Intracellular targeting of STIP1 inhibits human cancer cell line growth
Introduction
Extracellular and cell-surface molecules remain the most common druggable cancer targets (1-3). However, the use of intracellular therapeutic modalities is gaining momentum (4-6). In fact, a diverse group of oncoproteins involved in tumorigenesis are found in the cytoplasm and may serve as promising targets for novel anti-tumor therapeutic strategies (4,7,8).
Stress-induced phosphoprotein 1 (STIP1) is an adaptor protein that coordinates the functions of different chaperones, including heat shock proteins (HSPs), to facilitate protein folding within cells (9,10). Accumulating evidence indicates that STIP1, which is overexpressed in several malignancies, including liver, pancreatic, ovarian, colon, and breast tumors, plays a role in the pathogenesis of cancer (11-20). Notably, STIP1 expression has been detected not only on the cell surface but also in the cytosol (13,16), indicating both extracellular and intracellular STIP1 have oncogenic potential. In our previous study, we found that secreted STIP1 promoted the proliferation of ovarian cancer cells by activating the extracellular-regulated MAP kinases (ERK) or activin receptor-like kinase-2 (ALK2)-small mothers against the decapentaplegic (SMAD) signaling pathway (13,21). Conversely, intracellular STIP1 promotes the growth of ovarian cancer cells through two distinct mechanisms—the formation of an HSP90-janus kinase 2 (JAK2)-signal transducer and activator of transcription 3 (STAT3) complex and the glycogen synthase kinase-3 beta (GSK3β)-mediated phosphorylation of lysine-specific demethylase 1 (LSD1) (22,23). It has also been demonstrated that intracellular STIP1 mediates the heat-induced spread of hepatocellular carcinoma to distant sites by facilitating epithelial-mesenchymal transition via an increased nuclear shuttling of Snail1 (24). In addition, the exposure of cells to 4-(2-hydroxyethyl)-1-piperazineethanesulfonic acid (HEPES) is an effective means to promote the intracellular delivery of either antibodies or small peptides (25).
The present study represents a proof-of-concept investigation to test whether intracellular STIP1 serves as a druggable target in different human cancer cell lines. Two different strategies have been used to attain intracellular STIP1 inhibition: (I) HEPES-induced cytosolic delivery of anti-STIP1 antibodies, and (II) exposure of cells to a cell-penetrating peptide (denoted as peptide 520) capable of inhibiting STIP1.
We present the following article in accordance with the ARRIVE reporting checklist (available at http://dx.doi.org/10.21037/tcr-20-3333).
Methods
Cell cultures
Ovarian cancer cells (MDAH2774, TOV21G and ES2), cervical cancer cells (HeLa), breast cancer cells (MCF7), and lung adenocarcinoma cells (CL1-0) lines were obtained from the American Type Culture Collection (Manassas, VA, USA). Human HAP1 and STIP1 knockout HAP1 cell lines were purchased from Horizon Discovery (Campus Vienna Biocenter 3; Vienna, Austria). Non-cancerous cells-immortalized human endometrial stromal Cells (HESC) were purchased from Applied Biological Materials Inc. (abm) (Viking Way, Richmond, BC, V6V 2J5, Canada). Nasopharyngeal cancer cells (NPC-BM1), lung adenocarcinoma cells (CL1-0), colon cancer (HT29), tongue cancer cells (SAS), hepatocellular carcinoma cells (Huh7), and pancreatic cancer cells (BxPC3) were kindly provided by Dr. Chih-Ching Wu (Department of Medical Biotechnology and Laboratory Science, Chang Gung University, Taoyuan, Taiwan) whereas the luciferase-expressing murine ovarian cancer cells (MOSEC/Luc) was a gift from Dr. Chi-Long Chang (Institute of Biomedical Sciences, Mackay Medical College, Taiwan). MDAH2774, TOV21G, ES2, HeLa, MCF7, CL1-0 were cultured in Dulbecco’s Modified Eagle Medium (DMEM/F12 medium). BxPC3 and NPC-BM1, HT-29, MOSEC/Luc were grown in the Roswell Park Memorial Institute Medium (RPMI 1640 medium). SAS and Huh7 were maintained in the DMEM. Human HAP1 and STIP1 knockout HAP1 cells were cultured in Iscove-modified Dulbecco’s medium (IMEM medium). HESC were cultured in RPMI-1640 medium. All media were supplemented with 10% fetal bovine serum (FBS) and 1% penicillin and streptomycin, and were obtained from Invitrogen (Life Technologies, Carlsbad, CA, USA).
Antibodies, peptides, and chemicals
The following antibodies were used in the study: Alexa Fluor 546 anti-rabbit IgG (Thermo Scientific), Alexa Fluor 488 anti-mouse IgG (Thermo Scientific), glyceraldehyde-3-phosphate dehydrogenase (GAPDH), STIP1 and β-actin (Santa Cruz Biotechnology, Dallas, TX, USA), cleaved caspase-3, cleaved poly (ADP-ribose) polymerase (cleaved PARP) (Cell Signaling Technology, Danvers, MA, USA), LSD1 (Cell Signaling Technology, Danvers, MA, USA) and JAK2 (Abcam, Cambridge, UK). Peptide 520 (STIP1520–543: EHLKNPVIAQKIQKLMDVGLIAIR) and peptide 520 containing (D-arginine)8 were synthesized and purchased from GeneDireX (Las Vegas City, NE, USA) (23). Scramble peptide was also synthesized from GeneDireX according to the amino acid hydrophobicity of peptide 520. HEPES was obtained from Sigma-Aldrich (St. Louis, MO, USA). 4’, 6’-diamidino-2-phenylindole (DAPI) solution was obtained from Thermo Fisher Scientific (Waltham, MA, USA).
HEPES-mediated intracellular protein delivery
Cells (8×105) were plated in 6-cm dishes for 24 h. The culture media were subsequently replaced by Opti-MEM (Thermo Scientific, Waltham, MA, USA). Different proteins (7 µg), including antibodies and small peptides, were mixed with 400 µL of a pure HEPES (20 or 30 mM; pH 7.4) solution or Opti-MEM (negative control) for 15–30 min at room temperature. The transfection mixture was added to cultured cells in Opti-MEM without serum and incubated at 37 °C for 4–24 h. Briefly, the proteins and HEPES were mixed and incubated for 15 min, and the transfected proteins were detected using fluorescent microscopy after 24 h. The amount of individual components in the transfection mixtures was adjusted according to the size of the cultures. For example, when using a 6-well plate, 4 µg of protein were mixed with 200 µL of HEPES (25).
Immunofluorescent staining
After protein transfection, the cells in culture dishes were washed three times with phosphate-buffered saline (PBS) and fixed with 4% paraformaldehyde for 20 min. After washing with PBS, the cells were permeabilized with 0.1% Triton X-100 for 5 min and blocked with 10% bovine serum albumin for 30 min. In experiments involving Alexa Fluor 488 anti-mouse IgG (Thermo Scientific), the cells were stained with DAPI for 10 min. After DAPI staining, the slides were washed with PBS three times for 5 min. Fixed cell images were acquired using a confocal microscope with 63× object lens at excitation and emission wavelengths of 488 and 500–535 nm, respectively (Leica, Wetzlar, Germany).
Western blot analysis
Cells were lysed in a combination of radioimmunoprecipitation assay buffer (150 mM NaCl, 20 mM Tris-HCl (pH 7.5), 1% Triton X-100, 1% NP-40, 0.1% sodium dodecyl sulphate (SDS), and 0.5% deoxycholate) and protease/phosphatase inhibitors (Bionovas, Toronto, Canada). The Bradford assay (Bio-Rad, Hercules, CA, USA) was used to determine the total protein concentration. Each sample (50 µg of proteins for each) was subjected to SDS–polyacrylamide gel electrophoresis (SDS-PAGE). The resolved proteins were transferred onto a nitrocellulose membrane and labeled proteins were visualized using an enhanced chemiluminescence assay (Millipore, Bedford, MA, USA).
Cell viability and cytotoxicity assays
Different human cancer cell lines were cultured on 96-well plates, and either (I) subjected to HEPES-mediated intracellular delivery of anti-STIP1 (Abnova; Cat. No: H00010963-M02), (Abnova; Cat. No: H00010963-M35) or control IgG2 (Abnova; Cat. No: PAB14685-A01P) antibodies, or (II) exposed to cell-penetrating peptide 520 (23). Following incubation for 24 h, the cells were centrifuged at 300 ×g for 5 min, the supernatant was discarded, and the cells attached to the plates were analyzed using a commercially available [3-(4,5-Dimethylthiazol-2-yl)-2,5-diphenyltetrazolium bromide] (MTT) assay (Promega, Madison, WI, USA). Cell toxicity was assessed using the lactate dehydrogenase (LDH) method (Sigma-Aldrich) (25). Since the MTT assay is more sensitive than the LDH assay (26,27), the cells subjected to transfection were incubated for 48 h and centrifuged at 300 ×g for 5 min. The resulting supernatants were collected and analyzed using a commercially available LDH assay. The MTT and LDH signals were quantified using an enzyme-linked immunosorbent assay reader (Tecan Systems Inc., San Jose, CA, USA).
Animal experiments
Ten-week-old female immunocompetent C57BL/6 mice were about 20 g and obtained from the National Animal Center (Taipei, Taiwan) and all animal experiment procedures carried out in Laboratory Animal Center, Linkou Chang Gung Memorial Hospital (Taoyuan, Taiwan). Mice were intraperitoneally injected with stably luciferase-expressed C57BL/6 MOSEC/Luc cells (quantity: 1×106). C57BL/6 mice bearing MOSEC/Luc cells were randomly divided into two groups. Each treatment group consisted of 4 mice. After one-week, anti-STIP1 or control IgG antibodies pre incubated with 30 mM HEPES (100 µg antibodies/50 µL 30 mM HEPES) for 15 minutes then intravenously injected three times per week until sacrifice. The mice were sacrificed and the presence of tumors and ascites was determined. D-luciferin—the luciferase substrate—was injected intraperitoneally (75 mg/kg body weight) to monitor experimental tumor growth over time. Bioluminescent tumor images were visualized on an IVIS imaging system (Xenogen Corp., Alameda, CA, USA) and analyzed with the Living Image software (Xenogen Corp.).
Experiments were performed under a project license (NO.: 2019062502) granted by the Institutional Animal Care and Use Committee of the Chang Gung Memorial Hospital, in compliance with the tenets of the Guide for the Care and Use of Laboratory Animals published by the US National Institutes of Health (NIH Publication No. 85−23, revised 1996).
Statistical analysis
Continuous data are presented as the mean ± standard error of the mean (SEM). The results were compared using Student’s t-test or the Mann-Whitney U test, as appropriate. All calculations were performed in Graph Pad Prism software (version, 5.01; Graph Pad Inc., San Diego, CA, USA). Survival curves were plotted with the Kaplan-Meier method and compared with the log-rank test. Two-tailed P values <0.05 were considered statistically significant.
Results
Intracellular delivery of anti-STIP1 antibodies successfully targets STIP1 within cancer cells
Anti-STIP1 antibodies were delivered into cancer cells using the HEPES method (25) and subsequently stained with Alexa Fluor 488-conjugated secondary antibodies. The intracellular penetration of antibodies induced by HEPES was confirmed in ovarian cancer cells (MDAH2774) using confocal microscopy (Figure 1A). We then investigated the effects of STIP1 in STIP1-deficient HAP1 cells (knockout, KO). STIP1-expressing HAP1 cells (wild-type, WT) exhibited a higher viability and a lower extent of toxicity than STIP1 KO did, confirming the role of STIP1 in tumorigenesis. Cleaved poly ADP-ribose polymerase (PARP) and cleaved caspase 3, two markers of apoptosis, were also upregulated in STIP1 KO cells (Figure 1B,C). Taken together, these results indicate that the intracellular delivery of anti-STIP1 antibodies successfully targets STIP1, a molecule that promotes the viability of cancer cells.
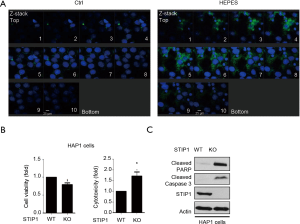
Intracellular delivery of anti-STIP1 antibodies induces cell death in different cancer cell lines
Anti-STIP1 antibodies were delivered into different STIP1-overexpressing malignant cells, including pancreatic cancer (BxPC3), head and neck cancer (NPC-BM1), lung cancer (CL1-0), breast cancer (MCF7), colon cancer (HT29), and ovarian cancer (ES2 and MDAH2774) cells. The intracellular delivery of anti-STIP1 consistently exerted cytotoxic effects in all the cancer cell lines (Figure 2A,B,C,D,E,F,G). Moreover, we observed an increased expression of the apoptotic marker cleaved caspase-3 in MDAH2774, TOV21G and ES2 cells (Figure 2H). Taken together, these results indicated that the HEPES-induced intracellular delivery of anti-STIP1 antibodies inhibits human cancer cell line growth. Moreover, an additional second anti-STIP1 antibody (Abnova; Cat. No: H00010963-M35) was used to perform the experiments of STIP1-mediated cell death in ovarian cancer ES2 cells (Figure S1A). In order to make sure that specificity for cancer cells, we used non-cancerous cells-immortalized human endometrial stromal cells (HESC) to perform the experiments of STIP1-mediated cell death. When treated with anti-STIP1 antibody (Abnova; Cat. No: H00010963-M02 and Cat. No: H00010963-M35) in HESC, there was no change in cell viability or cell cytotoxicity (Figure S1B). Therefore, anti-STIP1 antibody has the potential to develop into anticancer treatment due to its specificity towards tumor cells that express higher STIP1 (Figure S1C).
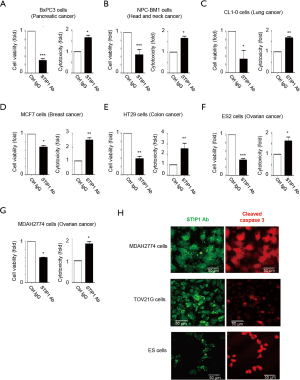
Spontaneous internalization of cell-penetrating peptide 520, an STIP1 inhibitor, inhibits human cancer cell line growth
Peptide 520, a (D-arginine)8-tagged STIP1520–543 peptide, is capable of spontaneously entering cells and is known to inhibit the viability of ovarian cancer cells (23). Peptide 520 was internalized spontaneously by pancreatic cancer (BxPC3), head and neck cancer (NPC-BM1), hepatocellular carcinoma (Huh7) cells, breast cancer (MCF7), and tongue cancer (SAS) cells (Figure 3). The internalization of peptide 520 reduced viability and increased toxicity in cancer cell lines.
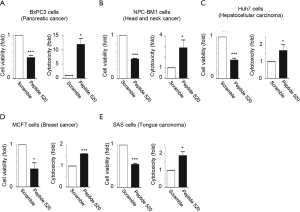
Intracellular delivery of anti-STIP1 antibodies leads to the degradation of two client proteins of STIP1, LSD1 and JAK2
STIP1 inhibition is known to promote the catabolism of LSD1 and JAK2 (22,23) and TRIM21 (tripartite motif-containing protein 21) has been reported that it mediated degradation of targeted proteins (28). We performed the intracellular delivery of anti-STIP1 antibodies into TRIM21-expressing HeLa cells consistently decreased the expression of STIP1 and its client proteins, LSD1 and JAK2 (Figure 4). Taken together, these results indicated that the intracellular delivery of anti-STIP1 antibodies can alter STIP1 downstream oncogenic signaling.
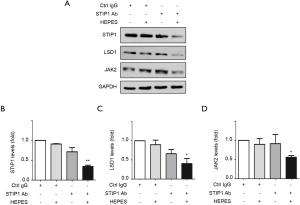
In vivo administration of anti-STIP1 antibodies reduces mortality in mice bearing experimental tumors.
To assess the feasibility of targeting STIP1 in vivo, C57BL/6 mice were intraperitoneally injected with stably luciferase-expressing C57BL/6 MOSEC/Luc cells followed by the intravenous administration of either anti-STIP1 antibodies or control IgG antibodies-HEPES mixture (100 µg antibodies/50 µL 30 mM HEPES). The results revealed that intravenous administration of anti-STIP1 antibodies group decreased tumor size and reduced mortality in mice bearing experimental tumors (P=0.06; log-rank test; Figure 5A,B).
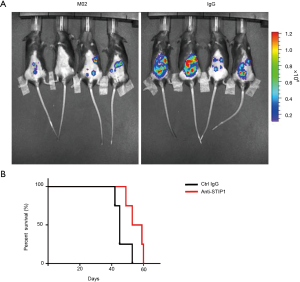
Discussion
The results of our study demonstrated that the intracellular targeting of STIP1, attained either via the HEPES-mediated cytosolic delivery of anti-STIP1 antibodies or the use of an inhibitory peptide, effectively inhibits human cancer cell line growth. Mechanistically, the intracellular targeting of STIP1 was found to alter downstream oncogenic signaling pathways (involving LSD1 and JAK2 proteins), ultimately resulting in cell death and apoptosis both in vitro (22,23). The detection of anti-STIP1 antibodies in cancer cells was demonstrated by staining of Alexa Fluor 488 anti-mouse IgG antibodies. Our data provide a basis for the further investigation of STIP1 as an intracellular cancer target, and support the notion that inhibiting intracellular molecules involved in carcinogenesis may be a valuable antitumor strategy (4,7,8,29,30).
STIP1 has been shown to be secreted in the extracellular space, where it promotes tumor growth and metastasis through the activation of various signaling cascades (13,21,31). However, STIP1 is also expressed in the cytoplasm of neoplastic cells (22,23,25), where it elicits numerous cancer growth signaling pathways, including the formation of the HSP90-JAK2-STAT3 complex, the GSK3β-LSD1 pathway, and the nuclear shuttling of Snail1 (22,23,25). The knockdown of STIP1 in pancreatic, ovarian, or gastric cancers has been shown to decrease cancer cell invasiveness and migration capacity via the inhibition of matrix metalloprotease-2 and Wnt/β-catenin signaling (12,14,32,33), as well as via the promotion of apoptotic cell death (34). Interestingly, the intracellular targeting of STIP1 in our study led to an increased expression of apoptotic markers (cleaved PARP and cleaved caspase 3) in STIP1 KO HAP-1 cells.
Traditional approaches used for the targeting of intracellular molecules as an anticancer strategy include the use of signal molecules fused with peptides (7). Human serum albumin, nanoparticles, and gelatin-based polymers are useful vehicles to facilitate the intracellular delivery of therapeutic antibodies (5). In this study, we demonstrated that the use of HEPES is suitable for promoting the penetration of a therapeutic antibody into the cytosol of malignant cells. These results contribute to paving the way for a future large-scale adoption of this methodological approach in preclinical studies of antibody-based intracellular therapeutic modalities in cancer. As an alternative approach to the intracellular delivery of STIP1 antibodies, the use of a cell-penetrating inhibitory peptide is effective at targeting STIP1 in malignant cells. We have previously shown that (D-arginine)8-tagged peptide 520 is able to enter the cytosol of ovarian cancer cells and block cell growth by disrupting the oncogenic HSP90-JAK2-STAT3 signaling pathway (23). Here, we expanded our previous findings by showing that peptide 520 effectively inhibits the in vitro growth of numerous other cancer cell lines from pancreatic, head and neck, breast, tongue, and liver malignancies.
Although we cannot rule out that anti-STIP1 antibodies can bind to STIP1 expressed on the cell surface, we believe that intracellular targeting of STIP1 is of major importance. Furthermore, a head-to-head comparison of the bioavailability of anti-STIP1 antibody and peptide 520 for targeting intracellular STIP1 should be addressed in future investigations. The role of STIP1 expression in different malignancies is also needed to determine the site-specificity of the tumor response.
Further preclinical research efforts are required to fully unravel the complexity of therapeutic responses of cancer cells to intracellular STIP1 inhibition.
In conclusion, our results provide a basis for further investigation of STIP1 as a promising anti-tumor therapeutic strategy. We demonstrated the feasibility of targeting intracellular STIP1 using anti-STIP1 antibody or peptide 520 as a potential anticancer strategy.
Acknowledgments
The authors would like to express their gratitude to the Microscopy Core Laboratory and the Genomic Medicine Core Laboratory and Laboratory Animal Center of Chang Gung Memorial Hospital for sharing their expertise.
Funding: This work was financially supported by grants from
Footnote
Reporting Checklist: The authors have completed the ARRIVE reporting checklist. Available at http://dx.doi.org/10.21037/tcr-20-3333
Data Sharing Statement: Available at http://dx.doi.org/10.21037/tcr-20-3333
Conflicts of Interest: All authors have completed the ICMJE uniform disclosure form (available at http://dx.doi.org/10.21037/tcr-20-3333). The authors have no conflicts of interest to declare.
Ethical Statement: The authors are accountable for all aspects of the work in ensuring that questions related to the accuracy or integrity of any part of the work are appropriately investigated and resolved. Experiments were performed under a project license (NO.: 2019062502) granted by the Institutional Animal Care and Use Committee of the Chang Gung Memorial Hospital, in compliance with the tenets of the Guide for the Care and Use of Laboratory Animals published by the US National Institutes of Health (NIH Publication No. 85−23, revised 1996).
Open Access Statement: This is an Open Access article distributed in accordance with the Creative Commons Attribution-NonCommercial-NoDerivs 4.0 International License (CC BY-NC-ND 4.0), which permits the non-commercial replication and distribution of the article with the strict proviso that no changes or edits are made and the original work is properly cited (including links to both the formal publication through the relevant DOI and the license). See: https://creativecommons.org/licenses/by-nc-nd/4.0/.
References
- Leader B, Baca QJ, Golan DE. Protein therapeutics: a summary and pharmacological classification. Nat Rev Drug Discov 2008;7:21-39. [Crossref] [PubMed]
- Smaglo BG, Aldeghaither D, Weiner LM. The development of immunoconjugates for targeted cancer therapy. Nat Rev Clin Oncol 2014;11:637-48. [Crossref] [PubMed]
- Weiner LM, Murray JC, Shuptrine CW. Antibody-based immunotherapy of cancer. Cell 2012;148:1081-4. [Crossref] [PubMed]
- Guo K, Li J, Tang JP, et al. Targeting intracellular oncoproteins with antibody therapy or vaccination. Sci Transl Med 2011;3:99ra85. [Crossref] [PubMed]
- Yin L, Yuvienco C, Montclare JK. Protein based therapeutic delivery agents: Contemporary developments and challenges. Biomaterials 2017;134:91-116. [Crossref] [PubMed]
- Battogtokh G, Cho YY, Lee JY, et al. Mitochondrial-Targeting Anticancer Agent Conjugates and Nanocarrier Systems for Cancer Treatment. Front Pharmacol 2018;9:922. [Crossref] [PubMed]
- Jameson KL, Mazur PK, Zehnder AM, et al. IQGAP1 scaffold-kinase interaction blockade selectively targets RAS-MAP kinase-driven tumors. Nat Med 2013;19:626-30. [Crossref] [PubMed]
- Dao T, Yan S, Veomett N, et al. Targeting the intracellular WT1 oncogene product with a therapeutic human antibody. Sci Transl Med 2013;5:176ra33. [Crossref] [PubMed]
- Schmid AB, Lagleder S, Grawert MA, et al. The architecture of functional modules in the Hsp90 co-chaperone Sti1/Hop. Embo J 2012;31:1506-17. [Crossref] [PubMed]
- Blair LJ, Genest O, Mollapour M. The multiple facets of the Hsp90 machine. Nat Struct Mol Biol 2019;26:92-5. [Crossref] [PubMed]
- Sun W, Xing B, Sun Y, et al. Proteome analysis of hepatocellular carcinoma by two-dimensional difference gel electrophoresis: novel protein markers in hepatocellular carcinoma tissues. Mol Cell Proteomics 2007;6:1798-808. [Crossref] [PubMed]
- Walsh N, O'Donovan N, Kennedy S, et al. Identification of pancreatic cancer invasion-related proteins by proteomic analysis. Proteome Sci 2009;7:3. [Crossref] [PubMed]
- Wang TH, Chao A, Tsai CL, et al. Stress-induced phosphoprotein 1 as a secreted biomarker for human ovarian cancer promotes cancer cell proliferation. Mol Cell Proteomics 2010;9:1873-84. [Crossref] [PubMed]
- Chao A, Lai CH, Tsai CL, et al. Tumor stress-induced phosphoprotein1 (STIP1) as a prognostic biomarker in ovarian cancer. PLoS One 2013;8:e57084. [Crossref] [PubMed]
- Kubota H, Yamamoto S, Itoh E, et al. Increased expression of co-chaperone HOP with HSP90 and HSC70 and complex formation in human colonic carcinoma. Cell Stress Chaperones 2010;15:1003-11. [Crossref] [PubMed]
- Van Simaeys D, Turek D, Champanhac C, et al. Identification of cell membrane protein stress-induced phosphoprotein 1 as a potential ovarian cancer biomarker using aptamers selected by cell systematic evolution of ligands by exponential enrichment. Anal Chem 2014;86:4521-7. [Crossref] [PubMed]
- Wu R, Liu F, Peng P, et al. Tumor stress-induced phosphoprotein 1 as a prognostic biomarker for breast cancer. Ann Transl Med 2018;6:302. [Crossref] [PubMed]
- Luo X, Liu Y, Ma S, et al. STIP1 is over-expressed in hepatocellular carcinoma and promotes the growth and migration of cancer cells. Gene 2018;662:110-7. [Crossref] [PubMed]
- Zhang S, Shao J, Su F. Prognostic significance of STIP1 expression in human cancer: A meta-analysis. Clin Chim Acta 2018;486:168-76. [Crossref] [PubMed]
- Zhang Z, Ren H, Yang L, et al. Aberrant expression of stress-induced phosphoprotein 1 in colorectal cancer and its clinicopathologic significance. Hum Pathol 2018;79:135-43. [Crossref] [PubMed]
- Tsai CL, Tsai CN, Lin CY, et al. Secreted stress-induced phosphoprotein 1 activates the ALK2-SMAD signaling pathways and promotes cell proliferation of ovarian cancer cells. Cell Rep 2012;2:283-93. [Crossref] [PubMed]
- Tsai CL, Chao AS, Jung SM, et al. Stress-induced phosphoprotein 1 acts as a scaffold protein for glycogen synthase kinase-3 beta-mediated phosphorylation of lysine-specific demethylase 1. Oncogenesis 2018;7:31. [Crossref] [PubMed]
- Tsai CL, Chao A, Jung SM, et al. Stress-induced phosphoprotein-1 maintains the stability of JAK2 in cancer cells. Oncotarget 2016;7:50548-63. [Crossref] [PubMed]
- Su T, Liao J, Dai Z, et al. Stress-induced phosphoprotein 1 mediates hepatocellular carcinoma metastasis after insufficient radiofrequency ablation. Oncogene 2018;37:3514-27. [Crossref] [PubMed]
- Chen SH, Chao A, Tsai CL, et al. Utilization of HEPES for Enhancing Protein Transfection into Mammalian Cells. Mol Ther Methods Clin Dev 2018;13:99-111. [Crossref] [PubMed]
- Fotakis G, Timbrell JA. In vitro cytotoxicity assays: comparison of LDH, neutral red, MTT and protein assay in hepatoma cell lines following exposure to cadmium chloride. Toxicol Lett 2006;160:171-7. [Crossref] [PubMed]
- Lobner D. Comparison of the LDH and MTT assays for quantifying cell death: validity for neuronal apoptosis? J Neurosci Methods 2000;96:147-52. [Crossref] [PubMed]
- Clift D, McEwan WA, Labzin LI, et al. A Method for the Acute and Rapid Degradation of Endogenous Proteins. Cell 2017;171:1692-706.e18. [Crossref] [PubMed]
- Veomett N, Dao T, Liu H, et al. Therapeutic efficacy of an Fc-enhanced TCR-like antibody to the intracellular WT1 oncoprotein. Clin Cancer Res 2014;20:4036-46. [Crossref] [PubMed]
- Dao T, Pankov D, Scott A, et al. Therapeutic bispecific T-cell engager antibody targeting the intracellular oncoprotein WT1. Nat Biotechnol 2015;33:1079-86. [Crossref] [PubMed]
- Wang J, You H, Qi J, et al. Autocrine and paracrine STIP1 signaling promote osteolytic bone metastasis in renal cell carcinoma. Oncotarget 2017;8:17012-26. [Crossref] [PubMed]
- Walsh N, Larkin A, Swan N, et al. RNAi knockdown of Hop (Hsp70/Hsp90 organising protein) decreases invasion via MMP-2 down regulation. Cancer Lett 2011;306:180-9. [Crossref] [PubMed]
- Huang L, Zhai E, Cai S, et al. Stress-inducible Protein-1 promotes metastasis of gastric cancer via Wnt/beta-catenin signaling pathway. J Exp Clin Cancer Res 2018;37:6. [Crossref] [PubMed]
- Beraldo FH, Soares IN, Goncalves DF, et al. Stress-inducible phosphoprotein 1 has unique cochaperone activity during development and regulates cellular response to ischemia via the prion protein. FASEB J 2013;27:3594-607. [Crossref] [PubMed]