Grape seed proanthocyanidin extract induces apoptosis of HL-60/ADR cells via the Bax/Bcl-2 caspase-3/9 signaling pathway
Introduction
Acute myeloid leukemia (AML) is a hematopoietic malignancy (1,2). It is the commonest type of acute leukemia in adults with an incidence of 50 patients per 1 million and a median diagnostic age of 68 y. This condition is very rare in pediatric patients, with an incidence of about 7 per 1 million children annually (1,3,4). With the approval of novel drugs in recent years, the frontline management of AML is rapidly changing. If tolerated, however, intensive chemotherapy remains the general therapeutic choice for patients suffering newly-diagnosed AML (5). A combination of cytarabine and an anthracycline (such as daunorubicin or idarubicin) has been the therapeutic standard of AML for several decades (1). Resistance to cytotoxic chemotherapy leads to AML relapse, resulting in a very low long-term survival rate ranging from 10–15% among patients aged 60 years and older (6). In recent years, many naturally-derived products such as parthenolide, triptolide and resveratrol have been found efficacious in the targeting of multiple leukemic stem cell pathways, resulting in AML remission (7). As such, increasing attention is being paid to the role of natural products in AML treatment.
Grape seed proanthocyanidin extract (GSPE), a compound extracted from grape seeds, is composed of flavane-3-alcohol monomers, dimers, trimers, and tetramers, as well as oligomers of proanthocyanidins, catechin, epicatechin gallate and epigallocatechin (8,9). Due to its significant health-promoting effects and potential clinical value, GSPE has attracted considerable interest in the recent year. The compound has been reported to promote skeletal muscle fiber transformation resulting in improvement of fatigue (10), enhance L-cell differentiation in intestinal organoids thus promoting hormone production in the treatment of type-2 diabetes and obesity (11-13), reverse multidrug resistance to cytotoxic agents in the setting of AML (14), inhibit secretion of complement component 3 thereby relieving renal interstitial inflammation and fibrosis (15) and exert neuroprotective effects in neonatal hypoxic-ischemic brain injury (16). A randomized, double-blind, placebo-controlled clinical study documented the benefit of GSPE on maintaining vascular endothelial function and normal blood pressure in participants with prehypertension (17). Furthermore, GSPE has also been reported to exert anti-cancer effects in malignancies such as esophageal squamous carcinoma (18), hepatocellular carcinoma (19), breast cancer (20), colon cancer (21) and AML (22). We previously documented the potential application of GSPE in combination with cytotoxic drugs for the treatment of AML (14). GSPE reverses drug resistance to both cytarabine and doxorubicin [also as known as adriamycin (ADR)] in HL-60/ADR cells via inhibition of the PI3K/Akt signaling pathway, resulting in the downregulated expression of multidrug resistance protein 1 (MDR1), multidrug resistance-associated protein 1 (MRP1) and lung resistance-related protein (LRP) (14). In addition, we previously found that GSPE directly mediates apoptosis in HL-60/ADR cells (14). Here, we aimed to elucidate the mechanism via which GSPE induces apoptosis in HL-60/ADR cells. We present the following article in accordance with the MDAR checklist (available at https://dx.doi.org/10.21037/tcr-21-920).
Methods
Cell lines and reagents
ADR-resistant human AML HL-60/ADR and parental HL-60 cells were purchased from the Institute of Hematology, Chinese Academy of Medical Sciences (Tianjin, China). Both HL-60 and HL-60/ADR cells were cultured in Roswell Park Memorial Institute (RPMI 1640; Gibco, Grand Island, USA) medium supplemented with 10% fetal bovine serum (FBS; purchased from Hangzhou Sijiqing Bioengineering Material Co. Ltd., Hangzhou, China) and maintained in a humidified incubator at 37 °C and 5% CO2. Cells were routinely passaged every other day. The HL-60/ADR cells were maintained in medium containing 1,000 ng/mL ADR (Shenzhen Main Luck Pharmaceuticals Co. Ltd., Shenzhen, China) to maintain an MDR phenotype and incubated in ADR-free medium for 2 weeks prior to experimentation.
GSPE with a purity exceeding 95% was purchased from Tianjin Jianfeng Natural Product R&D Co. Ltd. (Tianjin, China; Manufacturer's content Report) while 3-(4,5-dimethylthiazol-2-yl)-2,5-diphenyltetrazolium bromide (MTT) was purchased from Sigma (St Louis, USA). Trizol reagents were purchased from Invitrogen (Carlsbad, USA). The Annexin V-Fluorescein Isothiocyanate/Propidium Iodide (FITC/PI) Apoptotic Cell Detection Kit was purchased from BD Biotech (Franklin Lakes, USA). The PrimeScript™ RT Reagent Kit with gDNA Eraser and TB Green® Premix EX Taq™ (Tli RnaseH Plus) were purchased from Takara Biotechnology Inc (Dalian, China). Primer sequence design and other reagents were purchased from Sangon Biotech Inc (Shanghai, China). The Caspase-3/9 Activity Detection Kit was purchased from Beyotime Biotechnology Inc (Shanghai, China).
Cell viability assay
Cells were plated in 96-well plates at 5×104 cells/well (100 µL of cell suspension with a concentration of 5×105 cells/mL) and treated with various doses (6.25, 12.5, 25.0, 50.0, 75.0, 100.0, 125.0, 150.0 and 200.0 µg/mL) of GSPE for 24, 48 and 72 h. The total liquid volume was 150 µL. Then, 50 µL of MTT solution (2 mg/mL) was added to each well at 37 °C for another 4 h. Plates were centrifuged (Eppendorf, Hamburg, Germany) for 10 min at 2,500 rpm at room temperature; the supernatant fraction was subsequently discarded. In order to dissolve formazan, DMSO was added at 100 µL/well and mixed thoroughly. Absorbance (OD value) was read at 570 nm using a microplate reader (Bio-Rad Laboratories, Hercules, USA). All experiments were repeated 3 times. The cell viability rate was calculated according to the following formula: cell viability (%) = OD570, GSPE/OD570, control × 100%.
Trypan blue staining for viable cell counts
Cells were seeded in 24-well plates at 1.5×105 cells per well (500 µL of cell suspension with a concentration of 3×105 cells/mL) and treated with different concentrations (0, 25, 50, 75, 100 µg/mL) of GSPE for 24 h. Cell counts and viability were measured using Trypan blue staining and automated cell counting (Countstar® BioMarine, Shanghai Ruiyu Biotechnology Co. Ltd., Shanghai, China). Three duplicate wells were set up for each group studied and cells from each well were counted 3 times.
Apoptosis analysis by flow cytometry
Treated cells were harvested and resuspended in PBS solution at a concentration of 1×106 cells/mL; 100 µL of cells were then placed into Eppendorf tubes and mixed with 400 µL binding buffer. After Annexin V-FITC staining for 15 min and PI staining for 5 min at room temperature in the dark, samples were analyzed using a BD FACS Verse Flow cytometer (BD Biosciences, San Jose, USA) and Flow Jo Software (Tristar, Washington DC, USA). Cells in the Q2 phase (Annexin V-FITC +/PI −) were considered to be in the late stage of apoptosis, while cells in the Q4 phase (Annexin V-FITC +/PI +) were considered to be in the early stage of apoptosis. All experiments were performed independently in triplicate.
Real-time PCR analysis for the quantification of Bcl-2 and Bax mRNA expression
Cells were seeded in 6-well plates at 1.0×106 cells/well (2 mL of cell suspension with a concentration of 1×106 cells/mL) and treated with different concentrations (0, 25, 50, 75, 100 µg/mL) of GSPE for 24 h. Total RNA of all treated cells, including attached cells as well as cells in the supernatant, was extracted using Trizol reagent and reverse transcribed into cDNA using the RT Reagent Kit. Real-time PCR was performed using a Biosystems 7500 Fast Real-Time PCR (Bio-Rad, Laboratories, Hercules, USA) according to the manufacturer’s protocol. Primer sequences are shown in Table 1. Reaction conditions were as follows: 1 cycle of 20 s at 95 °C followed by 40 cycles of 3 s at 95 °C and 15 s at 60 °C. β-actin was used as an internal control. The relative expression of genes investigated was determined by the 2-ΔΔCT method.
Table 1
mRNA | Primer (5’→ 3’) |
---|---|
Bcl-2 (Forward) | AGACCGAAGTCCGCAGAACC |
Bcl-2 (Reverse) | GAGACCACACTGCCCTGTTG |
Bax (Forward) | GCGACTGATGTCCCTGTCTC |
Bax (Reverse) | GGCCTCAGCCCATCTTCTTC |
Caspase-3/9 activity analysis
Caspase-3 and caspase-9 activity was evaluated using the Caspase-3/9 Activity Assay Kit according to the manufacturer’s protocol. Briefly, treated cells were extracted and incubated in lysis buffer on ice for 15 min. The mixture was subsequently centrifuged at 16,000 g for 15 min at 4 °C. Supernatant was collected and incubated with Ac-DEVD-ρNA and Ac-LEHD-ρNA for 2 h. Absorbance was measured at 405 nm using a microplate reader. Caspase-3/9 activity of each sample was quantified according to the standard curve and normalized by protein concentration.
Statistical analysis
All experiments were performed three times. Data are presented as means ± SD; figures were drawn using Microsoft Excel 2019. Comparisons were performed via one-way ANOVA in GraphPad Prism 7. P<0.05 was considered statically significant.
Results
GSPE inhibits proliferation of HL-60/ADR cells
Our findings indicated that HL-60/ADR cells were resistant to cytarabine, ADR, vincristine, daunorubicin, mitoxantrone, pirarubicin, homoharringtonine and etoposide (14). Here, we re-evaluated the drug resistance of HL-60/ADR cells to ADR (Figure 1A). The IC50 of ADR in HL-60 and HL-60/ADR cells was 2.033 and 415.7 µM, respectively, and 204.5 times higher in HL-60/ADR cells than in HL-60 cells, confirming that the HL-60/ADR cells studied here were indeed drug resistant.
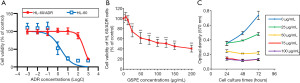
To determine whether GSPE directly inhibits HL-60/ADR cellular proliferation and which concentrations are most efficacious, HL-60/ADR cells were cultured with 6.25, 12.5, 25.0, 50.0, 75.0, 100.0, 125.0, 150.0 and 200.0 µg/mL concentrations of GSPE for 24, 48 and 72 h. As shown in Figure 1B, proliferation of HL-60/ADR cells was significantly inhibited after cell culturing with GSPE concentrations above 25.0 µg/mL for 24 h as compared to control samples cultured without GSPE (P<0.001). To verify whether the inhibitory effect of GSPE was irreversible, HL-60/ADR cells were cultured for 72 h with GSPE concentrations of 25, 50, 75 and 100 µg/mL (controls were cultured without GPSE). The optical density (OD) value exhibited a sustainable increase at 48 and 72 h when the concentration of GSPE was 25 µg/mL, indicating that GSPE does not completely inhibit HL-60/ADR cellular proliferation at this concentration (Figure 1C). When GSPE concentrations were 50, 75 and 100 µg/mL, the OD value (570 nm) of HL-60/ADR cells did not increase at 48 and 72 h as compared to the OD value at 24 h, which indicating that HL-60/ADR cellular proliferation was completely inhibited at GSPE concentrations above 50 µg/mL (Figure 1C).
GSPE induces apoptosis of HL-60/ADR cells
To confirm how GSPE inhibits proliferation of HL-60/ADR cells, we first examined changes in cellular morphology. After 24 h of culturing, cells were stained with trypan blue. As GSPE concentration at which cells were cultured increased, the proportion of apoptotic cells seen on microscopy increased, while cell survival decreased (Figure 2A-2E). The survival rate of cells cultured with GSPE concentrations above 50 µg/mL significantly decreased when compared with controls (P<0.001) (Figure 2F). Thus, morphological observations underscored that GSPE induces HL-60/ADR cell apoptosis.
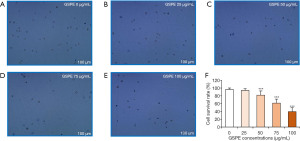
In order to further verify the apoptotic effects of GSPE in HL-60/ADR cells, cells were cultured with GSPE concentrations of 25, 50, 75 and 100 µg/mL for 24 h prior to analysis using flow cytometry. As shown in Figure 3, flow cytometry data revealed an increase in apoptotic cells associated with increasing GSPE concentrations. Indeed, GSPE concentrations above 25 µg/mL exhibited a stronger apoptotic effect in HL-60/ADR cells when compared to controls; the proportion of apoptotic cells increased in a dose-dependent manner. These findings underscore that GSPE directly induces apoptosis in HL-60/ADR cells.
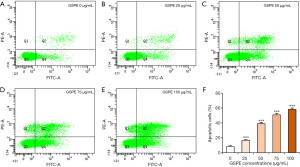
GSPE induces apoptosis via the alteration of Bax and Bcl-2 mRNA expression in HL-60/ADR cells
To further explore how GSPE induces apoptosis via the classical pathway, expression of Bcl-2 and Bax mRNA in HL-60/ADR cells was studied using real-time PCR. As the concentrations of GSPE increased, so did levels of Bax mRNA expression (Figure 4A). GSPE concentrations above 75 µg/mL were found to be associated with significantly increased Bax mRNA expression (P<0.001). However, Bcl-2 mRNA expression decreased as the concentrations of GSPE increased (Figure 4B). When GSPE concentrations were greater than 25 µg/mL, expression of Bcl-2 mRNA was significantly decreased (P<0.01). The Bax mRNA / Bcl-2 mRNA value was found to be significantly increased when GSPE concentrations were greater than 50 µg/mL (P<0.01; Figure 4C).
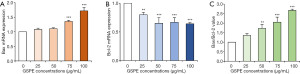
GSPE increases activity of caspases-3 and -9
Activity of caspases-3 and -9 were detected at 6, 12 and 24 h after culturing HL-60/ADR cells with varying GSPE concentrations. GSPE concentrations greater than 75 µg/mL were associated with significantly increased caspase-3 activity in HL-60/ADR cells cultured for 6 h (P<0.001); GSPE concentrations greater than 50 µg/mL were associated with significantly increased caspase-3 activity after 12 h (50 µg/mL, P<0.05; 75 µg/mL, P<0.01; 100 µg/mL, P<0.001; Figure 5A). After 24 h, GSPE concentrations greater than 50 µg/mL were found to be associated with significantly increased caspase-3 activity (P<0.001; Figure 5A). However, only a concentration of 100 µg/mL was found to significantly increase caspase-9 activity (Figure 5B). Thus, our findings confirmed that GSPE-induced apoptosis of HL-60/ADR cells occurs via the Bax/Bcl-2 caspase 3/9 pathway.
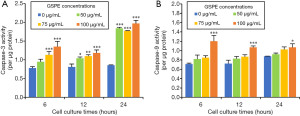
Discussion
Resistance to cytotoxic chemotherapy remains a challenge in the treatment of cancers such as AML (1). GSPE, a flavonoid polyphenolic compound extracted from grape seeds, has been reported to exhibit antitumor activity in the setting of malignancies such as bladder, breast and lung cancers (23,24). GSPE is composed of both monomeric and polymeric +catechin, -epicatechin gallate and -epigallocatechin linked together via either C4-C6 or C4-C8 bonds (13,25). According to the structural classification of proanthocyanidins, their commonest constitutive units can be divided into A-type and B-type procyanidins; GSPE is a B-type procyanidin (26). Our previous study reported that GSPE reverses the resistance of HL-60/ADR cells to cytosine, arabinoside and ADR via the inhibition of the PI3K/Akt signaling pathway (14). Interestingly, we found that GSPE directly induces apoptosis in HL-60/ADR cells even without the addition of chemotherapeutic drugs (14). This study was carried out to elucidate how GSPE induces apoptosis in HL-60/ADR cells.
There are 3 major apoptosis-associated pathways resulting in caspase activation: the mitochondrial/apoptosome pathway, the death receptor pathway, and the CTL/NK-derived granzyme B-dependent pathway (27). Caspase activation culminates during cell apoptosis and the inflammatory response (27). Mitochondrial apoptosome-driven caspase activation is a key caspase-activating mechanism initiated by cytotoxic drugs and results in caspase-2, -3, -6, -7, -8, -9, and -10 activation during apoptosis (27). Caspases-3 and -9, however, are the most important during mitochondrial apoptosome-driven caspase activation. The intrinsic mitochondrial pathway of apoptosis is tightly controlled by Bcl-2 family proteins, including Bcl-2, Bcl-XL and MCL-1 (28). While Bcl-2 is an anti-apoptotic protein, Bax is a pro-apoptotic effector protein. Inhibition of Bcl-2 was previously reported to promote apoptosis in leukemic cells (29).
GSPE has been reported to exhibit anticancer activity in the setting of several malignancies including AML (14,22), hepatocellular carcinoma (19), bladder cancer (23), pancreatic cancer (30) and colon cancer (21). Gao et al. previously reported that GSPE induces apoptosis in Jurkat cells via the c-Jun NH2-terminal (JNK) pathway by sustained JNK activation and Cip1/p21 up-regulation that finally culminates in caspase activation (31). Hu et al. previously reported that GSPE induces dose-dependent, mitochondria-associated apoptosis in human AML 14.2D10 cells (22). Guo et al. found that GSPE inhibits the proliferation of cells of the ECA109 esophageal squamous cancer cell line; apoptosis was reported to be induced via the activation of caspase-3 and attenuation of the activation of the NF-κB signaling pathway (18). In ECA 109 cells, GSPE was also found to promote Bax expression and decrease Bcl-2 expression in both time- and dose-dependent manners (18). Liu et al. reported that GSPE-induced apoptosis in cells of the BIU87 human bladder cancer line is associated with increased caspase-3 activity (23). GSPE was found to increase rates of apoptosis in the MIA PaCa-2 pancreatic adenocarcinoma cell line via the downregulation of Bcl-2 (30). In addition, GSPE was reported to induce apoptosis in the CaCo2 colorectal cancer cell line via a mechanism associated with the attenuation of the PI3K/PKB pathway (21). However, Ruan et al. (32) reported that GSPE is associated with decreased Bax expression and increased Bcl-2 expression, thus playing a critical role in the inhibition of H9C2 cell apoptosis after hypoxic culturing via the PI3K/AKT signaling pathway. Further beneficial effects exerted by GSPE include the prevention of bone loss induced by inflammation via a decrease in the phosphorylation and degradation of NF-κB inhibitor and inhibition of apoptosis via a decrease in the activity of caspases-3 and -9 (33).
Here, we confirmed that GSPE directly induces HL-60/ADR cell apoptosis via an upregulation of Bax expression, a downregulation of Bcl-2 expression and an increase in both caspase-3 and caspase-9 activity.
There were several limitations in this study. Firstly, this study was an extension of our previous work (14). We did not obtain additional funds for a detailed exploration of the apoptotic mechanism in this study; no western blot analysis of protein concentrations was carried out. In addition, we likewise did not study a number of other important anti- and pro-apoptotic proteins due to lack of funds. Funding was insufficient to support animal experimentation and in vivo validation. The aforementioned limitations would certainly be eliminated if sufficient funding was obtained by our group in the future.
Conclusions
The present study demonstrated that GSPE induces apoptosis of HL-60/ADR cells via the Bcl-2/Bax caspase-3/9 pathway. GSPE can not only be used as an adjunct in the setting of combination chemotherapy to reduce drug resistance, but also to directly inhibit tumor cell proliferation. A further toxicity study of GSPE in non-tumoral proliferating cells is thus warranted to evaluate its potential uses in clinic.
Acknowledgments
We thank Professor Qi-Hua Fu and Professor Qiu-Hui Pan from the National Children’s Medical Center (Shanghai) for providing us an experimental site as well as technical guidance. We would like to thank Dr. Erik Matro for his help in polishing our paper.
Funding: This work was supported by
Footnote
Reporting Checklist: The authors have completed the MDAR checklist. Available at https://dx.doi.org/10.21037/tcr-21-920
Data Sharing Statement: Available at https://dx.doi.org/10.21037/tcr-21-920
Peer Review File: Available at https://dx.doi.org/10.21037/tcr-21-920
Conflicts of Interest: All authors have completed the ICMJE uniform disclosure form (available at https://dx.doi.org/10.21037/tcr-21-920). The authors have no conflicts of interest to declare.
Ethical Statement: The authors are accountable for all aspects of the work in ensuring that questions related to the accuracy or integrity of any part of the work are appropriately investigated and resolved. The study was conducted in accordance with the Declaration of Helsinki (as revised in 2013). Institutional ethical approval and informed consent were waived.
Open Access Statement: This is an Open Access article distributed in accordance with the Creative Commons Attribution-NonCommercial-NoDerivs 4.0 International License (CC BY-NC-ND 4.0), which permits the non-commercial replication and distribution of the article with the strict proviso that no changes or edits are made and the original work is properly cited (including links to both the formal publication through the relevant DOI and the license). See: https://creativecommons.org/licenses/by-nc-nd/4.0/.
References
- Short NJ, Rytting ME, Cortes JE. Acute myeloid leukaemia. Lancet 2018;392:593-606. [Crossref] [PubMed]
- Döhner H, Weisdorf DJ, Bloomfield CD. Acute Myeloid Leukemia. N Engl J Med 2015;373:1136-52. [Crossref] [PubMed]
- Zjablovskaja P, Florian MC. Acute Myeloid Leukemia: Aging and Epigenetics. Cancers (Basel) 2019;12:103. [Crossref] [PubMed]
- Zwaan CM, Kolb EA, Reinhardt D, et al. Collaborative Efforts Driving Progress in Pediatric Acute Myeloid Leukemia. J Clin Oncol 2015;33:2949-62. [Crossref] [PubMed]
- Sekeres MA, Guyatt G, Abel G, et al. American Society of Hematology 2020 guidelines for treating newly diagnosed acute myeloid leukemia in older adults. Blood Adv 2020;4:3528-49. [Crossref] [PubMed]
- Döhner H, Estey E, Grimwade D, et al. Diagnosis and management of AML in adults: 2017 ELN recommendations from an international expert panel. Blood 2017;129:424-47. [Crossref] [PubMed]
- Siveen KS, Uddin S, Mohammad RM. Targeting acute myeloid leukemia stem cell signaling by natural products. Mol Cancer 2017;16:13. [Crossref] [PubMed]
- González-Quilen C, Grau-Bové C, Jorba-Martín R, et al. Protective properties of grape-seed proanthocyanidins in human ex vivo acute colonic dysfunction induced by dextran sodium sulfate. Eur J Nutr 2021;60:79-88. [Crossref] [PubMed]
- Margalef M, Pons Z, Iglesias-Carres L, et al. Gender-related similarities and differences in the body distribution of grape seed flavanols in rats. Mol Nutr Food Res 2016;60:760-72. [Crossref] [PubMed]
- Xu M, Chen X, Huang Z, et al. Grape seed proanthocyanidin extract promotes skeletal muscle fiber type transformation via AMPK signaling pathway. J Nutr Biochem 2020;84:108462. [Crossref] [PubMed]
- Casanova-Martí À, González-Abuín N, Serrano J, et al. Long Term Exposure to a Grape Seed Proanthocyanidin Extract Enhances L-Cell Differentiation in Intestinal Organoids. Mol Nutr Food Res 2020;64:e2000303. [Crossref] [PubMed]
- Grau-Bové C, González-Quilen C, Terra X, et al. Effects of Flavanols on Enteroendocrine Secretion. Biomolecules 2020;10:844. [Crossref] [PubMed]
- Liu M, Yun P, Hu Y, et al. Effects of Grape Seed Proanthocyanidin Extract on Obesity. Obes Facts 2020;13:279-91. [Crossref] [PubMed]
- Lin KN, Jiang YL, Zhang SG, et al. Grape seed proanthocyanidin extract reverses multidrug resistance in HL-60/ADR cells via inhibition of the PI3K/Akt signaling pathway. Biomed Pharmacother 2020;125:109885. [Crossref] [PubMed]
- Wang K, Wei H, Zhan J, et al. GSPE alleviates renal fibrosis by inhibiting the activation of C3/ HMGB1/ TGF-β1 pathway. Chem Biol Interact 2020;316:108926. [Crossref] [PubMed]
- Tu X, Wang M, Liu Y, et al. Pretreatment of Grape Seed Proanthocyanidin Extract Exerts Neuroprotective Effect in Murine Model of Neonatal Hypoxic-ischemic Brain Injury by Its Antiapoptotic Property. Cell Mol Neurobiol 2019;39:953-61. [Crossref] [PubMed]
- Odai T, Terauchi M, Kato K, et al. Effects of Grape Seed Proanthocyanidin Extract on Vascular Endothelial Function in Participants with Prehypertension: A Randomized, Double-Blind, Placebo-Controlled Study. Nutrients 2019;11:2844. [Crossref] [PubMed]
- Guo F, Hu Y, Niu Q, et al. Grape Seed Proanthocyanidin Extract Inhibits Human Esophageal Squamous Cancerous Cell Line ECA109 via the NF-κB Signaling Pathway. Mediators Inflamm 2018;2018:3403972. [Crossref] [PubMed]
- Sherif AA, Abdelhalim SZ, Salim EI. Immunohistochemical and biochemical alterations following administration of proanthocyanidin extract in rats hepatocellular carcinoma. Biomed Pharmacother 2017;93:1310-9. [Crossref] [PubMed]
- Song X, Siriwardhana N, Rathore K, et al. Grape seed proanthocyanidin suppression of breast cell carcinogenesis induced by chronic exposure to combined 4-(methylnitrosamino)-1-(3-pyridyl)-1-butanone and benzoapyrene. Mol Carcinog 2010;49:450-63. [Crossref] [PubMed]
- Engelbrecht AM, Mattheyse M, Ellis B, et al. Proanthocyanidin from grape seeds inactivates the PI3-kinase/PKB pathway and induces apoptosis in a colon cancer cell line. Cancer Lett 2007;258:144-53. [Crossref] [PubMed]
- Hu H, Qin YM. Grape seed proanthocyanidin extract induced mitochondria-associated apoptosis in human acute myeloid leukaemia 14.3D10 cells. Chin Med J (Engl) 2006;119:417-21. [Crossref] [PubMed]
- Liu J, Zhang WY, Kong ZH, et al. Induction of cell cycle arrest and apoptosis by grape seed procyanidin extract in human bladder cancer BIU87 cells. Eur Rev Med Pharmacol Sci 2016;20:3282-91. [PubMed]
- Ye X, Krohn RL, Liu W, et al. The cytotoxic effects of a novel IH636 grape seed proanthocyanidin extract on cultured human cancer cells. Mol Cell Biochem 1999;196:99-108. [Crossref] [PubMed]
- Prasain JK, Peng N, Dai Y, et al. Liquid chromatography tandem mass spectrometry identification of proanthocyanidins in rat plasma after oral administration of grape seed extract. Phytomedicine 2009;16:233-43. [Crossref] [PubMed]
- Monagas M, Quintanilla-López JE, Gómez-Cordovés C, et al. MALDI-TOF MS analysis of plant proanthocyanidins. J Pharm Biomed Anal 2010;51:358-72. [Crossref] [PubMed]
- Creagh EM, Conroy H, Martin SJ. Caspase-activation pathways in apoptosis and immunity. Immunol Rev 2003;193:10-21. [Crossref] [PubMed]
- van Delft MF, Huang DC. How the Bcl-2 family of proteins interact to regulate apoptosis. Cell Res 2006;16:203-13. [Crossref] [PubMed]
- Choi JH, Bogenberger JM, Tibes R. Targeting Apoptosis in Acute Myeloid Leukemia: Current Status and Future Directions of BCL-2 Inhibition with Venetoclax and Beyond. Target Oncol 2020;15:147-62. [Crossref] [PubMed]
- Cedó L, Castell-Auví A, Pallarès V, et al. Gallic acid is an active component for the anticarcinogenic action of grape seed procyanidins in pancreatic cancer cells. Nutr Cancer 2014;66:88-96. [Crossref] [PubMed]
- Gao N, Budhraja A, Cheng S, et al. Induction of apoptosis in human leukemia cells by grape seed extract occurs via activation of c-Jun NH2-terminal kinase. Clin Cancer Res 2009;15:140-9. [Crossref] [PubMed]
- Ruan Y, Jin Q, Zeng J, et al. Grape Seed Proanthocyanidin Extract Ameliorates Cardiac Remodelling After Myocardial Infarction Through PI3K/AKT Pathway in Mice. Front Pharmacol 2020;11:585984. [Crossref] [PubMed]
- Kwak SC, Cheon YH, Lee CH, et al. Grape Seed Proanthocyanidin Extract Prevents Bone Loss via Regulation of Osteoclast Differentiation, Apoptosis, and Proliferation. Nutrients 2020;12:3164. [Crossref] [PubMed]