Immune-related gene ANGPT1 is an adverse biomarker for endometrial carcinoma
Introduction
The morbidity and mortality of endometrial carcinoma (EC) have been increasing in recent years. Global cancer statistical results have revealed that there were 380 thousand newly diagnosed cases and 89 thousand deaths of EC in 2018 (1).
The progress of cancer immunotherapy, especially in the field of lung cancer and melanoma, has grown rapidly in the past few years, and the relationship between the human immune system and cancer has been gradually exposed (2). Immune cells play a significant role within the tumor microenvironment (TME). In the early stage, immune cells can identify and kill most tumor cells (3-5), but some cancer cells remain alive due to their ability to functionally sculpt their microenvironment via the secretion of various cytokines, chemokines, and other factors (6). In this way, immune escape may occur, which means that tumor cells can survive by evading the attack of immune cells. This makes it more difficult to cure the cancer, especially in patients with relapse, metastasis, and advanced cancer. Therefore, new biomarkers for cancer immunotherapy might be discovered by investigating the tumor microenvironment.
A few immunotherapy drugs based on the programmed cell death protein 1/programmed death-ligand 1 (PD1/PDL1) immune checkpoint have been approved by the Food and Drug Administration (FDA) for many tumors (7-9). Nevertheless, little research and limited achievements have been made in immunotherapy for EC to date. A study of immunotherapy for advanced or recurrent EC (n=24) with PD-L1 positive expression used the treatment of pembrolizumab (anti-PD1 monoclonal antibody) and found that the objective response rate was only 13% (10). Furthermore, the majority (72%) of EC patients are grouped as lacking microsatellite instability (MSI), which was a new application marker of anti-PD1/PDL1 monoclonal antibody (11). Therefore, since the increasingly popular immunotherapy has not yet achieved much in the field of EC, newly uncovered immune-related biomarkers will play a progressively important role in improving the diagnosis and treatment of EC.
In our study, we aimed at screening immune related hub genes in EC. We investigated the co-expression genes significantly associated with EC, screening out the differentially expressed genes (DEGs). We then collected immune related genes from the ImmPort database (https://www.immport.org/), where the intersection genes of 3 parts were selected as the candidate genes. Finally, we explored the candidate genes’ protein-protein interaction (PPI) network and prognosis, and identified angiopoietin 1 (ANGPT1) as the hub immune-related gene in EC. Many studies have indicated that ANGPT forms new blood vessels in cancer, providing the tumors sufficient nutrition and oxygen (12-14). Saito et al. demonstrated that expression of ANGPT1 was higher in normal epithelium than in endometrium adenocarcinoma (15). In immunotherapy, Grenga et al. found that inhibition of the angiopoietin/TIE2 pathway neutralized the binding of Ang1 and Ang2 to TIE2, making human carcinoma cell lines in the breast, prostate, and ovary significantly more sensitive to T cell-mediated attack (16). However, the specific mechanism of ANGPT1 in EC has not been deeply investigated.
We present the following article in accordance with the REMARK reporting checklist (available at https://dx.doi.org/10.21037/tcr-21-671).
Methods
Raw data
The transcriptome profiling and clinical data of EC were downloaded from The Carcinoma Genome Atlas (TCGA) database (https://portal.gdc.carcinoma.gov/). Immune-related genes (IRGs) were collected from the ImmPort database. The gene expression profiles of GSE17025 with 91 EC samples and 12 normal samples were collected from the Gene Expression Omnibus (GEO) database (https://www.ncbi.nlm.nih.gov/geo/).
Weighted gene co-expression network analysis
The scale-free co-expression network of EC was constructed via the R language package weighted gene co-expression network analysis (WGCNA) (https://www.r-project.org/). Based on the high throughput gene expression profiles, the WGCNA algorithm could search significantly related genes modules of carcinoma via gene co-expression network analysis (17). We selected a soft threshold power of β=3, Minimum number of module genes’ size (minModuleSize) =50, and scale-free R2=0.9 to construct the standard scale-free network. The first principal component of genes expression matrix of each corresponding module were defined as the module eigengenes (MEs). The clinically significant module was identified from the correlation between MEs and clinical disease types. We used the most significant module for subsequent analyses.
Screen of DEGs
We identified the DEGs via the limma package (3.44.0 version) in R software with the thresholds of log2 Fold Change |logFC| >2.0 and false discovery rate (FDR <0.05). The heatmap and volcano plot were constructed using the ggplot2 package.
Venn analysis of DEGs, IRGs, and MEs
The VennDiagram package was used to explore the intersections genes of DEGs, IRGs, and MEs. The intersection genes were deemed the candidate genes.
Enrichment function analysis
The R packages of clusterProfiler, enrichplot, and ggplot2 were used to explore the potential enrichment functions of Gene Ontology (GO) and Kyoto Encyclopedia of Genes and Genomes (KEGG) of the intersection genes. A p-value and q-value less than 0.05 were selected as significant enrichment functions.
PPI network construction
The PPI network of intersection genes of blue module genes in the co-expression network, DEGs, and IRGs were constructed through the STRING website with a confident score >0.4 (https://string-db.org/). The nodes of the top 10 degree genes were calculated using the cytoHubba plug-in in Cytoscape software (https://cytoscape.org/).
Survival analysis
We used the R packages rms, survival, and survminer to analyze the prognosis of EC patients. Univariate cox regression analysis was visualized by using the forest map of the ggplot2 package. The survival curve was established by Kaplan-Meier method, and the difference was statistically significant (log-rank P<0.05).
Verify the protein expression of hub gene
The Human Protein Atlas (HPA) is an open-access database, which uses transcriptomics and proteomics techniques to study protein expression in different human tissues and organs from RNA and protein levels (https://www.proteinatlas.org/). All the data of HPA are free for scientists to use. We investigated the hub gene’s protein expression levels between uterine tumor and normal tissue from immunohistochemistry (IHC) analysis provided by the HPA database.
Immune infiltration analysis
The CIBERSORT deconvolution algorithm is a method for characterizing the cell composition of complex tissues from their gene expression profiles to estimate the relative abundance of 22 immune cells types (18). We used the CIBERSORT algorithm to explore the immune infiltration in 552 EC samples. Then, to achieve quality filtering, 203 tumor samples with CIBERSORT algorithm P-values of less than 0.05 were selected for further analysis using their complete clinical information.
Statistical analysis
The soft threshold power of weighted gene co-expression network analysis were set as β=3, minModuleSize =50, and scale-free R2=0.9 (WGCNA package). The DEGs were identified via limma package with the thresholds of log2 Fold Change |logFC| >2.0 and false discovery rate (FDR <0.05). Wilcoxon was used for difference analysis, Spearman was used for correlation analysis, P<0.05 was considered statistically significant. Kaplan-Meier method was used to plot the survival curve, and log-rank P<0.05 was considered statistically significant.
Ethical statement
The study was conducted in accordance with the Declaration of Helsinki (as revised in 2013).
Results
Construction of the WGCN and identification of significant MEs
The TCGA-UCEC cohort with 575 samples was included to construct the WGCNA. We identified 12 modules with the average linkage hierarchical clustering, and used a dynamic pruning method to determine the MEs. Finally, we ascertained that the blue MEs with a 0.73 score were the greatest correlation coefficients, and the genes of blue MEs were selected for the further analysis (Figure 1).
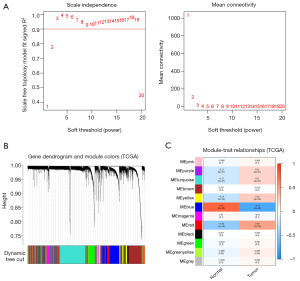
DEGs between endometrial tumor and normal tissues
The TCGA-UCEC cohort contains 552 tumor samples and 23 normal samples. A total of 1,008 down-regulated genes and 900 up-regulated genes (tumor vs. normal) were identified (Figure 2).
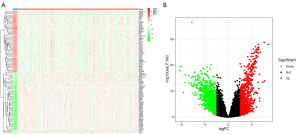
The Venn genes of blue MEs’ genes in the WGCN and DEGs and IRGs
The results showed that there were 1,908 DEGs of EC, 3,162 genes in blue MEs of the co-expression network, and 1,744 genes of IRGs (ImmPort database). Finally, 74 intersection genes were selected as the candidate genes for further analysis (Figure 3).
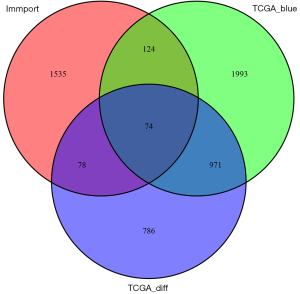
Functional enrichment analysis (GO and KEGG) of candidate genes
The findings indicated that the top 5 biological processes (BP) were calcium ion homeostasis, leukocyte migration, cellular calcium ion homeostasis, cellular divalent inorganic cation homeostasis, and epithelial cell proliferation. The top 5 cell components (CC) were the external side of plasma membrane, membrane raft, membrane microdomain, membrane region, and collagen-containing extracellular matrix. The top 5 molecular functions (MF) were the receptor ligand activity, signaling receptor activator activity, growth factor binding, G protein-coupled peptide receptor activity, and peptide receptor activity.
The top 10 pathways were the neuroactive ligand-receptor interaction, Ras signaling pathway, PI3K-Akt signaling pathway, Rap1 signaling pathway, calcium signaling pathway, MAPK signaling pathway, cytokine-cytokine receptor interaction, focal adhesion, gastric carcinoma, and cAMP signaling pathway (Figure 4).
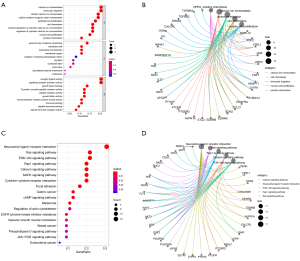
PPI network
The top 10 ranking genes of degree (connecting numbers with other nodes) were respectively C-X-C motif chemokine ligand 12 (CXCL12), secreted phosphoprotein 1 (SPP1), fibroblast growth factor 2 (FGF2), hepatocyte growth factor (HGF), ANGPT1, fibroblast growth factor 7 (FGF7), cadherin 1 (CDH1), thrombospondin 1 (THBS1), platelet derived growth factor receptor alpha (PDGFRA), and transforming growth factor beta receptor 2 (TGFBR2) (Figure 5).
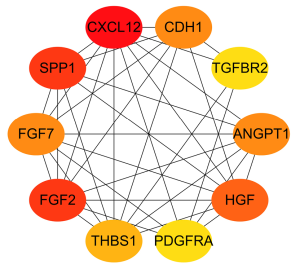
Prognosis analysis of candidate genes and identification of the hub gene of EC
Prognosis analysis results showed that there were 2 protective genes and 4 detrimental genes on EC patients’ overall survival (OS). We identified ANGPT1 as the hub immune-related gene of EC via Venn analysis of the prognosis genes and the top 10 degree PPI genes (Figure 6).
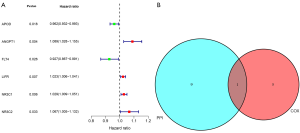
The expression and survival analysis of ANGPT1
The expression level of ANGPT1 was lower in EC than in normal tissue, and a lower expression of ANGPT1 was associated with a better OS (Figure 7).
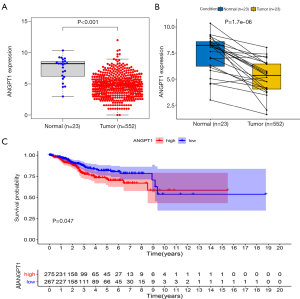
The verified results of ANGPT1 expression in EC
The IHC results of the HPA database showed that ANGPT1 had high protein expression in normal endometrial tissues (Figure 8A) and low protein expression in EC tissues (Figure 8B); the GSE17025 dataset demonstrated that ANGPT1 also had a low expression in EC (Figure 8C).
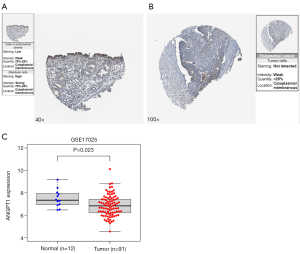
The immune infiltration analysis of EC cohort (CIBERSORT algorithm)
The CIBERSORT algorithm could calculate the relative abundance of 22 immune cells in each EC sample, and we selected 203 samples with P-values of less than 0.05 according to the CIBERSORT algorithm for further analysis. The relative abundance of 22 immune cells in 203 EC samples are shown in the histogram (Figure 9).
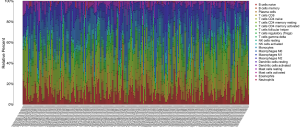
Furthermore, we explored the correlation between ANGPT1 and immune cells, and the results showed that the expression of ANGPT1 was negatively correlated with regulatory T cells (Tregs) (Figure 10A) and activated natural killer (NK) cells (Figure 10B), while it was positively correlated with the resting memory cluster of differentiation 4 (CD4) T cells (Figure 10C), activated memory CD4 T cells (Figure 10D), and activated dendritic cells (DCs) (Figure 10E).
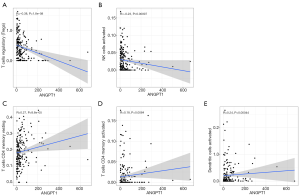
The Kaplan-Meier (grouping by the median of abundance) survival analysis results of 22 immune cells indicated that high infiltration of Tregs (P=0.005) and CD8 T cells (0.066) improved OS in EC patients (Figure 11).
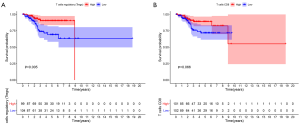
Discussion
In the present study, we identified ANGPT1 as hub immune-related gene in EC. Firstly, we identified the co-expression genes module associated with EC through WGCNA based on TCGA-UCEC cohort. Secondly, we screened the intersection genes from module genes, IRGs, and DEGs for prognostic analysis and PPI network. Finally, we investigated the correlation between ANGPT1 and immune cells. Our results indicated that ANGPT1 was down-regulated in EC patients, while decreased expression of ANGPT1 was associated with a better outcome in EC patients. Furthermore, Tregs were high-infiltrating in EC, and ANGPT1 was negatively correlated with Tregs.
The gene ANGPT1 encodes a secreted glycoprotein that belongs to the angiopoietin family (19), it binds to the endothelial cell membrane receptor tyrosine kinase w (also known as TEK) to contribute to tumor angiogenesis (20,21). In the present study, ANGPT1 was shown to be low-expressed in EC tissues. Saito et al. also found that the expression of ANGPT1 was lower in endometrial adenocarcinoma than in normal epithelium (15), which was consistent with our findings. Our results also demonstrated that ANGPT1 was associated with poor prognosis, and the overexpression of ANGPT1 resulted in reduced OS in EC patients, which might be associated with tumor microenvironment immune cells. Recent studies have shown that some anti-angiogenic therapies have immunomodulatory effects in both peripheral and tumor microenvironments (16,22). Antiangiogenic drugs tyrosine kinase inhibitors (TKIs) can target vascular endothelial growth factor (VEGF) and its receptors to inhibition of the angiopoietin/TIE2 pathway to delay the growth and development of tumors (23). Finke et al. and Grenga et al. found that the TKIs sunitinib and sorafenib altered the immune landscape and reduced the number or function of immunosuppressive cells (16,24). Furthermore, an anti-angiogenic TKIs combined vaccine can enhance the anti-tumor activity, which may be due to the activation of lymphocytes and myelocytes by the tumor microenvironment, normalization of blood vessels, decrease of tumor cell density, and improvement of vascular perfusion and oxygenation (25). A phase II trial which aimed to investigate the efficacy of trebananib (a TKI inhibitor) in recurrent/persistent EC indicated that the objective response rate (ORR) was 43.7% (26). Despite the limited efficacy, this showed the potential of TKI inhibitors in the treatment of EC. Since ANGPT1 is the 1 of the regulators of angiogenesis for EC, it is a potential therapeutic target for EC patients.
Enrichment function analysis of ANGPT1 showed that it is an essential part for leukocyte migration, epithelial cell proliferation, membrane regulation, growth factor binding, Ras signaling pathway, PI3K-Akt signaling pathway, Rap1 signaling pathway, MAPK signaling pathway, cytokine-cytokine receptor interaction, and so on. The enrichment functions results indicated that ANGPT1 participates in in various cancer processes. Activated leukocyte cell adhesion molecule is an adverse biomarker for endometrioid EC via promoting cancer cell migration, invasion, and metastasis (27). This indicates that leukocyte cells have a significant role in progression for endometrioid EC. Leukocyte cells have also been shown to include numbers of immune cells, which also indicated that the immune cells infiltration into TME may have a deep influence on EC patients. Regrettably, the association between ANGPT1 and immune cell infiltrations of EC remains unclear. The ANGPT1 is also an important regulator of epithelial cell proliferation; it is well known that EC is an epithelial cancer, and cell proliferation and growth are critical processes for tumor development. Cancer cells with a higher ability of proliferation and growth may indicate poorer outcomes. Several studies have investigated the inhibition of epithelial EC cell proliferation and growth via microRNA-29c or silencing the gene expression (28,29). Further, ANGPT1 also has an essential role in several signaling pathways in EC. Numerous studies have supported that the Ras signaling pathway, PI3K-Akt signaling pathway, Rap1 signaling pathway, and MAPK signaling pathway were important pathways in tumorigenesis, drug resistance, and progression of EC (30-34). In addition to several cancer pathways, ANGPT1 is also a significant regulator of cytokine-cytokine receptor interaction, and the PPI network showed that ANGPT1 interacted with CXCL12, FGF2, HGF, PDGFRA, and so on; most of them were the cytokine or cytokine receptor, as well as the regulator for the immune system (35-39). It has been shown that CXCL12 and FGF2 are adverse markers for UCEC via promotion of the proliferation, migration, and invasion of cancer cells (40,41). The interaction of ANGPT1 and other genes showed that ANGPT1 may be a significant regulator of the TME for EC.
In exploring the expression levels of ANGPT1 and its prognostic role for EC patients, we found that ANGPT1 was down-regulated in EC, and the lower expression of ANGPT1 was associated with longer lifespan in TCGA database analysis. This was validated via the expression levels of ANGPT1 in the GSE17025 dataset. Nevertheless, ANGPT1 is not only low-expressed in EC, but also in epithelial ovarian cancer and bladder cancer (42,43). However, the role of ANGPT1 in EC remains unclear. Flores-Pérez et al. showed that ANGPT1 and TGFBR2 were positive regulators of angiogenesis in breast cancer (44), indicating that ANGPT1 acted as an adverse marker for breast cancer patients. Despite of its role in breast cancer, ANGPT1 seemed to act as a tumor suppressor in lung cancer (45).
To date, with the better understanding of the TME in cancer, there is no doubt that TME plays a pivotal role in tumor occurrence, progression, drug resistance, and recurrence for EC (46-48). Since the enrichment functions and PPI network showed that ANGPT1 has a significant role or interaction in immune cell, cytokine, and cytokine receptor, we also investigated the relationship between ANGPT1 and the immune cell infiltration for EC. The results showed that ANGPT1 was negatively correlated with Tregs and activated NK cells. Witkiewicz et al. showed that there were high levels of NK cells but decreased Tregs in complex atypical endometrial hyperplasia and well-differentiated carcinoma treated with progestins (49). Further, a study by Degos et al. indicated that the TME can reshape NK cell phenotype and function to promote tumor progression (50); this finding showed us that NK cells can be changed in TME and promote the tumor progression, which contrasted the prior theory that NK cells only act protectively for cancer patients. The association between immune cell infiltration and its effect on the prognosis of EC patients showed that high infiltration of Tregs and CD8 T cells lengthens the OS of EC patients. Meanwhile, ANGPT1 was positively correlated with the resting memory of CD4 T cells, activated memory CD4 T cells, and activated DCs. Zhang et al. supported that the higher the expression of tumor-infiltrating immune cell (TIC) of CD4+ cells, the better outcome of EC patients (51). There have been DCs detected in the TME of EC as well, and the S100- and HLA-DR-positive DCs may act as tumor suppressors (52). From these findings, ANGPT1 is positively correlated with protection from immune cells infiltrate, but negatively correlated with the promoting tumor progression TICs in the TME of EC; thus ANGPT1 is a favorable IRG for EC patients.
Conclusions
It was shown that ANGPT1 was downregulated in EC patients, and its low expression indicated a better prognosis of EC patients. The enrichment functions of ANGPT1 showed that it is an essential regulator for immune response for EC. Analysis of the association between ANGPT1 and TICs showed that it has a positive correlation with protect immune cells infiltrate such as CD4 T cells, activated memory CD4 T cells, and activated DCs, but is negatively correlated with the promoting tumor progression TICs such as such as Tregs and activated NK cells in the TME of EC, thus ANGPT1 is an adverse IRG for EC patients.
Acknowledgments
Funding: None.
Footnote
Reporting Checklist: The authors have completed the REMARK reporting checklist. Available at https://dx.doi.org/10.21037/tcr-21-671
Conflicts of Interest: All authors have completed the ICMJE uniform disclosure form (available at https://dx.doi.org/10.21037/tcr-21-671). The authors have no conflicts of interest to declare.
Ethical Statement: The authors are accountable for all aspects of the work in ensuring that questions related to the accuracy or integrity of any part of the work are appropriately investigated and resolved. The study was conducted in accordance with the Declaration of Helsinki (as revised in 2013).
Open Access Statement: This is an Open Access article distributed in accordance with the Creative Commons Attribution-NonCommercial-NoDerivs 4.0 International License (CC BY-NC-ND 4.0), which permits the non-commercial replication and distribution of the article with the strict proviso that no changes or edits are made and the original work is properly cited (including links to both the formal publication through the relevant DOI and the license). See: https://creativecommons.org/licenses/by-nc-nd/4.0/.
References
- Bray F, Ferlay J, Soerjomataram I, et al. Global cancer statistics 2018: GLOBOCAN estimates of incidence and mortality worldwide for 36 cancers in 185 countries. CA Cancer J Clin 2018;68:394-424. [Crossref] [PubMed]
- Hegde PS, Chen DS. Top 10 Challenges in Cancer Immunotherapy. Immunity 2020;52:17-35. [Crossref] [PubMed]
- Lei X, Lei Y, Li JK, et al. Immune cells within the tumor microenvironment: Biological functions and roles in cancer immunotherapy. Cancer Lett 2020;470:126-33. [Crossref] [PubMed]
- Tanaka A, Sakaguchi S. Regulatory T cells in cancer immunotherapy. Cell Res 2017;27:109-18. [Crossref] [PubMed]
- Duhen T, Duhen R, Montler R, et al. Co-expression of CD39 and CD103 identifies tumor-reactive CD8 T cells in human solid tumors. Nat Commun 2018;9:2724. [Crossref] [PubMed]
- Hinshaw DC, Shevde LA. The Tumor Microenvironment Innately Modulates Cancer Progression. Cancer Res 2019;79:4557-66. [Crossref] [PubMed]
- Luo J, Nishikawa G, Prasad V. A systematic review of head-to-head trials of approved monoclonal antibodies used in cancer: an overview of the clinical trials agenda. J Cancer Res Clin Oncol 2019;145:2303-11. [Crossref] [PubMed]
- Raschi E, Mazzarella A, Antonazzo IC, et al. Toxicities with Immune Checkpoint Inhibitors: Emerging Priorities From Disproportionality Analysis of the FDA Adverse Event Reporting System. Target Oncol 2019;14:205-21. [Crossref] [PubMed]
- Galanina N, Bejar R, Choi M, et al. Comprehensive Genomic Profiling Reveals Diverse but Actionable Molecular Portfolios across Hematologic Malignancies: Implications for Next Generation Clinical Trials. Cancers (Basel) 2018;11:11. [Crossref] [PubMed]
- Ott PA, Bang YJ, Berton-Rigaud D, et al. Safety and Antitumor Activity of Pembrolizumab in Advanced Programmed Death Ligand 1-Positive Endometrial Cancer: Results From the KEYNOTE-028 Study. J Clin Oncol 2017;35:2535-41. [Crossref] [PubMed]
- Brooks RA, Fleming GF, Lastra RR, et al. Current recommendations and recent progress in endometrial cancer. CA Cancer J Clin 2019;69:258-79. [Crossref] [PubMed]
- Cai E, Yang D, Zhang Y, et al. Angiopoietin-1 is associated with a decreased risk of lymph node metastasis in early stage cervical cancer. Histol Histopathol 2020;35:1029-34. [PubMed]
- Fagiani E, Christofori G. Angiopoietins in angiogenesis. Cancer Lett 2013;328:18-26. [Crossref] [PubMed]
- Gillen J, Richardson D, Moore K. Angiopoietin-1 and Angiopoietin-2 Inhibitors: Clinical Development. Curr Oncol Rep 2019;21:22. [Crossref] [PubMed]
- Saito M, Sato Y, Watanabe J, et al. Angiogenic factors in normal endometrium and endometrial adenocarcinoma. Pathol Int 2007;57:140-7. [Crossref] [PubMed]
- Grenga I, Kwilas AR, Donahue RN, et al. Inhibition of the angiopoietin/Tie2 axis induces immunogenic modulation, which sensitizes human tumor cells to immune attack. J Immunother Cancer 2015;3:52. [Crossref] [PubMed]
- Langfelder P, Horvath S. WGCNA: an R package for weighted correlation network analysis. BMC Bioinformatics 2008;9:559. [Crossref] [PubMed]
- Newman AM, Liu CL, Green MR, et al. Robust enumeration of cell subsets from tissue expression profiles. Nat Methods 2015;12:453-7. [Crossref] [PubMed]
- Suri C, Jones PF, Patan S, et al. Requisite role of angiopoietin-1, a ligand for the TIE2 receptor, during embryonic angiogenesis. Cell 1996;87:1171-80. [Crossref] [PubMed]
- Bridges EM, Harris AL. The angiogenic process as a therapeutic target in cancer. Biochem Pharmacol 2011;81:1183-91. [Crossref] [PubMed]
- Jayson GC, Kerbel R, Ellis LM, et al. Antiangiogenic therapy in oncology: current status and future directions. Lancet 2016;388:518-29. [Crossref] [PubMed]
- Farsaci B, Higgins JP, Hodge JW. Consequence of dose scheduling of sunitinib on host immune response elements and vaccine combination therapy. Int J Cancer 2012;130:1948-59. [Crossref] [PubMed]
- Zhu XD, Tang ZY, Sun HC. Targeting angiogenesis for liver cancer: Past, present, and future. Genes Dis 2020;7:328-35. [Crossref] [PubMed]
- Finke JH, Rayman PA, Ko JS, et al. Modification of the tumor microenvironment as a novel target of renal cell carcinoma therapeutics. Cancer J 2013;19:353-64. [Crossref] [PubMed]
- Farsaci B, Donahue RN, Coplin MA, et al. Immune consequences of decreasing tumor vasculature with antiangiogenic tyrosine kinase inhibitors in combination with therapeutic vaccines. Cancer Immunol Res 2014;2:1090-102. [Crossref] [PubMed]
- Moore KN, Sill MW, Tenney ME, et al. A phase II trial of trebananib (AMG 386; IND#111071), a selective angiopoietin 1/2 neutralizing peptibody, in patients with persistent/recurrent carcinoma of the endometrium: An NRG/Gynecologic Oncology Group trial. Gynecol Oncol 2015;138:513-8. [Crossref] [PubMed]
- Fan L, Xu H, Yang R, et al. Combination of Capsaicin and Capsiate Induces Browning in 3T3-L1 White Adipocytes via Activation of the Peroxisome Proliferator-Activated Receptor gamma/beta3-Adrenergic Receptor Signaling Pathways. J Agric Food Chem 2019;67:6232-40. [Crossref] [PubMed]
- Shimoyama H, Shibata TK, Ito M, et al. Partial silencing of fucosyltransferase 8 gene expression inhibits proliferation of Ishikawa cells, a cell line of endometrial cancer. Biochem Biophys Rep 2020;22:100740. [Crossref] [PubMed]
- Van Sinderen M, Griffiths M, Menkhorst E, et al. Restoration of microRNA-29c in type I endometrioid cancer reduced endometrial cancer cell growth. Oncol Lett 2019;18:2684-93. [Crossref] [PubMed]
- Bosse T, ter Haar NT, Seeber LM, et al. Loss of ARID1A expression and its relationship with PI3K-Akt pathway alterations, TP53 and microsatellite instability in endometrial cancer. Mod Pathol 2013;26:1525-35. [Crossref] [PubMed]
- Kyle RA. Is there a correct time to begin treatment of multiple myeloma? Haematologica 1987;72:107-10. [PubMed]
- Schrauwen S, Depreeuw J, Coenegrachts L, et al. Dual blockade of PI3K/AKT/mTOR (NVP-BEZ235) and Ras/Raf/MEK (AZD6244) pathways synergistically inhibit growth of primary endometrioid endometrial carcinoma cultures, whereas NVP-BEZ235 reduces tumor growth in the corresponding xenograft models. Gynecol Oncol 2015;138:165-73. [Crossref] [PubMed]
- Weigelt B, Warne PH, Lambros MB, et al. PI3K pathway dependencies in endometrioid endometrial cancer cell lines. Clin Cancer Res 2013;19:3533-44. [Crossref] [PubMed]
- Zhang G, Cheng Y, Zhang Q, et al. ATXLPA axis facilitates estrogeninduced endometrial cancer cell proliferation via MAPK/ERK signaling pathway. Mol Med Rep 2018;17:4245-52. [PubMed]
- Katoh M. FGFR inhibitors: Effects on cancer cells, tumor microenvironment and whole-body homeostasis Int J Mol Med 2016;38:3-15. (Review). [Crossref] [PubMed]
- Manoguerra AS. Full thickness skin burns secondary to an unusual exposure to diquat dibromide. J Toxicol Clin Toxicol 1990;28:107-10. [Crossref] [PubMed]
- Peng S, Wang R, Zhang X, et al. EGFR-TKI resistance promotes immune escape in lung cancer via increased PD-L1 expression. Mol Cancer 2019;18:165. [Crossref] [PubMed]
- Vitiello GA, Bowler TG, Liu M, et al. Differential immune profiles distinguish the mutational subtypes of gastrointestinal stromal tumor. J Clin Invest 2019;129:1863-77. [Crossref] [PubMed]
- Zhang SZ, Wang QQ, Yang QQ, et al. NG2 glia regulate brain innate immunity via TGF-beta2/TGFBR2 axis. BMC Med 2019;17:204. [Crossref] [PubMed]
- Chen P, Xing T, Wang Q, et al. MicroRNA-202 inhibits cell migration and invasion through targeting FGF2 and inactivating Wnt/beta-catenin signaling in endometrial carcinoma. Biosci Rep 2019;39:BSR20190680. [Crossref] [PubMed]
- Liu P, Long P, Huang Y, et al. CXCL12/CXCR4 axis induces proliferation and invasion in human endometrial cancer. Am J Transl Res 2016;8:1719-29. [PubMed]
- Hata K, Nakayama K, Fujiwaki R, et al. Expression of the angopoietin-1, angopoietin-2, Tie2, and vascular endothelial growth factor gene in epithelial ovarian cancer. Gynecol Oncol 2004;93:215-22. [Crossref] [PubMed]
- Szarvas T, Jager T, Totsch M, et al. Angiogenic switch of angiopietins-Tie2 system and its prognostic value in bladder cancer. Clin Cancer Res 2008;14:8253-62. [Crossref] [PubMed]
- Flores-Pérez A, Marchat LA, Rodriguez-Cuevas S, et al. Dual targeting of ANGPT1 and TGFBR2 genes by miR-204 controls angiogenesis in breast cancer. Sci Rep 2016;6:34504. [Crossref] [PubMed]
- Yao S, Dong SS, Ding JM, et al. Sex-specific SNP-SNP interaction analyses within topologically associated domains reveals ANGPT1 as a novel tumor suppressor gene for lung cancer. Genes Chromosomes Cancer 2019; Epub ahead of print. [Crossref] [PubMed]
- Gu S, Ni T, Wang J, et al. CD47 Blockade Inhibits Tumor Progression through Promoting Phagocytosis of Tumor Cells by M2 Polarized Macrophages in Endometrial Cancer. J Immunol Res 2018;2018:6156757. [Crossref] [PubMed]
- Li BL, Wan XP. Prognostic significance of immune landscape in tumour microenvironment of endometrial cancer. J Cell Mol Med 2020;24:7767-77. [Crossref] [PubMed]
- Liu J, Nie S, Wu Z, et al. Exploration of a novel prognostic risk signatures and immune checkpoint molecules in endometrial carcinoma microenvironment. Genomics 2020;112:3117-34. [Crossref] [PubMed]
- Witkiewicz AK, McConnell T, Potoczek M, et al. Increased natural killer cells and decreased regulatory T cells are seen in complex atypical endometrial hyperplasia and well-differentiated carcinoma treated with progestins. Hum Pathol 2010;41:26-32. [Crossref] [PubMed]
- Degos C, Heinemann M, Barrou J, et al. Endometrial Tumor Microenvironment Alters Human NK Cell Recruitment, and Resident NK Cell Phenotype and Function. Front Immunol 2019;10:877. [Crossref] [PubMed]
- Zhang S, Minaguchi T, Xu C, et al. PD-L1 and CD4 are independent prognostic factors for overall survival in endometrial carcinomas. BMC Cancer 2020;20:127. [Crossref] [PubMed]
- Lijun Z, Xin Z, Danhua S, et al. Tumor-infiltrating dendritic cells may be used as clinicopathologic prognostic factors in endometrial carcinoma. Int J Gynecol Cancer 2012;22:836-41. [Crossref] [PubMed]