Can proton beam therapy be clinically relevant for the management of lung cancer?
Introduction
Lung cancer is the leading cause of cancer death in the United States and worldwide (1). According to the American Cancer Society, there were 221,200 new cases and an estimated 158,040 deaths in the USA in 2015. Mortality for lung cancer is higher than prostate, breast, colorectal, and pancreatic cancers combined. Local control (LC) is important for lung cancer. Failure to control local disease in the thorax accounts for 2/3 of deaths for lung cancer.
In terms of treatment, lung cancer has been divided into two major histologic types: non-small cell lung cancer (NSCLC) and small cell lung cancer (SCLC). According to SEER data, SCLC accounts for 10-15% of all lung cancer, and they are typically treated by systemic therapy. The rest are NSCLC, which have an overall prognosis of 15% 5-year survival. Lung cancer survival significantly decreases with later staging (SEER). Early stages IA and IB have 49% and 45% 5-year survival, respectively. Stage IIA and IIB have about 30% 5-year survival. However, there is sharp decline in survival from stages IIIA (14%) to IIIB (5%) to IV (1%). Treatment guidelines have been developed by the National Cancer Care Network (NCCN) depending on the stage of the disease at presentation.
Local disease is the major cause of symptoms in lung cancer patients. Radiation therapy (RT) plays a role in almost all stages of lung cancer. For stage I NSCLC, surgery alone is recommended for medically operable tumors. Alternatively, RT is used as a sole modality to treat stage I NSCLC for patients who are medically inoperable or refuse surgery. In stage II-III NSCLC, radiation is used in combination with systemic therapy (chemotherapy and/or targeted therapy) and sometimes surgery to provide definitive treatment. Radiation is also used in limited stage SCLC in combination with systemic therapy for curative treatment. RT is the most effective treatment for palliation for stage IV lung cancer (2).
Treatment of lung cancer is challenging due to: (I) most patients are diagnosed at late stages III or IV; (II) most lung patients are elderly (2/3 patients are 65 years old or older) and often have co-morbidities; (III) current treatments for lung cancer are difficult to tolerate since they require radical surgery and/or combined systemic cytotoxic drugs and radiation to the above organs; (IV) lung cancers are radiation-resistant and surrounded by healthy lung tissues, which are radiation sensitive. Furthermore, lung tumors are often located near other critical organs such as the heart, esophagus, and spinal cord. Too much radiation to the lung can cause radiation pneumonitis, which can decrease lung function, require long term steroid and oxygen, and sometimes cause death. The risk of radiation pneumonitis is dependent on both irradiated volume and dose. Radiation esophagitis can cause pain and difficulty with swallowing, hence causing weight loss. Sometimes, it can cause ulceration and stenosis as late side effects. Too much radiation to the heart can lead to major cardiac adverse events such as myocardial infarction, pericarditis, etc. Similar to the lung, the risk of complication to the heart and esophagus is both dose and volume dependent.
Thus, any improvements to lung cancer radiation treatment would ideally increase dose to the tumor and/or decrease dose to surrounding healthy tissues. Because of the Bragg peak effect, particle beam treatment has unique physical properties that allow RT to be delivered with less normal tissue exposure compared with photon-based RT (3); hence, lung cancer is a perfect application for particle beam therapy for these reasons. In this article, the role of proton beam therapy (PBT) in lung cancer is explored in three scenarios: (I) early stage lung cancer; (II) locally advanced lung cancer; (III) recurrent lung cancer.
Physics and radiobiology of proton beam therapy
Starting in the mid 1950’s, over 130,000 patients have undergone particle beam treatments, of which, about 110,000 are proton and the rest are light ions such as carbon and helium. Traditionally, the therapeutic use of charged particles is motivated primarily by their inverted depth-dose profile from the Bragg peak; thus, the collateral damage induced in healthy tissues surrounding the tumor is limited. Distinct beams of different energies can then be integrated to achieve the prescribed dose in a region as large as the target volume, resulting in the production of what is designated as the spread-out Bragg peak (SOBP) (4) (Figure 1). A proton is produced by removing the orbiting electron from the hydrogen atom, resulting in a positively charged hydrogen ion. These ions are further accelerated to a typical energy of 70-250 mega electron-volts (MeV) in an accelerator such as synchrotron, or cyclotron. These protons are then delivered to a patient precisely to the appropriate depth in the body through either a “passive scattering” or “beam scanning” technique (5). Protons can be delivered with two different radiation techniques: passive scattering proton therapy (PSPT) or pencil beam scanning technique (PBS). In PSPT, the tumor volume is irradiated as a whole, using collimators and compensators for dose conformality. With PBS, the target volume is scanned spot-by-spot with a narrow proton beam, sequential targeting of 300 to 600 spots in a voxel-like array, enabling intensity-modulated proton therapy (IMPT) (6,7).
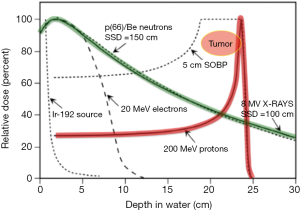
Charged particles such as protons have minimal ionization along their beam path, the dose delivered to any point along the path is minimal, and the entrance dose for an individual beam is less than that for a comparable photon beam (8). Consequently, deep-seated tumors, especially those close to organs at risk (OAR), represent an ideal configuration for exploiting the advantageous physical characteristics of charged particle beams (5). Typically, the integral dose delivered to the body can be 2-3 times lower with protons when compared to X-rays delivered by intensity modulated radiation therapy (IMRT) (9). The increased linear energy transfer (LET) compared to X-rays when protons are near the Bragg peak is correlated with a localized energy deposition and induction of enhanced unrepairable biological damage (10). Protons are also different from photon irradiation in terms of killing power. The relative biological effectiveness (RBE) for proton RT is estimated at 1.1 times that for photons. Hence, proton RT has theoretically 10% more cancer kill capability for each gray (Gy) than photon RT (11). As historically described in the literature, this absorbed energy leads to breaks in DNA strands and free radical formation, resulting in cell death predominantly in rapidly reproducing tissue. Tumor response, as we now know, is strongly associated with local microenvironment, communication, and interaction between cancer cells and healthy cells, as well as among targeted and non-targeted cells (12).
A current aspect of discussion is whether the use of a fixed relative biological effectiveness in PBT is still appropriate, or if a variable RBE is more pertinent taking into account the dependency on LET, tissue properties, total dose, and dose fractionation (5). The main benefits of PBT when compared to photon are based on the above mentioned interactions with matter when the particles are traveling in a patient. The fact that the proton beam virtually stops at the target volume results in marginal radiation exposure beyond the tumor, allowing for the sparing of distally placed tissues (13). In contrast, photons travel through the entire body from the entrance to the exit point. This difference in dosage to distal OAR makes proton therapy a more ideal treatment option for tumors surrounded by critical tissues. Maximal sparing of these crucial organs is important in potentially improving patient outcomes (survival, quality of life, and toxicity) (11).
From a genuinely physics point of view, the dose distribution of protons is in most cases superior to that of photons. The lateral dose fall-off or beam penumbra is better than photon at shallow depth, then it becomes worse for protons as the proton beam travels deep into the tissue (14). Several studies demonstrate that PBT allows the delivery of higher tumor doses compared to photons while sparing healthy tissues. The Radiation Oncology Collaborative Comparison consortium performed a planning study for 25 NSCLC, stage IA-IIIB patients. On 4D F18-labeled fluorodeoxyglucose (FDG) positron emission tomography (PET)—computed tomography (CT) scans, the gross tumor, clinical and planning target volumes, and OAR were delineated and it was illustrated that passive scatter proton therapy resulted in the lowest dose to the OARs, while keeping the dose to the target at 70 Gy. The integral dose was higher for 3D-Conformal RT (CRT) (59%) and IMRT (43%) than for PSPT. The mean lung dose was 18.9 Gy for 3D-CRT, 16.4 Gy for IMRT, and 13.5 Gy for PSPT (15). Chang et al. completed a planning study comparing 3D-CRT, IMRT, and PSPT in 25 patients with stage I-III NSCLC. Using photons, the mean tumor dose of 63 Gy and the mean V20 (lung) was 34.8%, compared to a mean proton dose of 74 cobalt gray equivalent (CGE) to the tumor and a mean V20 (lung) of 31.6%. The authors concluded that proton treatment appears to reduce dose to normal tissues significantly, even with dose escalation, compared with standard-dose photon therapy, either 3D-CRT or IMRT (16). In stage I NSCLC, protons also achieved lower doses to OARs than photon stereotactic body radiation therapy (SBRT) (17).
It is essential to recognize that range uncertainties, or uncertainty regarding where the proximal and distal edges of the Bragg peak will be in the patient, are a perilous component to proton therapy planning (18,19). In hypo-dense tissues, where beam attenuation is low (e.g., lung), this consideration carries a major importance, especially at the most distal part of the SOBP. Because of organ motion as well as changes in lung density during respiration, PBT in the lung requires significantly more effort in planning and dose validation (2).
Proton therapy for early stage lung cancer
SBRT surface has been found to be as effective as surgery in regards to primary tumor control rates and overall survival (OS) for patients who refuse surgery or with medically inoperable early-stage lung cancer (20-22).
Since 1999 studies have reported an 80 to 90 percent rate of LC for patients with stage I NSCLC treated with hypofractionated proton beam radiotherapy (23-27). Supporting the idea that a higher dose can result in better tumor control, dose escalation has been actively studied in the past two decades. These clinical investigations confirmed a dose-response relationship both in terms of LC and OS duration (28).
Bush et al. reported phase I-II study from Loma Linda University for a group of 111 patients with NSCLC stage T1-2 N0 using a 2-week course of hypofractionated proton therapy for 60-70 Gy in 10 treatments with tolerable side effect and good LC (about 75% for T2 patients at 4 years), no grade 3 pneumonitis, or change in FEV1 or DLCO function. There were four patients with rib fractures (tumor abutting the chest wall).
The range uncertainties were studied and compared the use of protons and photons for SBRT for NSCLC by Seco et al. (29). The authors attempted an overview of the advantages and disadvantages of the usage of this different particles reproduced in Table 1.
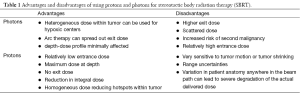
Full table
This concept is demonstrated in Figure 2, which shows the comparative dosimetry with photon plan on the left and proton plan on the right. The photon plan has higher dose conformity at the high dose region near the tumor due to range uncertainty while the proton plan can spare much better at the low dose region. An example of an actual patient is shown in Figure 3.
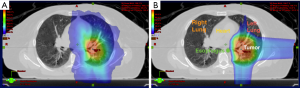
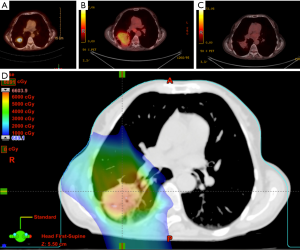
Some authors explored dose escalation with SBRT using proton. Onishi et al. found that among different SBRT regimens, a biologically effective dose (BED) of at least 100 Gy is associated with a higher 5-year LC rate (91.9% vs. 73.6%) and longer OS (88.4% vs. 69.4%) than a BED of less than 100 Gy (30). Bush et al. reported treatment dose escalated from 51 to 70 CGE in 10 fractions for 111 patients (T1/T2) (4). LC and survival were well-established to improve with escalated doses. OS at 5 years was 18%, 32%, and 51% at 51 CGE, 60 CGE, and 70 CGE. Nonetheless, tumors greater than 5 cm were associated with worse LC. There were no cases of radiation pneumonitis, suggesting room for increased dose delivery to improve outcomes (3). Nihei et al., reported treatment dose between 70-94 CGE in 20 fractions for 37 patients (T1/T2 <5 cm) (25). Two-year LC and survival were 98% and 84%, respectively. Late pulmonary toxicity was associated with tumor shrinkage during treatment, demonstrating the need for adaptive replanning (31). Recently, Makita et al. (24) explored toxicities and prognostic factors related to two high-dose PBT protocols (BED ≥100 Gy) for treatment of stage I NSCLC. Patients (n=32) with peripherally located tumors were given 66 Gy (RBE) over 10 fractions while patients (n=24) with centrally located tumors were given 80 Gy (RBE) over 25 fractions. The three-year OS, progression-free survival (PFS), LC rates were 81.3%, 73.4%, and 96.0%, respectively.
The authors concluded that both high-dose PBT protocols achieved high LC rates with tolerable toxicities and that the standardized uptake value was a significant prognostic factor.
At Scripps Proton Therapy Center, patient is set-up in supine position with both arms above the head and immobilized with a vacuum bag and head holder Figure 4. 4D CT scan was done using Varian RPM system to evaluate tumor motion. If the tumor motion is 1 cm or less, then the ITV approach was done. The GTV was contoured on lung window on inspiration and expiration phases, GTV was expanded with 5 mm margin for CTV (excluding chest wall or bronchus). The two CTV are combined to form ITV. Setup uncertainty (5 mm) and range uncertainty are added to form beam specific PTV as shown in Figure 4. Typically, two fields are used to treat the tumor daily, and orthogonal kV images were obtained for each field prior to the treatment.
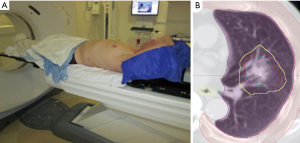
For patients with tumor more than 1 cm, then we use the deep inspiration breath hold (DIBH) technique with the SDX Spirometric Motion Management System (QFix, Avondale, PA, USA) as shown in Figure 5. The system consists of a spirometric breathing device, goggle, and a computer system. The system allows patients to voluntarily hold their breath at a set level with the visual feedback.
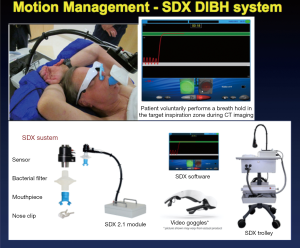
Figure 6 showed a case of early stage lung cancer that was treated using the SDX system to mitigate the tumor motion. Intensity modulation proton therapy (IMPT) was used to treat this early stage non-small cell lung cancer (NSCLC) with 70 Gy in 10 fractions. Two posterior fields were used. Tumor motion was minimized using the SDX system. The panels below showed the PET/CT images before (A) and 8 months after treatment (B).
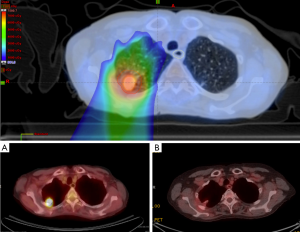
Proton therapy for locally advanced lung cancer
Stage II/III NSCLC are treated with combination of chemotherapy and RT. Median survival for stage II/III NSCLC has improved from 12 months in the mid 1980 (RTOG 7301) to 29 months currently (RTOG 0617), as shown in Figure 7.
RTOG 9410 clinical trial demonstrated better median survival (17 months over 15 months) and lower local relapse rate (41% vs. 50%) of concurrent chemo-radiation over sequential chemotherapy followed RT of 60 Gy. This gain is offset by higher rate of grade 3 or higher GI toxicity (23% vs. 4%). The grade 3 or higher lung toxicity is about 13-14% for these groups of patients. Elective nodal radiation was done in this study, and 3-D conformal photon radiation was used. RTOG 0617 compared dose escalation of 74 vs. 60 Gy, both are given concurrently with systemic therapy. The patients in the lower dose arm has better median survival of 28.7 vs. 20.3 months (36). This study does not use elective nodal irradiation and radiation was given by either 3-D conformal or IMRT technique with photon. Subsequent analysis of this study speculated that the lower survival in patients of higher dose group due to the cardiac toxicity, radiation pneumonitis and esophagitis, and possible geographic miss. There is a current prospective randomized study comparing proton versus photon IMRT (concurrent with platinum doublet chemotherapy) for stage II to IIIB NSCLC at MD Anderson and MGH. The maximum dose of 74 Gy in 2 Gy per fraction is prescribed if dose tolerance is achievable, if not, then dose is reduced by 2 Gy step until dose constraints to all OAR are met. This RTOG 1308 protocol has enrolled about 300 patients of total 560 patients at the time of this writeup, and the median heart dose is lower in the proton arm.
Chang et al. reported a phase II trial in which 44 patients were treated with proton therapy for a dose of 74 Cobalt Gray Equivalent (CGE) in 37 fractions with concurrent chemotherapy (carboplatin and paclitaxel) (37). In this study, the median OS was 29.4 months, PFS is 63% and OS is 86% at 1 year. Local failure was 9%, and no patient experienced grade 4 or 5 proton-related adverse events.
Sejpal et al. (38) compared the outcomes of PBT + chemotherapy (n=62), 3D-CRT + chemotherapy (n=74), and IMRT + chemotherapy (n=66) in patients with stage III NSCLC. The median total radiation dose was 63 Gy and 74 CGE respectively the photon and proton groups. The incidence of severe pneumonitis and esophagitis was lower in the proton group even with higher radiation dose. The median survival times were 17.7 months for the 3-D CRT group, 17.6 months for the IMRT group, and 24.4 months for the proton therapy group (P=0.1). It can be predicted that higher doses of PBT could be delivered to lung tumors with lower rates of esophagitis and pneumonitis, despite current concern regarding the apparent lack of benefit and potentially harmful of dose escalation in locally advanced (stage III) NSCLC (36).
Grutters et al. (39) performed a meta-analysis of observational studies comparing photon, proton, and carbon-ion radiotherapy in the treatment of NSCLC, in which they concluded that proton beam radiotherapy did not offer a statistically significant improvement in OS when compared to photon-based stereotactic RT. However, both modalities were significantly better than conventional photon RT. Particle therapy may be more beneficial in stage III NSCLC, especially in reducing adverse events, and may spare lung volumes from receiving low-dose irradiation from exiting photon beams (39). Thus, proton therapy may offer an advantage by safely delivering higher doses within a short overall treatment time (hypofractionation) (40).
Majority of current proton therapy in the USA is done using passive scatter proton therapy. There are few centers exploring the use of pencil beam scanning. There are several modes of pencil beam scanning such as uniform scanning, spot scanning, raster scanning. A detailed descriptions is beyond the scope of this article. Pencil beam scanning allows the use of intensity modulated proton therapy (IMPT). IMPT is more subjective to organ motion; however, IMPT allows for better dose conformality and organ sparing. IMPT also allows simultaneous boost within the target volume. An example of dose distribution is shown in Figure 8.
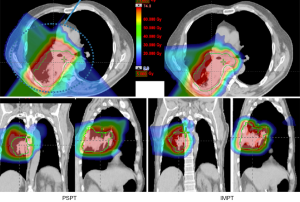
Proton therapy for recurrent lung cancer
Patients, who have previous received RT and now with local recurrence, present a challenge to current treatment. They are often not a surgical candidate and systemic chemotherapy does not work well in this patient population. Re-irradiation with SBRT or proton therapy offers a new curative treatment modality with reasonable side effect profile. SBRT works well in patients with a small recurrence with lots of motions and not in central location (2 cm away from the main bronchus and trachea). SBRT is given with 5 or fewer fractions and chemotherapy is not given concurrently. For patient with larger tumor and/or nodal recurrence in area of less motion, proton therapy is a better option. Re-irradiation with proton therapy can be given as hypofractionated or standard fractionation regimen and can be given concurrently with systemic chemotherapy. The larger and the more complex shape of the tumor, the better proton therapy is. The majority of these patients need sparing of the remaining healthy lung (even at low dose), heart, and esophagus. This is where proton excels. There are several phase I-II trials ongoing investigating role of proton therapy for this group of patients. Figures 9,10 shows cases of re-treatment with IMPT for two patients with local recurrent NSCLC after previous radiation treatment.
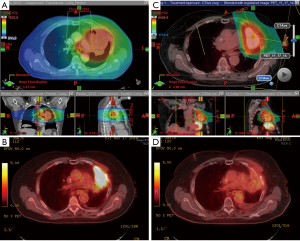
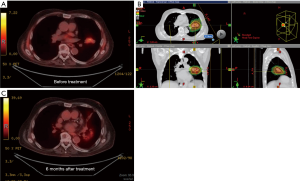
Current clinical trials
At the time of this writing, there are 17 clinical trials that are investigating the use of proton therapy for lung cancer. Of the trials, 13 are open to patient accrual and 4 are closed. There is one pilot study, 13 phase I and/or II studies, one phase III study, and two medical physics studies. Ten of the trials are single institution, while the other seven are multi-institutional. Six of the trials involve combined modalities (i.e., concurrent chemotherapy). For more information about these clinical trials, please refer to the PTCOG website (www.PTCOG.ch) or clinicaltrials.gov. Areas under investigation with current clinical trials are:
- Prospective randomized trial for stage II/III between photon and proton: RTOG-1308 is a phase 3 randomized study comparing proton versus IMRT, allowing the dose range of 60-74 Gy, similar to the RTOG 0617; however, with proton therapy, it is hoped that the reduction in critical structure dose (heart and lung) will improve survival. PCORI trial is being proposed by Dr. Brad Hoppe at University of Florida to compare proton versus photon for stage II/III;
- Hypofractionation regimen for stage II/III. This is done to reduce the treatment cost of proton therapy and extending the experience from proton therapy for early stage lung cancer. Dr. Brad Hoppe (University of Florida, USA) started a protocol with total of 60 Gy given 15, 17, 20, and 24 fraction regimens given concurrently with chemotherapy. Dr. Cliff Robinson (Washington University, USA) proposed 15 fraction regimen for total dose of 45, 48.75, 52.5, 56.25, and 60 Gy concurrently with chemotherapy;
- SBRT boost to the primary tumor after treating the mediastinal nodes with proton therapy. Dr. Kristin Higgins (Emory University, USA) proposed 44 Gy given in 22 fractionations, followed by boost to primary tumor with SBRT with following regimens, 18 Gy in 2 fractions, 20 Gy in 2 fractions, 30 Gy in 5 fractions, 30 Gy in 5 fractions, 35 Gy in 5 fractions and 40 Gy in 5 fractions;
- Proton therapy for post-op treatment for patients with N2 disease. Drs. Cliff Robinson (Washington University, USA) and Charles Simone (University of Pennsylvania) proposed a randomized trial of photon versus proton for post-op radiation of patients with stage IIIA (pN2) for dose of 50.4 to 54 Gy;
- Re-irradiation protocols for patients who received previous radiation to the lung or nearby area. There are several institutions investigating this area.
Conclusions
The unique properties of proton therapy allow the sparing of normal structures around the target tumors, which could be beneficial for lung cancer patients with tumors near critical structures, limited pulmonary reserve, or with larger tumors (3). Proton therapy can lower the risk of treatment side effects and provide a valuable tool for dose escalation or re-irradiation. Implementation of proton therapy is currently hindered by the cost of the technology and limited approval from healthcare payers. Per treatment fraction, proton therapy is more expensive than standard photon therapy. However, if one factors into the cost of treatment excess side effects and sequential mortality and also the new hypofractionation schemes, proton therapy can be established to be cost-effective for the management of selected cases of lung cancer (41). The current argumentation tends to overlook the existing evidence on clinical- and cost-effectiveness data. Patients deserve the best technology available, on the medical and ethical basis of the best available evidence. Therefore, when analyzing new and emerging technologies, all risks of treatment-related side effects and complications, as well as all possible clinical improvements, need to be taken under consideration (42,43).
On another front, with more vendors available, there are more competitions to have the equipments less expensive, more reliable with more features. Technology and its implementation continue to improve on a daily basis. Pencil beam scanning including IMPT may produce better clinical results than scattered PBT (11). More research is needed to successfully optimize proton delivery treatments. Improvements are needed to optimize the motion management, volumetric image guidance, and adaptive therapy. More research is needed to create plans that are more robust in the face of uncertainty, especially for the new pencil beam scanning systems. Additional prospective trials for lung cancer are needed to clarify the category of patients that will benefit the most from proton therapy. Findings of increased quality of life, LC, and survival will hopefully lead to greater cost-effectiveness in the future.
Acknowledgments
Funding: None.
Footnote
Provenance and Peer Review: This article was commissioned by the Guest Editors (Lyudmila Bazhenova and Ajay Pal Singh Sandhu) for the series “Recent advances in radiotherapy and targeted therapies for lung cancer” published in Translational Cancer Research. The article has undergone external peer review.
Conflicts of Interest: All authors have completed the ICMJE uniform disclosure form (available at http://dx.doi.org/10.3978/j.issn.2218-676X.2015.08.15). The series “Recent advances in radiotherapy and targeted therapies for lung cancer” was commissioned by the editorial office without any funding or sponsorship. HG serves as an unpaid Co-Editor-in-Chief of Translational Cancer Research. The authors have no other conflicts of interest to declare.
Ethical Statement: The authors are accountable for all aspects of the work in ensuring that questions related to the accuracy or integrity of any part of the work are appropriately investigated and resolved.
Open Access Statement: This is an Open Access article distributed in accordance with the Creative Commons Attribution-NonCommercial-NoDerivs 4.0 International License (CC BY-NC-ND 4.0), which permits the non-commercial replication and distribution of the article with the strict proviso that no changes or edits are made and the original work is properly cited (including links to both the formal publication through the relevant DOI and the license). See: https://creativecommons.org/licenses/by-nc-nd/4.0/.
References
- Siegel R, Ma J, Zou Z, et al. Cancer statistics, 2014. CA Cancer J Clin 2014;64:9-29. [PubMed]
- Allen AM, Pawlicki T, Dong L, et al. An evidence based review of proton beam therapy: the report of ASTRO's emerging technology committee. Radiother Oncol 2012;103:8-11. [PubMed]
- Bush DA, Cheek G, Zaheer S, et al. High-dose hypofractionated proton beam radiation therapy is safe and effective for central and peripheral early-stage non-small cell lung cancer: results of a 12-year experience at Loma Linda University Medical Center. Int J Radiat Oncol Biol Phys 2013;86:964-8. [PubMed]
- Tommasino F, Durante M. Proton radiobiology. Cancers (Basel) 2015;7:353-81. [PubMed]
- Grant JD, Chang JY. Proton-based stereotactic ablative radiotherapy in early-stage non-small-cell lung cancer. Biomed Res Int 2014;2014:389048.
- Wink KC, Roelofs E, Solberg T, et al. Particle therapy for non-small cell lung tumors: where do we stand? A systematic review of the literature. Front Oncol 2014;4:292. [PubMed]
- McGowan SE, Burnet NG, Lomax AJ. Treatment planning optimisation in proton therapy. Br J Radiol 2013;86:20120288 [PubMed]
- Skinner HD, Komaki R. Proton radiotherapy in the treatment of lung cancer. Transl Cancer Res 2012;1:264-70.
- Lomax AJ, Bortfeld T, Goitein G, et al. A treatment planning inter-comparison of proton and intensity modulated photon radiotherapy. Radiother Oncol 1999;51:257-71. [PubMed]
- Goodhead DT. Energy deposition stochastics and track structure: what about the target? Radiat Prot Dosimetry 2006;122:3-15. [PubMed]
- Schild SE, Rule WG, Ashman JB, et al. Proton beam therapy for locally advanced lung cancer: A review. World J Clin Oncol 2014;5:568-75. [PubMed]
- Prise KM, O'Sullivan JM. Radiation-induced bystander signalling in cancer therapy. Nat Rev Cancer 2009;9:351-60. [PubMed]
- Halperin EC, Perez CA, Brady LW, et al. Perez and Brady's Principles and Practice of Radiation Oncology. Philadelphia: Lippincott Williams & Wilkins, 2007.
- Engelsman M, Schwarz M, Dong L. Physics controversies in proton therapy. Semin Radiat Oncol 2013;23:88-96. [PubMed]
- Roelofs E, Engelsman M, Rasch C, et al. Results of a multicentric in silico clinical trial (ROCOCO): comparing radiotherapy with photons and protons for non-small cell lung cancer. J Thorac Oncol 2012;7:165-76. [PubMed]
- Chang JY, Zhang X, Wang X, et al. Significant reduction of normal tissue dose by proton radiotherapy compared with three-dimensional conformal or intensity-modulated radiation therapy in Stage I or Stage III non-small-cell lung cancer. Int J Radiat Oncol Biol Phys 2006;65:1087-96. [PubMed]
- Bongers EM, Dahele MR, Slotman BJ, et al. Proton stereotactic body radiation therapy for clinically challenging cases of centrally and superiorly located stage I non-small-cell lung cancer: in regards to Register et al. Int J Radiat Oncol Biol Phys 2011;80:1015-1022. Int J Radiat Oncol Biol Phys 2012;82:492-author reply 492. [PubMed]
- Urie M, Goitein M, Wagner M. Compensating for heterogeneities in proton radiation therapy. Phys Med Biol 1984;29:553-66. [PubMed]
- Schaffner B, Pedroni E. The precision of proton range calculations in proton radiotherapy treatment planning: experimental verification of the relation between CT-HU and proton stopping power. Phys Med Biol 1998;43:1579-92. [PubMed]
- Chang JY, Balter PA, Dong L, et al. Stereotactic body radiation therapy in centrally and superiorly located stage I or isolated recurrent non-small-cell lung cancer. Int J Radiat Oncol Biol Phys 2008;72:967-71. [PubMed]
- Timmerman R, Paulus R, Galvin J, et al. Stereotactic body radiation therapy for inoperable early stage lung cancer. JAMA 2010;303:1070-6. [PubMed]
- Shirvani SM, Jiang J, Chang JY, et al. Comparative effectiveness of 5 treatment strategies for early-stage non-small cell lung cancer in the elderly. Int J Radiat Oncol Biol Phys 2012;84:1060-70. [PubMed]
- Hata M, Tokuuye K, Kagei K, et al. Hypofractionated high-dose proton beam therapy for stage I non-small-cell lung cancer: preliminary results of a phase I/II clinical study. Int J Radiat Oncol Biol Phys 2007;68:786-93. [PubMed]
- Makita C, Nakamura T, Takada A, et al. High-dose proton beam therapy for stage I non-small cell lung cancer: Clinical outcomes and prognostic factors. Acta Oncol 2015;54:307-14. [PubMed]
- Nihei K, Ogino T, Ishikura S, et al. High-dose proton beam therapy for Stage I non-small-cell lung cancer. Int J Radiat Oncol Biol Phys 2006;65:107-11. [PubMed]
- Bush DA, Slater JD, Bonnet R, et al. Proton-beam radiotherapy for early-stage lung cancer. Chest 1999;116:1313-9. [PubMed]
- Bush DA, Slater JD, Shin BB, et al. Hypofractionated proton beam radiotherapy for stage I lung cancer. Chest 2004;126:1198-203. [PubMed]
- Bradley J, Graham MV, Winter K, et al. Toxicity and outcome results of RTOG 9311: a phase I-II dose-escalation study using three-dimensional conformal radiotherapy in patients with inoperable non-small-cell lung carcinoma. Int J Radiat Oncol Biol Phys 2005;61:318-28. [PubMed]
- Seco J, Panahandeh HR, Westover K, et al. Treatment of non-small cell lung cancer patients with proton beam-based stereotactic body radiotherapy: dosimetric comparison with photon plans highlights importance of range uncertainty. Int J Radiat Oncol Biol Phys 2012;83:354-61. [PubMed]
- Onishi H, Shirato H, Nagata Y, et al. Hypofractionated stereotactic radiotherapy (HypoFXSRT) for stage I non-small cell lung cancer: updated results of 257 patients in a Japanese multi-institutional study. J Thorac Oncol 2007;2:S94-100. [PubMed]
- Chang JY, Komaki R, Wen HY, et al. Toxicity and patterns of failure of adaptive/ablative proton therapy for early-stage, medically inoperable non-small cell lung cancer. Int J Radiat Oncol Biol Phys 2011;80:1350-7. [PubMed]
- Kong FM, Ten Haken RK, Schipper MJ, et al. High-dose radiation improved local tumor control and overall survival in patients with inoperable/unresectable non-small-cell lung cancer: long-term results of a radiation dose escalation study. Int J Radiat Oncol Biol Phys 2005;63:324-33. [PubMed]
- Rengan R, Rosenzweig KE, Venkatraman E, et al. Improved local control with higher doses of radiation in large-volume stage III non-small-cell lung cancer. Int J Radiat Oncol Biol Phys 2004;60:741-7. [PubMed]
- Machtay M, Bae K, Movsas B, et al. Higher biologically effective dose of radiotherapy is associated with improved outcomes for locally advanced non-small cell lung carcinoma treated with chemoradiation: an analysis of the Radiation Therapy Oncology Group. Int J Radiat Oncol Biol Phys 2012;82:425-34. [PubMed]
- Socinski MA, Blackstock AW, Bogart JA, et al. Randomized phase II trial of induction chemotherapy followed by concurrent chemotherapy and dose-escalated thoracic conformal radiotherapy (74 Gy) in stage III non-small-cell lung cancer: CALGB 30105. J Clin Oncol 2008;26:2457-63. [PubMed]
- Bradley JD, Paulus R, Komaki R, et al. Standard-dose versus high-dose conformal radiotherapy with concurrent and consolidation carboplatin plus paclitaxel with or without cetuximab for patients with stage IIIA or IIIB non-small-cell lung cancer (RTOG 0617): a randomised, two-by-two factorial phase 3 study. Lancet Oncol 2015;16:187-99. [PubMed]
- Chang JY, Komaki R, Lu C, et al. Phase 2 study of high-dose proton therapy with concurrent chemotherapy for unresectable stage III nonsmall cell lung cancer. Cancer 2011;117:4707-13. [PubMed]
- Sejpal S, Komaki R, Tsao A, et al. Early findings on toxicity of proton beam therapy with concurrent chemotherapy for nonsmall cell lung cancer. Cancer 2011;117:3004-13. [PubMed]
- Grutters JP, Kessels AG, Pijls-Johannesma M, et al. Comparison of the effectiveness of radiotherapy with photons, protons and carbon-ions for non-small cell lung cancer: a meta-analysis. Radiother Oncol 2010;95:32-40. [PubMed]
- De Ruysscher D, Chang JY. Clinical controversies: proton therapy for thoracic tumors. Semin Radiat Oncol 2013;23:115-9. [PubMed]
- Grutters JP, Pijls-Johannesma M, Ruysscher DD, et al. The cost-effectiveness of particle therapy in non-small cell lung cancer: exploring decision uncertainty and areas for future research. Cancer Treat Rev 2010;36:468-76. [PubMed]
- Holm S, Takala T. High hopes and automatic escalators: a critique of some new arguments in bioethics. J Med Ethics 2007;33:1-4. [PubMed]
- Grutters JP, Abrams KR, de Ruysscher D, et al. When to wait for more evidence? Real options analysis in proton therapy. Oncologist 2011;16:1752-61. [PubMed]