM, Wu Q, et al. Inhibition of angiogenesis by extracellular protein kinase A. Cancer Lett 2009;283:68-73. [Crossref] [PubMed]
- Bijlsma MF, Sadanandam A, Tan P, et al. Molecular subtypes in cancers of the gastrointestinal tract. Nat Rev Gastroenterol Hepatol 2017;14:333-42. [Crossref] [PubMed]
- Farran B, Muller S, Montenegro RC. Gastric cancer management: Kinases as a target therapy. Clin Exp Pharmacol Physiol 2017;44:613-22. [Crossref] [PubMed]
- Van Cutsem E, Kohne CH, Hitre E, et al. Cetuximab and chemotherapy as initial treatment for metastatic colorectal cancer. N Engl J Med 2009;360:1408-17. [Crossref] [PubMed]
- Verdaguer H, Sauri T, Macarulla T. Predictive and prognostic biomarkers in personalized gastrointestinal cancer treatment. J Gastrointest Oncol 2017;8:405-17. [Crossref] [PubMed]
- Carlomagno N, Incollingo P, Tammaro V, et al. Diagnostic, Predictive, Prognostic, and Therapeutic Molecular Biomarkers in Third Millennium: A Breakthrough in Gastric Cancer. Biomed Res Int 2017;2017:7869802.
- Wu P, Nielsen TE, Clausen MH. FDA-approved small-molecule kinase inhibitors. Trends Pharmacol Sci 2015;36:422-39. [Crossref] [PubMed]
- Walsh DA, Perkins JP, Krebs EG. An adenosine 3',5'-monophosphate-dependant protein kinase from rabbit skeletal muscle. J Biol Chem 1968;243:3763-5. [PubMed]
- Chin KV, Yang WL, Ravatn R, et al. Reinventing the wheel of cyclic AMP: novel mechanisms of cAMP signaling. Ann N Y Acad Sci 2002;968:49-64. [Crossref] [PubMed]
- Ben-Mahrez K, Sorokine I, Thierry D, et al. Circulating antibodies against c-myc oncogene product in sera of colorectal cancer patients. Int J Cancer 1990;46:35-8. [Crossref] [PubMed]
- Lubin R, Schlichtholz B, Bengoufa D, et al. Analysis of p53 antibodies in patients with various cancers define B-cell epitopes of human p53: distribution on primary structure and exposure on protein surface. Cancer Res 1993;53:5872-6. [PubMed]
- Ryu MO, Kim BG, Choi US, et al. Extracellular cyclic adenosine monophosphate-dependent protein kinase A autoantibody and C-reactive protein as serum biomarkers for diagnosis of cancer in dogs. Vet Comp Oncol 2019;17:99-106. [Crossref] [PubMed]
- Loilome W, Yooyuen S, Namwat N, et al. PRKAR1A overexpression is associated with increased ECPKA autoantibody in liver fluke-associated cholangiocarcinoma: application for assessment of the risk group. Tumour Biol 2012;33:2289-98. [Crossref] [PubMed]