Lung stereotactic body radiotherapy (SBRT): a single institution’s outcomes and methodology in the context of a literature review
Background
Lung cancer is the leading cause of cancer death in the United States and worldwide, representing 13-14% of new cancers but 26-28% of deaths from cancer in the US in 2015 (1). Approximately 75% of lung cancer is non-small cell histology (NSCLC), of which up to 25% of patients present with early stage disease. Despite lung cancer afflicting mostly elderly patients, stage I-II NSCLC still portends as little as 5-10% overall survival (OS) at 5 years, compared to 50-80% 5-year OS with therapy. The standard of care for early stage lung cancer has historically been surgical resection due to a randomized clinical trial from the 1960s in which surgery improved OS compared to radiotherapy (RT) (2). RT has since then been reserved for patients who are medically inoperable or decline an invasive procedure, which is usually due to risk of complications. Primary RT in this setting using 3-dimensional conformal techniques (3D-CRT) has continued to show suboptimal results, with 3-year local control of 30-50% and 5-year OS of 15-30% (3-6), largely because dose escalation in conventionally fractionated RT is limited by surrounding normal tissue toxicity.
Stereotactic body radiotherapy (SBRT), also known as stereotactic ablative radiotherapy (SABR), was first applied extracranially at Indiana University in 2000 (7). By immobilizing the patient in a body frame, the margin required to account for tumor motion and patient setup error could be decreased, therefore enabling dose escalation to a highly conformal target. The RTOG undertook a phase II study in which medically inoperable patients with stage I NSCLC were treated with SBRT and showed 3-year OS of 55% and local control over 90%, a significant improvement on outcomes with 3D-CRT (8). Other groups have reported comparable results (9), and subsequent analyses showed that achieving a biologically effective dose using an α/β ratio of 10 Gy (BED10) >100 Gy optimized tumor control (10). Further developments in imaging have enabled real-time tumor tracking to further reduce treatment target volume. A recent pooled analysis of two randomized clinical trials of SBRT compared to lobectomy for stage I (<4 cm) NSCLC found that SBRT was at least as effective as surgery, with 3-year OS of 95% (11).
Meanwhile, data has emerged suggesting that treatment of oligometastatic disease to the lung could improve patient outcomes. In the early 1990s, the International Registry of Lung Metastases collected data on 5,206 patients treated with lung metastasectomy in major thoracic centers in both Europe and the United States over the prior four decades, and reported 5-year OS of 36% in patients achieving complete resection (12). With subsequent improvements in systemic therapy coupled with increased efficacy and safety of lung RT as a non-invasive alternative to surgery, lung SBRT has recently been applied in the setting of limited intrapulmonary metastases, with initial publication of phase I/II studies showing efficacy (13).
In January 2007, the University of California San Diego’s (UCSD) Department of Radiation Oncology implemented a frameless image-guided lung SBRT program for the treatment of both primary NSCLC and oligometastatic intrapulmonary disease. In this article we will review (I) our center’s general approach to management including our experience with clinical outcomes and toxicity in the context of a review of the literature; (II) our preferred technique (including simulation and real-time tumor tracking); as well as (III) our results and strategy for patient follow-up using PET to monitor tumor response in the post-SBRT setting.
General approach to management
Our practice at UCSD has been to offer SBRT for patients with early stage primary NSCLC that are medically inoperable or who refuse surgical resection. The vast majority of these patients have stage I tumors (T1-T2aN0M0) that are biopsy-proven and PET-negative for nodal involvement, though we have also treated many patients with multiple primary lung cancers (MPLCs) (see Table 1 for summary of published results). While most patients have disease in the lung periphery, up to 30% of patients have central lesions. This reflects the histology of tumors in our patient population, such that the majority (60-70%) of NSCLCs are adenocarcinomas and the minority are squamous cell carcinomas. We also have routinely treated patients with oligometastatic disease to the lung, though these lesions are more often peripheral (over 90%) to minimize the risk of toxicity (21). Our typical RT dose and fractionation is 48 Gy in 4 fractions, with larger and/or central lesions receiving a lower dose of 50 Gy in 5 fractions to reduce possible risk of complications while still achieving BED10 of 100 Gy (see Table 2 for fractionation regimens and BED) as has previously been shown to improve tumor control in the setting of primary NSCLC (10,22). Our dose for oligometastatic lesions has historically been more conservative at 50 Gy in 5 fractions, though we are recently moving toward dose escalation in an attempt to improve local control in the absence of evidence showing increased toxicity. Most recently we have treated elderly patients with very small (T1a, ≤2 cm) peripheral lesions to 30-34 Gy in a single fraction per recent publications showing safety and efficacy from the Cleveland Clinic (23), though our data are not yet published. Patients were followed with clinical exam and imaging (usually chest CT) every 2-6 months. If there was concern on CT image for growth of the lesion, a PET was performed. Local tumor control was defined by biopsy confirming viable tumor or radiographic evidence of increasing size of lesion on follow-up imaging, FDG uptake on PET (if available), and tumor board consensus.
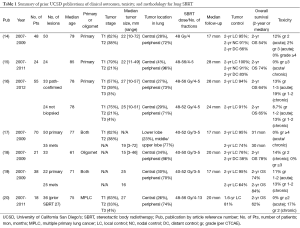
Full table
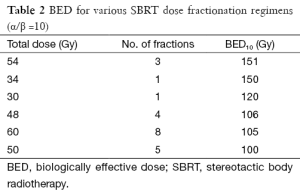
Full table
Clinical outcomes and toxicity
Primary non-small cell lung cancer (NSCLC)
For primary NSCLC with mean follow-up of 17-28 months, UCSD has reported 2-year actuarial local control of 91-100% and 2-year OS of 54-74% (14-16). Our studies included primarily T1-T2 lesions, though one patient with T3N0 was included (16). Larger tumor size was associated with inferior rates of distant control (14,16), which is similar to findings from other studies (8,9). We did not, however, find a difference in local control based on tumor size, though this may be due to our small median tumor size (<3 cm) (14). Larger series with greater numbers of tumors >3 cm have shown that larger tumor size (as well as T stage) is associated with decreased local control (8,24). At UCSD, we have employed our technique using 48 Gy in 4 fractions or 50 Gy in 5 fractions in several patients with tumors measuring >5 cm, and our anecdotal experience is that local control rates are lower when compared to smaller lesions treated to similar doses. Increased incidence of local recurrence in larger lesions is not unique to SBRT, but is also reported after surgical resection even in the setting of negative margins (12). Therefore, whether this higher failure rate is strictly due to insufficient radiation dose or also pathologic factors associated with larger lesions including satellite tumor cells or microscopic tumor spread is unclear (25).
Histologic subtype of NSCLC in early stage lung cancer appears to affect distant control, with RTOG 0236 prospectively showing 3-year disseminated recurrence in 5.9% of squamous cell carcinomas compared to 30.7% of non-squamous histology (8). Systemic therapy options have reflected this difference in biology, with pemetrexed chemotherapy and newer biologic agents targeting EGFR and ALK mutations showing greater efficacy in adenocarcinomas (especially those with identified mutations) compared to squamous cell carcinomas. However, the role of histology in primary tumor control is less clear, especially for small lesions where the local control rates often exceed 95%. While most studies do not specifically report local control based on histology, one retrospective study from Japan showed no difference in long-term local control, despite more rapid initial tumor shrinkage in lesions of squamous histology compared to adenocarcinomas of comparable size (26). This is likely due to the ablative nature of high doses of radiation employed during each fraction of SBRT, which engages different radiobiologic mechanisms for tumor cell damage that rely less on the linear quadratic equation and alpha/beta ratio that drive the relative radiosensitivity of squamous cell carcinomas. For larger lesions, however, we postulate that histology could play a role in local recurrence. In a pathologic study of mostly stage I NSCLC, adenocarcinomas evaluated at time of surgical pathology review had on average 2.5 mm of microscopic extension compared to only 1.1 mm in squamous cell carcinomas when matched for tumor diameter (27). It is therefore likely that tailoring local therapy better to histology, as well as biology such as mutational status, could result in improved local control.
Tumor location is primarily notable for increased risk of toxicity due to proximity to organs at risk (OAR). In one of the early publications of results with lung SBRT for early stage primary NSCLC, Indiana University reported increased grade 3-5 toxicity with treatment of central lesions (28), but this was in the setting of high doses of RT (60-66 Gy in 3 fractions). With data that BED10 ≥100 Gy provides excellent tumor control, we have employed 48 Gy in 4 fractions or 50 Gy in 5 fractions for large central lesions with minimal toxicity, reporting only one case of grade 3 esophagitis (14) and no other significant toxicity specific to central tumors. Peripheral lesions are at increased risk of rib fracture which was observed but not life threatening (17). While local failure is generally very low in patients with primary NSCLC in our studies so numbers are small, we did report local recurrence in 19% of central tumors (3 of 16 lesions) compared to only 2% in peripheral lesions (1 of 41) (16). There is conflicting evidence in the literature regarding local control of central tumors with some reports showing increased local failure rates (24), while others do not (29,30). The Cleveland Clinic recently reported very good local control (91.5% at 1.5 years) in lesions that were both large (>5 cm) and primarily central (68%) though this could also be explained by the predominance of squamous histology (67%) which may, in large lesions, be more radiosensitive and require narrower margins (29). Most studies of SBRT reflect the modern era of early stage lung cancer in which adenocarcinoma histology predominates due to the slow decline in smoking rates.
We initially found that younger age (<70 years) was a significant predictor of distant failure (14), though age did not correlate with worse OS in a Cox regression model when lesion size was accounted for. We subsequently reported a subset analysis of octogenarians which showed a higher 2-year OS of 74%, though these patients had slightly smaller tumors that were located more peripherally (96% vs. 4% centrally located) (15). While these patients could have also been more heavily selected for having fewer comorbidities, the cumulative risk of competing mortality was comparable to our prior publication (13% vs. 15%, respectively) (14). Other centers have also not reported differences in local control based on age alone (30). We reported no grade 3 or 4 toxicity in this elderly population (15). Therefore, the aggressive nature of even early stage NSCLC coupled with the low risk of toxicity warrants treatment with SBRT in elderly patients.
We have also reported results comparing lung SBRT for primary lung cancer in the setting of presence or absence of pathologic confirmation and found no difference in local control or OS (16). Patients were well-matched for age, smoking history, and reason for receiving SBRT instead of surgery. Though patients with pathologically-confirmed lesions did have slightly earlier tumor stage than those without pathologic confirmation (T1 57% vs. 75%, respectively), mean tumor size was comparable (2.7 vs. 2.5 cm, respectively). There were no differences seen in 2-year local control (94% vs. 91%) or 2-year OS (64% vs. 65%) for patients with or without pathologic confirmation, respectively. Patients without pathologic confirmation were diagnosed radiographically based on progressive growth on CT scan or presence of hypermetabolic activity on PET/CT, with malignancy defined as maximum standardized uptake value SUV ≥2.5.
Intrapulmonary oligometastases
Our local control rates using SBRT for metastases to the lung is slightly lower than definitive SBRT for early stage primary NSCLC, with 2-year local control rates of 74-76% (17-19). This is consistent with prior reports (31), in which the limitation of SBRT is hypothesized to be dose, based on the observation that the histologies of many primaries that metastasize to lung are less radiosensitive than NSCLC. Our dataset was too small to perform subset analysis by histology, but 27% of the lesions were metastases from sarcoma or melanoma primaries, which generally have lower rates of local control with RT and may benefit from further dose escalation (18,32). Meanwhile, a recent retrospective review from Stanford found that colorectal primary was the most significant predictor of local failure in patients with oligometastatic disease (33), and 23% of metastatic lesions in our study were colorectal in origin. Given that studies showing BED10 >100 Gy were done in primary NSCLC, it seems reasonable to consider dose escalation in these patients, particularly for peripheral lesions. Another potential factor reducing the efficacy of SBRT in intrapulmonary metastases is local microscopic tumor spread, which contributes to positive margins in patients undergoing surgical resection, or lung metastasectomy, and is known to increase the risk of local recurrence (12).
In our study, local control greater than 12 months was significantly associated with improved distant control, and progression free survival was improved with a single lung metastasis compared to two or more lesions in the lung. These results are comparable to a large surgical series from the International Registry of Lung Metastases, in which 5,206 patients were treated with pulmonary metastasectomy (12). Our reported 2-year OS was 78% with median survival was 30 months, which are comparable to reports from other series (32,33). These survival rates often exceed those in early stage NSCLC, primarily due to relatively advanced age and comorbidities of patients diagnosed with NSCLC and undergoing SBRT, but further highlight the importance of durable local control in appropriately selected patients with oligometastatic disease. Multiple studies are ongoing to assess the role of SBRT to oligometastatic disease in extending survival.
Multiple primary lung cancers (MPLCs)
We have also employed SBRT in the treatment of MPLCs, which accounts for up to 4% of NSCLC (34). Our study of 18 patients included 6 patients with synchronous tumors and 12 patients with metachronous tumors, of which 27 lesions were treated with SBRT, 6 lesions were treated with prior fractionated RT, and 3 lesions had previously been resected (20). The vast majority of these patients (89%) were determined to be poor surgical candidates due to comorbid medical conditions and/or poor pulmonary reserve at time of SBRT. With median follow-up of 20 months, observed local control was 81%, and 2-year actuarial OS was 62%. Three of 6 deceased patients had developed metastatic disease. The challenge in MPLC is confirming the diagnosis of multiple primaries rather than metastases even from primary lung cancer. Criteria used still include those outlined by Martini and Melamed (35), though diagnosis is even more difficult in inoperable patients that we encounter for SBRT. Nonetheless, our local control was at least as good as what we have reported with treating single metastatic lesions in the lung (17,18).
No acute grade ≥2 toxicity was observed in our patients with MPLCs treated with SBRT. Clinical pneumonitis, as defined by cough and evidence of inflammation in the appropriate region on chest CT, was observed in 3 of 18 patients (17%) at 3, 4 and 8 months following completion of RT, and was the only late toxicity reported (20). Two of the 3 patients that developed pneumonitis had two centrally-located primary tumors. Surgical studies have noted that squamous tumors are more often centrally located and associated with a local environment that is characterized by inflammatory changes (27), so it is postulated that histology more than central location drives risk of subsequent radiation pneumonitis. Fortunately symptoms resolved in all patients with administration of steroids.
Lung stereotactic body radiotherapy (SBRT) technique
Since the inception of UCSD’s lung SBRT program in 2007, we have made several iterations to our technique. Here we describe our current technique, with brief commentary on these modifications. Patient immobilization consists of a customized airtight vacuum bag throughout the thorax, with a wing board and U-shaped handles to place the arms above the head. Use of abdominal compression for motion management has previously been noted to produce symptomatic chest wall discomfort, so we sought an imaging-based alternative (7). The Varian Respiratory Position Management System (RPM v.1.7.5, Varian Medical System, Palo Alto, CA, USA) is used to monitor respiration-induced tumor motion. The RPM reflector block is placed on the patient’s abdomen (between the xiphoid process and umbilicus), and the position of the surrogate is monitored throughout the simulation process. Patients are instructed to breathe normally, avoiding deep breaths, sighing or talking. Video goggles were used initially to help guide patient respiration, but this process was discontinued after the system showed little to no benefit.
For the majority of patients, a 4-dimensional (4D) CT is performed on a 4-slice large bore scanner (QXi LightSpeed CT, GE Healthcare, Fairfield, CT), generating 10 phase-sorted CT image sets (0-90%, 2.5 mm slice thickness). The 0% phase image corresponds to maximum inspiration and the 50% phase image corresponds to maximum expiration. For patients that have not had an outside PET scan, a 4D PET/CT is performed on a 64-slice scanner (VCT LightSpeed, GE Healthcare) generating 5 phase-sorted PET images (10%, 30%, 50%, 70%, 90%, 3.3 mm slice thickness). As part of our 4D PET/CT protocol, a free-breathing CT scan for attenuation correction, a 3D PET (arms down) scan and 4D CT scan are also performed.
A medical physicist is present at the 4D simulation to characterize the respiration-induced tumor motion and decide on a gating strategy. The tumor position is observed in all 10 phase images, and the tumor motion is recorded in the superior-inferior, anterior-posterior and left-right directions. Based on the tumor motion and image quality, a decision is made to (I) gate with a ~50% duty cycle around expiration (“GATE3070”); (II) gate with a ~100% duty cycle throughout normal respiration (“GATE100”); or (III) treat independent of respiration (“NOGATE”). If a “GATE3070” technique is chosen (i.e., the gating window is set between the 30-70% respiratory phases), a maximum intensity projection (MIP) image and an average (AVG) image are created from the 30-70% respiratory phases as well. If a “GATE100” technique is chosen (i.e., the gating window is set for all phases of normal respiration), MIP and AVG images are created from all respiratory phases. The same images used for a “GATE100” technique can be used for a “NOGATE” technique. The decision matrix illustrated in Figure 1 is used to help determine the most beneficial gating strategy.
Image registration, contouring and treatment planning are performed in Eclipse (v.10, Varian Medical Systems). The MIP image is fused with the diagnostic PET image and the corresponding 4D PET phase images (when available) for contouring the internal target volume (ITV). The lesion is first contoured using the mediastinal window, then the lung window is examined to ensure that the gross tumor volume (GTV) is not underestimated. The ITV to planning target volume (PTV) margin is typically set as 5 mm (isotropic). However, a larger margin of 8 mm is applied in the superior-inferior direction for tumors with large motion and significant imaging artifacts. 3D-CRT and static field intensity modulated radiation therapy (IMRT) techniques have been used to create lung SBRT treatment plans, but volumetric arc therapy (VMAT) plans are the current standard of care at UCSD. Currently 1-2 arcs are used, with no entrance angles through the contralateral lung. Plans are normalized so that 100% of the ITV, and 95% of the PTV, are covered by the prescription dose. In addition, the ratios of the 100% and 50% isodose curve volumes to the PTV volume are also examined. Typical OAR include the lungs (minus the PTV), spinal cord, carina, heart, great vessels/aorta, esophagus, ribs, chest wall and skin.
Treatment verification on the linac is performed using the RPM, on-board imaging (OBI) and cone beam computed tomography (CBCT) systems (v.1.4, Varian Medical Systems). Patients are initially setup using the alignment lasers and tattoos given during the simulation. Orthogonal kilovoltage (kV) images are used to verify the alignment of the spinal structures near the target region, followed by CBCT to align the soft tissue and target location (when visible). The RPM trace guides are then set to the corresponding amplitude gating window (e.g., GATE3070 or GATE100), and a medical physicist verifies the stability of the respiratory trace prior to the start of treatment.
PET/CT in the post-SBRT setting
We have reported our findings using PET/CT scans for patients treated with SBRT for both primary NSCLC and metastatic lung tumors (19). Patients were followed by monthly clinical exam as well as imaging at 3-6 month intervals. The study involved blinding of the radiologist prior to re-review of all scans, including 86 PET/CT scans for 38 patients. Maximum standardized uptake value (SUVmax) thresholds have been proposed to guide identification of malignancy and tumor demarcation, such as SUV >2.5 (36) or >40% of the maximum SUV, but the universal validity of such thresholds is unclear (37). The mean pre-treatment SUVmax in our study was 4.95 for primary tumors, with this value decreasing by 47% on imaging 2-6 months post-treatment in patients that responded to treatment. While some reports suggest maximal response within 3 months, we advocate waiting until 6 months after SBRT for maximal SUVmax response. The mean SUVmax for metastatic lesions was 3.18, with response more variable than in primary lesions. The SUV was noted in metastatic lesions to even increase for as long as 6 months post-RT, with a decrease sometimes not seen until 10 months after treatment. Pre-SBRT SUVmax was noted to not correlate with local control or OS for either primary or metastatic lesions. In the absence of discrete SUVmax levels identified in this and other studies, we continue to use SUVmax >2.5 as a general guideline for active tumor (except for well-differentiated adenocarcinomas with are known to be poorly FDG-avid), though post-treatment comparison to pre-treatment values is most important in determining treatment respone. Generally we have adopted the strategy of waiting for two consecutive rises in SUVmax in patients with enlarging lesions on CT following SBRT to warrant treatment for recurrent disease, particularly in the setting of oligometastatic disease where apparent increases in activity are more common, unless patient shows significant clinical decline.
Conclusions
At UCSD we have applied a system of lung SBRT successfully to patients with primary NSCLC, intrapulmonary oligometastases, and MPLCs with high efficacy and low toxicity. Our technique maximizes patient comfort through absence of a body frame and fiducial markers in the tumor, instead employing a customized vacuum sealed bag for reproducible setup, and 4D-CT imaging at simulation coupled with OBI and an RPM system for motion management, based on the gating decision made at simulation. This technique is a reasonable alternative for patients that are inoperable or refuse surgery, with increasing data emerging to suggest efficacy may be equivalent to surgical resection. While patient selection is important, we found this treatment safe even in elderly patients. We are concerned that outcomes may be reduced in patients with large central lesions, and anticipate results from the ongoing RTOG 0813 trial to help determine maximum safe and effective dose for central tumors. Ultimately distant failure remains the greatest barrier to outcomes with larger, more advanced lesions. Systemic agents, particularly targeted biologic agents, appear promising and emphasize the importance of differentiating treatment based on biology to achieve better disease control. Meanwhile, we have begun to further shorten radiation treatment of very small peripheral lesions to single fraction SBRT, though will await the results of RTOG 0915 before applying single fraction routinely, particularly in >T1a lesions. Lung SBRT is also promising in the setting of oligometastatic disease, with properly selected patients living several years on modern systemic therapy, and therefore increasing the importance of durable control for intrapulmonary lesions. Follow-up should include clinical exam, CT-based imaging, and PET particularly in patients with enlarging lesions on CT. Metastatic lesions in particular can have delayed response to RT, so caution with interpreting PET within 6 months of SBRT is advised. Ultimately multidisciplinary tumor board discussion for borderline cases is recommended.
Acknowledgments
Funding: None.
Footnote
Provenance and Peer Review: This article was commissioned by the editorial office, Translational Cancer Research for the series “Recent advances in radiotherapy and targeted therapies for lung cancer”. The article has undergone external peer review.
Conflicts of Interest: All authors have completed the ICMJE uniform disclosure form (available at http://dx.doi.org/10.3978/j.issn.2218-676X.2015.08.07). The series “Recent advances in radiotherapy and targeted therapies for lung cancer” was commissioned by the editorial office without any funding or sponsorship. APS served as the unpaid Guest Editor of the series and serves as an unpaid editorial board member of Translational Cancer Research. The authors have no other conflicts of interest to declare.
Ethical Statement: The authors are accountable for all aspects of the work in ensuring that questions related to the accuracy or integrity of any part of the work are appropriately investigated and resolved.
Open Access Statement: This is an Open Access article distributed in accordance with the Creative Commons Attribution-NonCommercial-NoDerivs 4.0 International License (CC BY-NC-ND 4.0), which permits the non-commercial replication and distribution of the article with the strict proviso that no changes or edits are made and the original work is properly cited (including links to both the formal publication through the relevant DOI and the license). See: https://creativecommons.org/licenses/by-nc-nd/4.0/.
References
- Siegel RL, Miller KD, Jemal A. Cancer statistics, 2015. CA Cancer J Clin 2015;65:5-29. [PubMed]
- Morrison R, Deeley TJ, Clelan WP. The Treatment of Carcinoma of the Bronchus: A Clinical Trial to Compare Surgery and Supervoltage Radiotherapy. Lancet 1963;281:683-4.
- Bradley J, Graham MV, Winter K, et al. Toxicity and outcome results of RTOG 9311: a phase I-II dose-escalation study using three-dimensional conformal radiotherapy in patients with inoperable non-small-cell lung carcinoma. Int J Radiat Oncol Biol Phys 2005;61:318-28. [PubMed]
- Kaskowitz L, Graham MV, Emami B, et al. Radiation therapy alone for stage I non-small cell lung cancer. Int J Radiat Oncol Biol Phys 1993;27:517-23. [PubMed]
- Hayman JA, Martel MK, Ten Haken RK, et al. Dose escalation in non-small-cell lung cancer using three-dimensional conformal radiation therapy: update of a phase I trial. J Clin Oncol 2001;19:127-36. [PubMed]
- Rosenzweig KE, Fox JL, Yorke E, et al. Results of a phase I dose-escalation study using three-dimensional conformal radiotherapy in the treatment of inoperable nonsmall cell lung carcinoma. Cancer 2005;103:2118-27. [PubMed]
- Timmerman R, Papiez L, McGarry R, et al. Extracranial stereotactic radioablation: results of a phase I study in medically inoperable stage I non-small cell lung cancer. Chest 2003;124:1946-55. [PubMed]
- Timmerman R, Paulus R, Galvin J, et al. Stereotactic body radiation therapy for inoperable early stage lung cancer. JAMA 2010;303:1070-6. [PubMed]
- Baumann P, Nyman J, Hoyer M, et al. Outcome in a prospective phase II trial of medically inoperable stage I non-small-cell lung cancer patients treated with stereotactic body radiotherapy. J Clin Oncol 2009;27:3290-6. [PubMed]
- Onishi H, Shirato H, Nagata Y, et al. Hypofractionated stereotactic radiotherapy (HypoFXSRT) for stage I non-small cell lung cancer: updated results of 257 patients in a Japanese multi-institutional study. J Thorac Oncol 2007;2:S94-100. [PubMed]
- Chang JY, Senan S, Paul MA, et al. Stereotactic ablative radiotherapy versus lobectomy for operable stage I non-small-cell lung cancer: a pooled analysis of two randomised trials. Lancet Oncol 2015;16:630-7. [PubMed]
- Pastorino U, Buyse M, Friedel G, et al. Long-term results of lung metastasectomy: prognostic analyses based on 5206 cases. J Thorac Cardiovasc Surg 1997;113:37-49. [PubMed]
- Rusthoven KE, Kavanagh BD, Burri SH, et al. Multi-institutional phase I/II trial of stereotactic body radiation therapy for lung metastases. J Clin Oncol 2009;27:1579-84. [PubMed]
- Nath SK, Sandhu AP, Kim D, et al. Locoregional and distant failure following image-guided stereotactic body radiation for early-stage primary lung cancer. Radiother Oncol 2011;99:12-7. [PubMed]
- Sandhu AP, Lau SK, Rahn D, et al. Stereotactic body radiation therapy in octogenarians with stage I lung cancer. Clin Lung Cancer 2014;15:131-5. [PubMed]
- Haidar YM, Rahn DA 3rd, Nath S, et al. Comparison of outcomes following stereotactic body radiotherapy for non-small cell lung cancer in patients with and without pathological confirmation. Ther Adv Respir Dis 2014;8:3-12. [PubMed]
- Nath SK, Sandhu AP, Jensen L, et al. Frameless image-guided stereotactic body radiation therapy for lung tumors with 4-dimensional computed tomography or 4-dimensional positron emission tomography/ computed tomography. Clin Lung Cancer 2011;12:180-6. [PubMed]
- Kim D, Fuster MM, Nath SK, et al. Clinical outcomes following image-guided stereotactic body radiation for pulmonary oligometastases. J Radiosurgery & SBRT 2012;2:63-72.
- Gill BS, Rivandi AH, Sandhu SP, et al. The role of positron emission tomography following radiosurgical treatment of malignant lung lesions. Nucl Med Commun 2012;33:607-12. [PubMed]
- Rahn DA, Thakur S, Makani S, et al. Stereotactic body radiation therapy (SBRT) for multiple primary lung cancers (MPLC): a review and case series. J Radiosurgery & SBRT 2013;2:135-40.
- Rowe BP, Boffa DJ, Wilson LD, et al. Stereotactic body radiotherapy for central lung tumors. J Thorac Oncol 2012;7:1394-9. [PubMed]
- Zhang J, Yang F, Li B, et al. Which is the optimal biologically effective dose of stereotactic body radiotherapy for Stage I non-small-cell lung cancer? A meta-analysis. Int J Radiat Oncol Biol Phys 2011;81:e305-16. [PubMed]
- Videtic GM, Stephans KL, Woody NM, et al. 30 Gy or 34 Gy? Comparing 2 single-fraction SBRT dose schedules for stage I medically inoperable non-small cell lung cancer. Int J Radiat Oncol Biol Phys 2014;90:203-8. [PubMed]
- Baumann P, Nyman J, Lax I, et al. Factors important for efficacy of stereotactic body radiotherapy of medically inoperable stage I lung cancer. A retrospective analysis of patients treated in the Nordic countries. Acta Oncol 2006;45:787-95. [PubMed]
- Welter S, Theegarten D, Trarbach T, et al. Safety distance in the resection of colorectal lung metastases: a prospective evaluation of satellite tumor cells with immunohistochemistry. J Thorac Cardiovasc Surg 2011;141:1218-22. [PubMed]
- Miyakawa A, Shibamoto Y, Kosaki K, et al. Early response and local control of stage I non-small-cell lung cancer after stereotactic radiotherapy: difference by histology. Cancer Sci 2013;104:130-4. [PubMed]
- Giraud P, Antoine M, Larrouy A, et al. Evaluation of microscopic tumor extension in non-small-cell lung cancer for three-dimensional conformal radiotherapy planning. Int J Radiat Oncol Biol Phys 2000;48:1015-24. [PubMed]
- Timmerman R, McGarry R, Yiannoutsos C, et al. Excessive toxicity when treating central tumors in a phase II study of stereotactic body radiation therapy for medically inoperable early-stage lung cancer. J Clin Oncol 2006;24:4833-9. [PubMed]
- Woody NM, Stephans KL, Marwaha G, et al. Stereotactic Body Radiation Therapy for Non-Small Cell Lung Cancer Tumors Greater Than 5 cm: Safety and Efficacy. Int J Radiat Oncol Biol Phys 2015;92:325-31. [PubMed]
- Verstegen NE, Lagerwaard FJ, Hashemi SM, et al. Patterns of Disease Recurrence after SABR for Early Stage Non-Small-Cell Lung Cancer: Optimizing Follow-Up Schedules for Salvage Therapy. J Thorac Oncol 2015;10:1195-200. [PubMed]
- Yamamoto T, Jingu K, Shirata Y, et al. Outcomes after stereotactic body radiotherapy for lung tumors, with emphasis on comparison of primary lung cancer and metastatic lung tumors. BMC Cancer 2014;14:464. [PubMed]
- Norihisa Y, Nagata Y, Takayama K, et al. Stereotactic body radiotherapy for oligometastatic lung tumors. Int J Radiat Oncol Biol Phys 2008;72:398-403. [PubMed]
- Binkley MS, Trakul N, Jacobs LR, et al. Colorectal Histology Is Associated With an Increased Risk of Local Failure in Lung Metastases Treated With Stereotactic Ablative Radiation Therapy. Int J Radiat Oncol Biol Phys 2015;92:1044-52. [PubMed]
- Verhagen AF, Tavilla G, van de Wal HJ, et al. Multiple primary lung cancers. Thorac Cardiovasc Surg 1994;42:40-4. [PubMed]
- Martini N, Melamed MR. Multiple primary lung cancers. J Thorac Cardiovasc Surg 1975;70:606-12. [PubMed]
- Hong R, Halama J, Bova D, et al. Correlation of PET standard uptake value and CT window-level thresholds for target delineation in CT-based radiation treatment planning. Int J Radiat Oncol Biol Phys 2007;67:720-6. [PubMed]
- Biehl KJ, Kong FM, Dehdashti F, et al. 18F-FDG PET definition of gross tumor volume for radiotherapy of non-small cell lung cancer: is a single standardized uptake value threshold approach appropriate? J Nucl Med 2006;47:1808-12. [PubMed]