Benefits of intraoral stents for sparing normal tissue in radiotherapy of nasopharyngeal carcinoma: a radiobiological model-based quantitative analysis
Introduction
Nasopharyngeal carcinoma (NPC) is a kind of head and neck cancers which is particularly prevalent in southern China and Southeast Asia (1). Radiotherapy (RT) plays an efficient role in the treatment of NPC with successful results (2). However, radiation-induced injury has still been observed and is frequently associated with normal tissue complications, which may affect prognosis for patients and reduce quality of life (3-5). Although advances in intensity-modulated radiotherapy (IMRT) have allowed greater sparing of normal tissues, yet oral-related injuries remain a major challenge and, therefore, several strategies have been adopted to reduce the risk of oral complications.
Recent studies have documented the advantage of using intraoral stent and other similar devices for accurate positioning during RT and sparing normal adjacent tissue (6,7). Using this device can increase the distance between the mandible and the maxilla, thus minimizing the risk of radiation-induced complications in normal oral tissue (8). By analyzing the dosimetric parameters, prior studies have found that intraoral stent was effective in decreasing dose to normal tissues (9-11). However, it is difficult to quantify the radiation-induced toxicities by a dosimetry analysis alone.
Radiobiological model, which uses the dose-volume histogram (DVH), allows for quantification of normal tissue complication probability (NTCP) (12-15) and is widely used in clinical decision making (16), treatment technique selection (17), and planning optimization (18). However, few studies have attempted to quantify the benefit of intraoral stent at normal tissue sparing by using these radiobiological models. Therefore, the purpose of our study was to quantify the effect of intraoral stent on radiation-induced normal tissue toxicity and tumor control probability (TCP) in patients with NPC receiving concurrent chemo-radiotherapy (CCRT) using radiobiological model analysis. We present the following article in accordance with the STROBE reporting checklist (available at https://dx.doi.org/10.21037/tcr-21-1324).
Methods
Patient characteristics
The eligibility criteria for patient enrollment are as follows: (I) biopsy-proven NPC; (II) Helical Tomo radiotherapy (RT) was administered at Nanjing Drum Tower Hospital; (III) completion of planned RT treatment; (IV) plan CT and RT dose data at enrollment were available. Between October 2015 and July 2020, a total of thirty-three patients with NPC who underwent Helical Tomo radiotherapy at Nanjing Drum Tower Hospital were evaluated and divided into 2 groups: with intraoral stent (group 1, n=16) and without intraoral stent (group 2, n=17). The study was conducted in accordance with the Declaration of Helsinki (as revised in 2013). The study was approved by the Ethics Committee of Nanjing Stomatological Hospital, Medical School of Nanjing University (No. NJSH-2021NL-041) and individual consent for this retrospective analysis was waived.
For group 1 (with intraoral stent), the NPC patients wearing a customized intraoral stent during RT are the protocol implemented by our center from November 2019.
For group 2 (without intraoral stent), the NPC patients who did not wear an intraoral stent due to limitations (e.g., trismus or limited jaw opening) or patients received RT before November 2019.
The patient general characteristics were described in Table 1.
Table 1
Characteristic | Patients with oral stents (n=16) | Patients without oral stents (n=17) | P |
---|---|---|---|
Gender | 0.605 | ||
Male | 12 | 14 | |
Female | 4 | 3 | |
Age (years) | 0.215 | ||
Median [range] | 52 [29–75] | 47 [23–68] | |
AJCC staging | 0.576 | ||
III | 13 | 15 | |
IVa | 3 | 2 |
NPC, nasopharyngeal carcinoma.
Construction of the custom-made intraoral stent
Construction of the custom-made intraoral stent contained the following steps, which were applied to the patient cohort receiving it. Firstly, oral examinations were performed and unretainable decayed teeth were extracted. The maxillary and mandibular impressions were then taken from the patients’ dentitions. Next, wax occlusal dike was used to transfer maxillo-mandibular relationship. Subsequently, the wax patterns were made on the articulator. Finally, after packing, plastic filling, curing, and polishing, the production customized intraoral stent was completed. Figure 1 showed the customized intraoral stent used in patients with NPC.
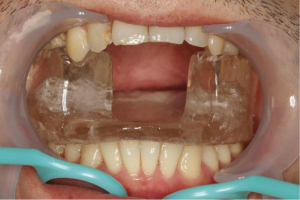
Target delineation and IMRT planning
Patients were placed in supine position and immobilized using a thermoplastic mask against head and shoulder. Planning CT scans were performed by a Siemens SOMATOM Definition CT scanner (Siemens, Ehrlangen, Germany) using standard scan parameters: 120 kVp, 120 mA, 5 mm slice thickness, 0.8×0.8 pixel spacing. The acquired CT images were transferred to MIM Meastro (MIM Software, Inc., Cleveland, OH, USA) and the regions of interest were contoured.
The contrast-enhanced CT and magnetic resonance (MR) images were fused to the planning CT images and the targets were delineated by both experienced radiologists and radiation oncologists. The delineation principle of tumor target was the same as those described in our previous work (19). The gross tumor volume (GTV) including the primary tumor and involved lymph nodes identified by imaging, clinical examination, as well as endoscopic findings. The clinical target volume (CTV) was determined to include regions that have a high risk of microscopic tumor involvement. The planning target volume (PTV) was constructed by adding an additional margin of 3–5 mm to the CTV according to the immobilization and localization uncertainties.
IMRT was administered using Helical Tomotherapy (HT), for which plans were designed by Tomo treatment planning system (Accuray, Inc., USA). The doses were prescribed to cover at least 95% of PTV while meeting the dose constraint for organs at risk (OAR) (20). All HT plans were optimized with the same prescription dose and same dose constraint for OARs.
Dosimetry analysis
For PTVs, the conformity index (CI) and homogeneity index (HI) were used to compare two groups of HT plans (with or without intraoral stent) (21,22).
For OARs, mean dose (Dmean) to mandible, temporal-mandibular joint (TMJ), parotid, and submandibular gland were recorded for the two groups. Besides, according to Eisbruch et al. (23), the surfaces of the inner lips, buccal mucosa, tongue, base of tongue, floor of mouth, and palate were countered as a distinct organ, namely the oral cavity. The mean dose to the oral cavity was also evaluated and recorded.
TCP and NTCP
Differential dose volume histogram (DVH) at 0.01 Gy interval of each patient was exported to RADBIOMOD version 0.3b (14) for TCP and NTCP calculations.
TCP calculation
The TCP was calculated using the modified Webb-Nahum model by Avazon et al. (24), which is based on Poisson statistics and the linear-quatratic (LQ) model. The model assumes the number of clonogens in the tumor is Poisson-distributed and calculates the probability of no viable clonogens left after a course of RT. The model can be described as follows:
The meanings and sources of the parameters used in this TCP model are summarized in Table 2.
Table 2
Parameters (unit) | Average value of uniform distribution |
---|---|
α(Gy−1) | 0.33 |
σα(Gy−1) | 0.07 |
α/β(Gy) | 10 |
ρ(cm−3) | 107 |
Tk(days) | 28 |
Tpot(days) | 3 |
HF | 0.22 |
OER | 1.5 |
Dose per fraction (Gy) | 2 |
Fractions/week | 5 |
Prescription dose (Gy) | 70 |
α and β, intrinsic radiosensitivity parameters of tumor cell in hit of single-hit and double-hit effect; σα, standard deviation of α; ρ, clonogenic cell density; Tk, kick-off time; Tpot, potential doubling time; TCP, tumor control probability; HF, hypoxic fraction; OER, oxygen enhancement ratio. The values listed in this table are sourced from Avanzo et al. (24).
NTCP calculation
We adopted a parallel architecture model (25) fitted by Bhide et al. (12), to calculate the NTCP of the oral cavity. The LKB models fitted by Burman et al. (26) and Semenenko et al. (27), were employed to calculate the NTCP of the parotid gland and TMJ, respectively.
The parallel architecture model is a logistic function and described as follow:
The LKB model consisting of three equations:
Details about the meanings and sources of these parameters can be seen in Table 3.
Table 3
OAR | LKB model parameters | Parallel model parameters | Clinical endpoint | α/β | ||||
---|---|---|---|---|---|---|---|---|
n | m | TD50 | k | TD50 | ||||
Parotid | 1 | 0.53 | 31.4Gy | – | – | Xerostomia expressed as reduction in stimulated salivary flow at 6 months after radiotherapy (26) | 3 (27) | |
TMJ | 0.07 | 0.1 | 72 Gy | – | – | Marked limitation of joint function (25) | 2 (26) | |
Oral cavity | – | – | – | 1 | 51 Gy | Grade 3 oral mucositis (12) | 10 (12) |
NTCP, normal tissue complications probability; OAR, organ at risk; n, volume dependence of the complication probability; m, slope of the dose-response curve; TD50, dose at which 50% of patients experience toxicity; k, slope of the dose-response curve. The values listed in this table are sourced from Semenenko et al. (27), Burman et al. (26), and Bhide et al. (12).
Statistical analysis
Statistical analysis was performed using unpaired Mann-Whitney U test by statistical software GraphPad Prism version 8.3.0 (GraphPad Software Inc., San Diego, CA, USA), to compare 2 groups. P<0.05 was considered statistically significant.
Results
Comparison of CI and HI of the tumor target
As shown in Table 4, the differences in CI and HI between the two groups (with vs. without intraoral stent) were not statistically significant (CI, P=0.056; HI, P=0.676), which means that intraoral stent has no significant effect on target dose distribution. Figure 2 shows the dose distribution of tumor target.
Table 4
Parameters | Patients with oral stents | Patients without oral stents | P |
---|---|---|---|
CI | 0.863±0.032 | 0.844±0.021 | 0.056 |
HI | 0.105±0.145 | 0.067±0.014 | 0.676 |
CI, conformity index; HI, homogeneity index; PTV, planning target volume.
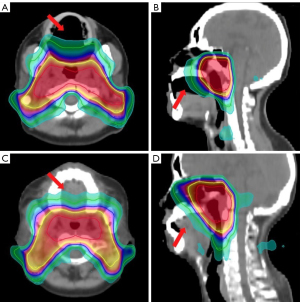
Comparison of dosimetry metrics of the critical structures
The dosimetry metrics of critical structures are summarized in Table 5. Compared with patients without intraoral stent, the mean dose (Dmean) to oral cavity, mandible, and bilateral parotid were significantly lower in patients use intraoral stent (P<0.05 for all). The Dmean of bilateral TMJ and submandibular glad was slightly lower in patients with intraoral stent, although it did not reach statistical significance (Table 5, Figure 3).
Table 5
OAR | Patients with oral stents (Dmean, Gy) | Patients without oral stents (Dmean, Gy) | P |
---|---|---|---|
Oral cavity | 32.98±1.91 | 37.31±2.79 | <0.001† |
Mandible | 34.63±3.22 | 39.46± 2.52 | <0.001† |
TMJ-L | 38.64±7.01 | 42.71±4.75 | 0.072 |
TMJ-R | 36.92±7.63 | 39.17±4.07 | 0.213 |
Parotid-L | 30.4±3.33 | 35.93±4.17 | <0.001† |
Parotid-R | 29.42±2.25 | 32.25±2.36 | 0.001† |
Submandibular gland-L | 54.01±7.09 | 56.40±6.77 | 0.443 |
Submandibular gland-R | 52.33±6.74 | 55.73±5.86 | 0.191 |
†, P<0.05 were considered significant. OAR, organ at risk; Dmean, mean dose; TMJ-R, right temporal-mandibular joint; TMJ-L, left temporal-mandibular joint.
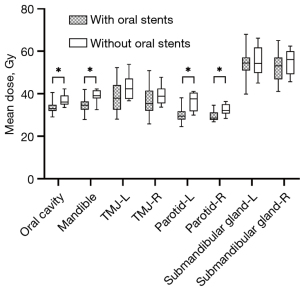
Comparison of TCP of the tumor target and NTCP of the critical structures
In Figure 4, as well as Table 6, we show the results of TCP and NTCP analysis. For TCP of tumor targets, no statistically significant differences were observed between the two groups (P=0.056), indicating that whether or not to use an intraoral stent has no significant effect on tumor control. As shown for NTCP, complication probability of oral cavity (P<0.001) was significantly decreased in patients with intraoral stent, and there was also a strong trend toward a decrease in parotids (P<0.001). Furthermore, in NTCP analysis of bilateral TMJ, no statistical differences were observed (P=0.841).
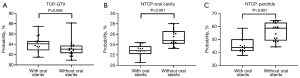
Table 6
Structure | Patients with oral stents (%) | Patients without oral stents (%) | P |
---|---|---|---|
TCP | |||
GTV | 84.02±1.57 | 83.18±1.56 | 0.056 |
NTCP | |||
Oral cavity | 22.83±1.12 | 25.35±1.55 | <0.001† |
TMJ | 0.43±0.53 | 0.22±0.62 | 0.841 |
Parotids | 45.83±6.30 | 55.94±6.85 | <0.001† |
†, P<0.05 were considered significant. TCP, tumor control probability; NTCP, normal tissue complications probability; GTV, gross tumor volume; TMJ-R, right temporal-mandibular joint; TMJ-L, left temporal-mandibular joint.
Discussion
Radiation-induced oral complications are common and significantly affect the quality of life of patients. Prior work has documented the effectiveness of an intraoral stent in reducing oral-related toxicity in head and neck cancer patients treated with radiotherapy. The major advantages of the intraoral stents were reproducible positioning and minimizing the radiotoxicity of normal structures. Feng et al. (11) and Verrone et al. (8,9), for example, report that wearing an intraoral stent can effectively reduce the dose to oral tissues. However, few studies have quantitatively assessed the effect of wearing intraoral stents on complication risk and tumor control rate based on dose-response analysis. Quantitative descriptors of dose-response curve can be generated by radiobiological models and used for comparison between different regimens (e.g., with or without intraoral stents). In this study, we adopted the radiobiological models, including the LKB (26,27) and parallel architecture (12) NTCP models, as well as the modified Webb-Nahum TCP model (24), to quantitatively explore the value of customized intraoral stent for sparing normal tissue during radiotherapy for NPC.
The method used in previous work for evaluating the benefits of oral stents is to assess the dosimetric parameters derived from the DVH (e.g., Dmean) (8,9,11). In agreement with previous work, dosimetric analysis of this study showed that patients who wore the intraoral stent had a decreasing trend in radiation dose to adjacent normal tissue, especially for oral cavity, mandible and bilateral parotid, which may be due to the intraoral stent increased distance between the mandible and the maxilla. In addition, the dose distribution characteristics (CI and HI), showed no significant differences between the two groups, suggesting that customized intraoral stent showed no significant effect on target dose coverage. However, this sole assessment of dosimetric parameters is a very simple method to quantify the effect of intraoral stents and has some limitations. Namely the previous studies were unable to make full use of DVH and radio-sensitivity factors to evaluate the reduction of radiotoxicity burden and the impact of tumor control by using intraoral stents.
Radio-biological models have been proposed as a way to overcome these limitations. In this work, we applied radiobiological models to investigate the protective value of wearing intraoral stent. As for the comparison of oral mucositis risk between the two groups, we used the parallel architecture model, which assumed that the organ (e.g., oral cavity) consist of numerous subunits that respond to radiation independently. The model is defined as a sigmoid function that describes the probability of damaging a subunit at a given bioequivalent dose. We found that patients wearing intraoral stent significantly reduced grade 3 oral mucositis complication probability by 2.52% on average compared to unused ones (NTCPmean: 22.83% vs. 25.35%). Similar to parallel architecture model, the LKB model is also widely used for assessing the radiotoxicity and the model parameters are available from the previous work. The result showed that the incidence of xerostomia for parotids were significant lower in oral stents group than in the control group, achieved a reduction of 10.11% (NTCPmean: 45.83% vs. 55.94%). Apart from NTCP analysis, a Webb-Nahum Poisson TCP model was employed for assessing the tumor control of the two groups. The Webb-Nahum model assumes that the number of surviving clonogens is Poisson-distributed, by which the probability of no surviving clonogens in the tumor after radiotherapy could be calculated. In this work, we choose a modified Webb-Nahum model that incorporates additional radiobiological factors including radiosensitivity, hypoxia, and repopulation. Our findings showed that wearing the intraoral stent is independent of tumor control (P>0.05). In this way, instead of assessing multiple dosimetric parameters, a single NTCP and TCP indicators could be used to quantify the advantage of wearing an intraoral stent. These radiobiological indicators could replace the dosimetric parameters in order to closely reflect the clinical goals of using oral stents in radiotherapy.
In clinical practice, the goal of radiotherapy plan evaluation is to achieve the best compromise, including a low complication probability value (NTCP) and a high TCP. The findings of this study extend the previous work (8,9,11), confirming that the intraoral stent was effective for reducing the risk of oral complications without compromising tumor control. Most notably, this is the first study to our knowledge to investigate the protective value of wearing intraoral stent using radiobiological models. Our results provide quantitative evidence for the value of intraoral stent at sparing normal tissue and suggest that this device appears to be effective in normal tissue protection for patients with NPC during radiotherapy.
Several limitations are worth noting in present study, namely its retrospective study design in small sample size and the sample was not reassessed once after the end of treatment. Future study should therefore include follow-up work designed to evaluate the patients’ quality of life and validate performance of the radiobiological model in a large cohort. In addition, radiobiological models published by other groups contain different types and parameters, each of which may affect the prediction result and limit their generalizability. Besides, although the IMRT plans with or without oral stents were designed by the same experienced physicist (Juan Liu) using the TomoTherapy treatment planning system, the degree of familiarity and techniques of planning skills would refine gradually as time progresses, which may have a potential impact on dosimetry results.
Despite these current challenges and limitations, the potential for intraoral stents is immense. It is widely recognized that benefits associated with wearing intraoral stent for radiotherapy is evident. Moreover, the radiobiological models could be useful in estimating the reduction of radiotoxicity burden that might be guided as part of tumor dose escalation protocols.
In conclusion, radiobiological model analysis demonstrated the potential value of custom-made intraoral stent at reducing complication risk during radiotherapy for NPC without compromising target dose coverage or tumor control.
Acknowledgments
We would like to thank Tong Bai (Department of Radiotherapy, Shandong Tumor Hospital &Institute) for her help in polishing our paper.
Footnote
Funding: This study was supported by
Reporting Checklist: The authors have completed the STROBE reporting checklist. Available at https://dx.doi.org/10.21037/tcr-21-1324
Data Sharing Statement: Available at https://dx.doi.org/10.21037/tcr-21-1324
Conflicts of Interest: All authors have completed the ICMJE uniform disclosure form (available at https://dx.doi.org/10.21037/tcr-21-1324). The authors have no conflicts of interest to declare.
Ethical Statement: The authors are accountable for all aspects of the work in ensuring that questions related to the accuracy or integrity of any part of the work are appropriately investigated and resolved. The study was conducted in accordance with the Declaration of Helsinki (as revised in 2013). The study was approved by the Ethics Committee of Nanjing Stomatological Hospital, Medical School of Nanjing University (No. NJSH-2021NL-041) and individual consent for this retrospective analysis was waived.
Open Access Statement: This is an Open Access article distributed in accordance with the Creative Commons Attribution-NonCommercial-NoDerivs 4.0 International License (CC BY-NC-ND 4.0), which permits the non-commercial replication and distribution of the article with the strict proviso that no changes or edits are made and the original work is properly cited (including links to both the formal publication through the relevant DOI and the license). See: https://creativecommons.org/licenses/by-nc-nd/4.0/.
References
- Wei KR, Zheng RS, Zhang SW, et al. Nasopharyngeal carcinoma incidence and mortality in China, 2013. Chin J Cancer 2017;36:90. [Crossref] [PubMed]
- Chen L, Hu CS, Chen XZ, et al. Concurrent chemoradiotherapy plus adjuvant chemotherapy versus concurrent chemoradiotherapy alone in patients with locoregionally advanced nasopharyngeal carcinoma: a phase 3 multicentre randomised controlled trial. Lancet Oncol 2012;13:163-71. [Crossref] [PubMed]
- De Sanctis V, Bossi P, Sanguineti G, et al. Mucositis in head and neck cancer patients treated with radiotherapy and systemic therapies: Literature review and consensus statements. Crit Rev Oncol Hematol 2016;100:147-66. [Crossref] [PubMed]
- Sroussi HY, Epstein JB, Bensadoun RJ, et al. Common oral complications of head and neck cancer radiation therapy: mucositis, infections, saliva change, fibrosis, sensory dysfunctions, dental caries, periodontal disease, and osteoradionecrosis. Cancer Med 2017;6:2918-31. [Crossref] [PubMed]
- Wang X, Hu C, Eisbruch A. Organ-sparing radiation therapy for head and neck cancer. Nat Rev Clin Oncol 2011;8:639-48. [Crossref] [PubMed]
- Inoue Y, Yamagata K, Nakamura M, et al. Are Intraoral Stents Effective for Reducing the Severity of Oral Mucositis During Radiotherapy for Maxillary and Nasal Cavity Cancer? J Oral Maxillofac Surg 2020;78:1214.e1-8. [Crossref] [PubMed]
- Alves LDB, Menezes ACS, Pereira DL, et al. Benefits of intraoral stents in patients with head and neck cancer undergoing radiotherapy: Systematic review. Head Neck 2021;43:1652-63. [Crossref] [PubMed]
- Verrone JR, Alves Fde A, Prado JD, et al. Impact of intraoral stent on the side effects of radiotherapy for oral cancer. Head Neck 2013;35:E213-7. [Crossref] [PubMed]
- Verrone JR, Alves FA, Prado JD, et al. Benefits of an intraoral stent in decreasing the irradiation dose to oral healthy tissue: dosimetric and clinical features. Oral Surg Oral Med Oral Pathol Oral Radiol 2014;118:573-8. [Crossref] [PubMed]
- Chen D, Chen X, Chen X, et al. The efficacy of positioning stents in preventing Oral complications after head and neck radiotherapy: a systematic literature review. Radiat Oncol 2020;15:90. [Crossref] [PubMed]
- Feng Z, Wang P, Gong L, et al. Construction and clinical evaluation of a new customized bite block used in radiotherapy of head and neck cancer. Cancer Radiother 2019;23:125-31. [Crossref] [PubMed]
- Bhide SA, Gulliford S, Schick U, et al. Dose-response analysis of acute oral mucositis and pharyngeal dysphagia in patients receiving induction chemotherapy followed by concomitant chemo-IMRT for head and neck cancer. Radiother Oncol 2012;103:88-91. [Crossref] [PubMed]
- Marks LB, Yorke ED, Jackson A, et al. Use of normal tissue complication probability models in the clinic. Int J Radiat Oncol Biol Phys 2010;76:S10-9. [Crossref] [PubMed]
- Chang JH, Gehrke C, Prabhakar R, et al. RADBIOMOD: A simple program for utilising biological modelling in radiotherapy plan evaluation. Phys Med 2016;32:248-54. [Crossref] [PubMed]
- Dean JA, Wong KH, Welsh LC, et al. Normal tissue complication probability (NTCP) modelling using spatial dose metrics and machine learning methods for severe acute oral mucositis resulting from head and neck radiotherapy. Radiother Oncol 2016;120:21-7. [Crossref] [PubMed]
- Lambin P, Roelofs E, Reymen B, et al. 'Rapid Learning health care in oncology' - an approach towards decision support systems enabling customised radiotherapy'. Radiother Oncol 2013;109:159-64. [Crossref] [PubMed]
- Langendijk JA, Lambin P, De Ruysscher D, et al. Selection of patients for radiotherapy with protons aiming at reduction of side effects: the model-based approach. Radiother Oncol 2013;107:267-73. [Crossref] [PubMed]
- Kierkels RG, Korevaar EW, Steenbakkers RJ, et al. Direct use of multivariable normal tissue complication probability models in treatment plan optimisation for individualised head and neck cancer radiotherapy produces clinically acceptable treatment plans. Radiother Oncol 2014;112:430-6. [Crossref] [PubMed]
- Li S, Wang K, Hou Z, et al. Use of Radiomics Combined With Machine Learning Method in the Recurrence Patterns After Intensity-Modulated Radiotherapy for Nasopharyngeal Carcinoma: A Preliminary Study. Front Oncol 2018;8:648. [Crossref] [PubMed]
- Chang ATY, Hung AWM, Cheung FWK, et al. Comparison of Planning Quality and Efficiency Between Conventional and Knowledge-based Algorithms in Nasopharyngeal Cancer Patients Using Intensity Modulated Radiation Therapy. Int J Radiat Oncol Biol Phys 2016;95:981-90. [Crossref] [PubMed]
- Feuvret L, Noël G, Mazeron JJ, et al. Conformity index: a review. Int J Radiat Oncol Biol Phys 2006;64:333-42. [Crossref] [PubMed]
- Srivastava SP, Cheng CW, Das IJ. The dosimetric and radiobiological impact of calculation grid size on head and neck IMRT. Pract Radiat Oncol 2017;7:209-17. [Crossref] [PubMed]
- Eisbruch A, Kim HM, Terrell JE, et al. Xerostomia and its predictors following parotid-sparing irradiation of head-and-neck cancer. Int J Radiat Oncol Biol Phys 2001;50:695-704. [Crossref] [PubMed]
- Avanzo M, Stancanello J, Franchin G, et al. Correlation of a hypoxia based tumor control model with observed local control rates in nasopharyngeal carcinoma treated with chemoradiotherapy. Med Phys 2010;37:1533-44. [Crossref] [PubMed]
- Jackson A, Ten Haken RK, Robertson JM, et al. Analysis of clinical complication data for radiation hepatitis using a parallel architecture model. Int J Radiat Oncol Biol Phys 1995;31:883-91. [Crossref] [PubMed]
- Burman C, Kutcher GJ, Emami B, et al. Fitting of normal tissue tolerance data to an analytic function. Int J Radiat Oncol Biol Phys 1991;21:123-35. [Crossref] [PubMed]
- Semenenko VA, Li XA. Lyman-Kutcher-Burman NTCP model parameters for radiation pneumonitis and xerostomia based on combined analysis of published clinical data. Phys Med Biol 2008;53:737-55. [Crossref] [PubMed]