Dexmetomidine promotes the activity of breast cancer cells through miR-199a/HIF-1α axis
Introduction
The incidence of breast cancer is second only to cervical cancer in women, and with the gradual increase in incidence in recent years, breast cancer has become one of the foremost malignant tumors threatening the life and health of urban women (1,2). At present, clinicians mainly determine whether breast cancer is present according to the patient’s clinical signs, imaging manifestations, and related tumor markers. Early diagnosis is the key to improve the cure rate of breast cancer, but there is a lack of obvious clinical features during the early stages of breast cancer. In recent years, although important progress has been made in the treatment of breast cancer in the areas of surgery, radiotherapy, chemotherapy, and endocrine and targeted therapies, the prognosis of patients has not significantly improved. The molecular mechanisms of the occurrence and development of breast cancer need to be assessed further (3,4). Studies have confirmed that drugs play an important role in the progression of breast cancer. For example, flavonoids such as quercetin, as dietary substances of great concern to ordinary people, have potential as alternative or supplementary drugs for breast cancer (5). On the other hand, hyperin plays a role as an anticancer drug by activating the Bax-caspase-3 axis and inhibiting the NF-κB signal pathway through reactive oxygen species (ROS)-related apoptosis (6). Therefore, in-depth elucidation of the mechanisms of drugs in the treatment of breast cancer has important clinical significance.
Dexmetomidine (DMED) is a highly selective α2-adrenergic receptor agonist, which is mainly used as a sedative and analgesic in clinical practice. There is evidence that DMED can exert anti-apoptosis and protective functions on cells and organs (7,8). Studies have proven that DMED promotes metastasis in rodent models of breast, lung, and colon cancer (9). Also, Zhang et al. have shown that DMED inhibits the progression of esophageal cancer through the miR-143-3p/EPS8 axis (10). On the other hand, DMED promotes the migration of breast cancer cells through exocrine TMPRSS2 secretion mediated by Rab11 (11). Similarly, DMED could promote the proliferation, migration and invasion of breast cancer cells through the activation of α2B-adrenoceptor/ERK signaling (12). Whereas, the role of DMED in regulating the miRNA-mRNA axis in the malignant phenotype of breast cancer is not clear.
MicroRNA (miRNA) is an evolutionarily conserved non-coding RNA containing 19–22 nucleotides. It not only plays an important role in regulating the normal development of organisms but also participates in the regulation of the pathological processes of many types of malignant tumors (13). The role of miRNAs in the occurrence and development of breast cancer has been reported (14). For example, previous studies have shown that dual targeting of miR-124-3p and ABCC4 promotes the sensitivity of breast cancer cells to doxorubicin (15). Also, MiR-199a has been shown to inhibit the activity of breast cancer cells (16). Wu et al. have confirmed that DMED prevents oxidative damage to PC12 cells by regulating miR-199a/HIF-1α (17). At the same time, DMED attenuates neuronal injury induced by cerebral ischemia-reperfusion (IR) by regulating miR-199a (18). However, the role of DMED in regulating miR-199a in breast cancer is unknown. Moreover, Cao et al. have confirmed that HIF-1α can promote the activity of breast cancer cells (19). This shows that HIF-1α plays a cancer-promoting role in breast cancer. In view of the current state of research, in this study, MCF-7 and MDA-MB-231 breast cancer cell lines were treated with different concentrations of DMED to explore the role of DMED in promoting the activity of breast cancer cells through the miR-199a/HIF-1α axis. The results showed that DMED could inhibit the downregulation of miR-199a and promote the activity of breast cancer cells. Further experiments confirmed that DMED promoted the progression of breast cancer by regulating the miR-199a/HIF-1α axis.
We present the following article in accordance with the MDAR reporting checklist (available at https://dx.doi.org/10.21037/tcr-21-1937).
Methods
Cell culture
Human breast cancer cell lines (MCF-7 and MDA-MB-231) were purchased from ATCC (Rockville, IN, USA). The cells were cultured in RPMI-1640 complete medium (GIBCO, Carlsbad, CA, USA) containing 10% fetal bovine serum (FBS, HyClone, Logan, UT, USA) and 1% penicillin/streptomycin, cultured in an incubator at 37 °C and 5% CO2 saturated humidity; the medium was changed every 2–3 days. When the cells were close to fusion, they were digested and passaged with 0.25% trypsin (20). DMED purchased from Chen Xin Pharmaceutical Co., Ltd. DMED at different concentrations (0, 1, 10 and 100 nm) were added to the medium of breast cancer cells. After 24 hours of incubation, the medium was renewed.
Cell transfection
MCF-7 and MDA-MB-231 cells in logarithmic growth phase were inoculated on 6-well plates with a cell density of 5×105/well. When the cells had grown to 50% to 60%, the miR-199a mimics or miR-199a inhibitors of 100 nmol/L and the control group were transfected into MCF-7 and MDA-MB-231 cells according to the instructions of lipid Lipofectamine 2000 transfection reagents (ThermoFisherScience, Waltham, MA, USA). The cells were collected after incubation for 48 hours, and the transfection efficiency was detected by quantitative real-time polymerase chain reaction (QRT-PCR).
QRT-PCR
After the total RNA of cells in each group was extracted with Trizol reagent and the concentration was detected, it was reverse transcribed into cDNA according to the operation instructions of Takara kit, and amplified and detected. Reaction conditions: pre denaturation at 95 °C for 30s, denaturation at 95 °C for 5s, annealing/extension at 60 °C for 30s, a total of 40 cycles. The relative expression of target gene was calculated by 2−∆∆CT, ∆CT = target gene − β-actin, ∆∆ = ∆CT experiment − ∆CT control. As the internal references for miR-199a and HIF-1α mRNA, U6 and β-actin were employed, respectively. The primers were synthesized by Shanghai Shenggong Biology Co., Ltd. (Shanghai, China).
Detection of cell activity using 3-(4,5-dimethylthiazol-2-yl)-2,5-diphenyl-2H-tetrazolium bromide (MTT) assay
Cells in logarithmic growth phase were taken to prepare a 1×103/mL single cell suspension, 100 L per well, inoculated on a 96 well plate, 37 °C, 5% CO2, incubated in incubator, and after 24 hours of conventional culture, serum-free medium was used under aseptic conditions. Different reagents were added to the negative control group and interference group for cell transfection. The culture medium of each well was discarded and 20 µL MTT solution (5 mg/mL, MedChem Express, Monmouth Junction, NJ, USA) was added to each well, and the cells were cultured for 4 hours. The culture medium in the hole was removed, 150 µL dimethyl sulfoxide was added to each well, and it was put on the shaker and vibrated at low speed for 10 minutes to dissolve the crystal completely. Absorbance value was measured at 490 nm of each well with the microplate meter. The average value of six wells was taken as the result, and the absorbance value was used to express the cell proliferation. All experiments were performed 3 times in each group (21).
Caspase-3 activity detection
Steps were as follows: collection of cells, washing with phosphates buffered saline (PBS), extraction of cell lysate, protein quantification, sodium dodecyl (lauryl) sulfate-polyacrylamide gel (SDS)-PAGE electrophoresis, nitrocellulose membrane or polyvinylidene difluoride (PVDF) membrane transfer, 5% skimmed milk powder blocking for 2 hours, 4 °C overnight, caspase-3 poly-antibody or monoclonal antibody reaction at room temperature for 2 hours, and tris-buffered saline (TBS) (containing 0.05% Tween 20 TBS) washed 3 times, 5–10 min/times. Horseradish peroxidase (HRP)-labeled sheep anti-mouse immunoglobulin (IgG) or alkaline phosphatase (AP)-labeled sheep anti-mouse IgG reacted at room temperature, followed by washing 3 times with TBS and polysorbate 20, 5–10 min each time, ECL development or nitro blue tetrazolium/5-bromo-4-chloro-indolyl-phosphate (NBT/BCIP) staining.
Detection of cell migration ability by transwell
The transwell cell movement test was used to detect cell migration and invasion. In all, 2×104 cells/well were added to the upper transwell chamber, and 600 µL culture medium containing 20% FBS was added to the lower chamber and cultured at 37 °C. After 12 hours, the upper chamber cells were removed, fixed using 4% paraformaldehyde, stained with 0.1% crystal violet, dried, photographed and counted. All experiments were performed 3 times.
Detection of apoptosis by flow cytometry
Apoptosis was detected using annexin V-fluorescein isothiocyanate (FITC) double staining. Twenty-four hours after transfection, the cells were digested using trypsin, collected and inoculated in a 6-well plate. The cell density was adjusted to 2×106 cells per well. The cells were cultured for 24 hours, and the supernatant was discarded. After precooling the PBS washing twice, binding buffer was used to suspend cells. Human 5 µL annexin V-FITC and 5 µL propidium iodide (PI) were added to the cell suspension, and then incubated at room temperature for 15 minutes. Flow cytometry was used to detect the apoptosis rate within 1 hour, and the operation was performed according to the instructions of the kit. All experiments were performed 3 times.
Double luciferase reporter gene experiment
293T cells were digested with trypsin and re-suspended in a cell medium containing 10% FBS after centrifugation and inoculated on a 48-well plate at a density of 4×104 cells per well. After 24 hours of culture (when the cell density was 40% to 50%), the corresponding miR-199a expression vector (20 nmol/L), HIF-1α 3'UTR reporter vector (80 ng) and pRL-TK (expressing sea kidney luciferase protein as internal reference, 40 ng) were co-transfected into 293T cells using calcium phosphate transfection. Forty-eight hours after transfection, the cells were lysed with Dual-Luciferase Reporter Assay (Promega, Madison, WI, USA), and the luciferase activity was determined using a multi-function enzyme labeling instrument.
Western blot
After the cells were treated, the culture medium was abandoned, protein lysate (Roche, Basel, Switzerland) was added, and all proteins were separated. A total of 50 g of protein was added to 12% polyacrylamide gel and subjected to 100 V electrophoresis for 2 hours. It was then electrically transferred to a PVDF membrane. After sealing with 5% skimmed milk powder at room temperature for 1 hour, the membrane was washed 3 times with TBST, each time for 10 min and incubated with an antibody (concentration 1:1,000) overnight at 4 °C. After washing the membrane with TBST, the membrane was incubated at room temperature with HRP labeled anti-rabbit second antibody (concentration 1/2,000) for 1 h. Then, the membrane was washed 3 times with TBST, 10 min each time. Finally, western blot special reagent (Invitrogen, Waltham, MA, USA) was used for color imaging, and ImageJ was used to analyze the gray value of each protein. All experiments were performed 3 times.
Statistical analysis
The data were analyzed using SPSS 17.0 statistical software (SPSS Inc., Chicago, IL, USA). Measurement data were expressed using mean ± standard deviation (). Multiple factors were compared using one-way analysis of variance, and independent sample t-tests were used to compare two groups. If P<0.05, the difference between the two groups was considered significant.
Results
DMED induced cytotoxicity and apoptosis in breast cancer
The inhibitory effect of DMED on the growth of breast cancer cells and normal breast epithelial cells was detected using MTT assay. DMED promoted the growth of MCF-7 and MDA-MB-231 cells in a dose-dependent manner (Figure 1A). Flow cytometry analysis showed that the number of apoptotic MCF-7 and MDA-MB-231 cells decreased significantly after treatment with DMED (Figure 1B). The effect of DMED on apoptosis of MCF-7 and MDA-MB-231 cells was dose-dependent. The activation of caspase is important in the process of apoptosis (22). To determine whether DMED can change the activity of caspase in breast cancer cells, the activity of caspase-3 was determined. As shown in Figure 1C, DMED significantly increased the activity of caspase-3 in MCF-7 and MDA-MB-231 cells but had no effect on the activity of caspase-3 in mammary epithelial cells.
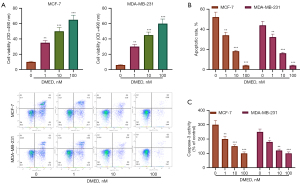
DMED down-regulated miR-199a expression
Then, we explored the effect of DMED on the level of miR-199a in breast cancer cells. QRT-PCR results showed that DMED downregulated the level of miR-199a in MCF-7 and MDA-MB-231 cells in a dose-dependent manner (P<0.05, Figure 2A,2B). Futhermore, DMED upregulated the level of HIF-1α in MCF-7 and MDA-MB-231 cells in a dose-dependent manner (P<0.05, Figure 2C,2D).
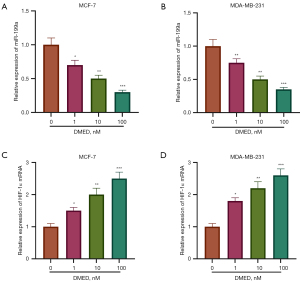
DMED increased the expression of miR-199a, downregulated the activity of breast cancer cells, and inhibited apoptosis
To investigate the effect of DMED on the activity and apoptosis of breast cancer cells, MiR-199a inhibitor was used to transfect MCF-7 and MDA-MB-231 cells. Results showed that miR-199a inhibitor was successfully transfected (P<0.05, Figure 3A). The results of MTT and transwell assays showed that the transfection of miR-199a inhibitor promoted the proliferation of breast cancer cells compared with the control group, and DMED promoted the proliferation of breast cancer cells compared with the miR-199a inhibitor group (P<0.05, Figure 3B,3C). On the other hand, the results of flow cytometry and caspase-3 activity assay showed that, compared with the control group, the transfection of miR-199a inhibitor reduced the apoptosis rate and the activity of caspase-3 in MCF-7 and MDA-MB-231 cells. Compared with the miR-199a inhibitor group, DMED decreased the apoptosis of breast cancer cells (P<0.05, Figure 3D,3E). This suggests that DMED further enhanced the downregulation of miR-199a to promote the activity of breast cancer cells and inhibit apoptosis.
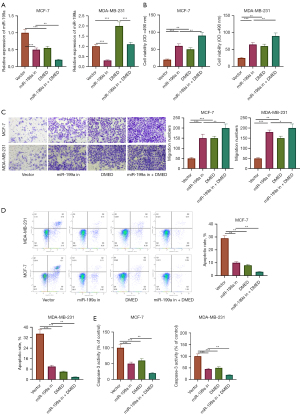
MiR-199a targeted HIF-1α
Subsequently, we explored the mechanism of miR-199a in breast cancer, screened the downstream targets of miR-199a through bioinformation database and showed that HIF-1α contained a conservative binding site of miR-199a (Figure 4A). For HIF-1α wild type reporter gene plasmid, the luciferase activity of miR-199a mimic transfection group was significantly lower than that of the negative control group (P<0.05). For HIF-1α mutant reporter gene plasmid, there was no significant difference in luciferase activity between the two groups (P>0.05, Figure 4B). Then, we explored the effect of DMED on the level of HIF-1α in breast cancer cells. Results from QRT-PCR showed that DMED upregulated the level of HIF-1α mRNA in MCF-7 and MDA-MB-231 cells in a dose-dependent manner (P<0.05, Figure 4C). On the other hand, the transfection of miR-199a mimics and inhibitors downregulated and upregulated the expression of HIF-1α in MCF-7 and MDA-MB-231 cells, respectively (P<0.05, Figure 4D). These results indicate that HIF-1α is the target of miR-199a and is negatively regulated by miR-199a.
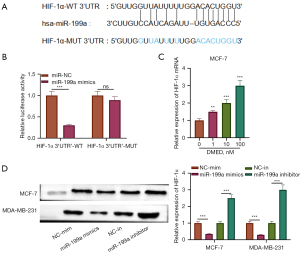
DMED promoted the progression of breast cancer through miR-199a/HIF-1α
Finally, the mechanism of DMED regulating miR-199a/HIF-1α axis to inhibit the progression of breast cancer was explored. The results of QRT-PCR showed that, compared with the control group, the expression of HIF-1α in DMED group was upregulated, the expression of HIF-1α in the HIF-1α overexpression group was upregulated, and compared with the HIF-1α overexpression group, the expression of HIF-1α in the HIF-1α + miR-199a mimics group was downregulated (P<0.05, Figure 5A). At the same time, the results of MTT and a transwell assay showed that, compared with the control group, DMED promoted the proliferation of breast cancer cells. Similarly, the overexpression of HIF-1α promoted the proliferation of breast cancer cells. Compared with the HIF-1α overexpression group, the cell activity of HIF-1α + miR-199a mimics group was downregulated (P<0.05, Figure 5B,5C). Subsequently, the results of an apoptosis assay showed that DMED inhibited the apoptosis of breast cancer cells, and HIF-1α also inhibited the apoptosis of breast cancer cells. Compared with the vector group, on the other hand, and compared with the HIF-1α overexpression group, apoptosis increased in the HIF-1α + miR-199a mimics group (P<0.05, Figure 5D,5E). These results suggest that DMED regulates the miR-199a/HIF-1α axis to promote the proliferation of breast cancer cells and inhibit apoptosis, thus promoting the progression of breast cancer.
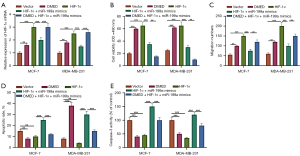
Discussion
In recent years, great progress has been made in the clinical treatment of breast cancer, but the incidence of, and mortality from, breast cancer are still on the rise (23). Therefore, it is of great significance to find effective and safe anticancer drugs. In addition, the study of the molecular mechanisms of breast cancer activity and apoptosis can provide a new basis for the treatment of breast cancer (24). It is of great significance to explore the molecular mechanism of drugs in the treatment of breast cancer.
In recent years studies have shown that DMED plays an important role in cell apoptosis and activity. Li et al. confirmed that DMED can significantly inhibit the loss of pulmonary microvascular endothelial cell (PMVEC) activity and apoptosis induced by IR serum (25). In addition, Sun et al. have confirmed that DMED can protect glial cells by reducing cell death (26). On the other hand, DMED is involved in the progression of cancer. Studies have shown that DEX can reduce the incidence and intensity of delirium after pneumonectomy in elderly patients with lung cancer. At the same time, DMED can provide effective cardio-brain protection in colorectal cancer surgery (27). Recent animal data suggest that DMED has a potential role in promoting cancer recurrence and metastasis during the perioperative period, especially after breast surgery (28). Other studies have shown that DMED has a protective effect on disease by regulating miRNA. Studies by Bao et al. showed that DMED may play an anti-inflammatory effect in LPS-stimulated BV2 cells by upregulating miR-340, and DMED may be a potential drug for the treatment of neuroinflammation (29). Similarly, miRNA-151-3p enhanced the neuroprotective effect of DMED on Aβ by targeting DAPK-1 and TP53 (30). In esophageal cancer, DMED inhibits the progression of esophageal cancer through the miR-143-3p/EPS8 axis (10). In addition, DMED upregulates miRNA-185 by inhibiting the SOX9/Wnt/β-catenin signaling pathway to inhibit ovarian cancer growth (31). In this study, we found that DMED can downregulate the expression of miR-199a in breast cancer MCF-7 and MDA-MB-231 cells. Further experiments showed that DMED promoted the activity of breast cancer cells and inhibited apoptosis through the miR-199a/HIF-1α axis.
MiR-199a plays an important role in regulating cell survival, apoptosis, and tumor cell metastasis. For example, miR-199a can inhibit the activity of thyroid cancer cells and promote apoptosis (32). MiRNA is associated with breast cancer cell activity and apoptosis. For example, miRNA-106a promotes breast cancer cell proliferation, clone formation, migration, and invasion by inhibiting apoptosis (33). In addition, studies by Xu et al. showed that miR-199a can inhibit the activity of breast cancer (34). MiR-199a is regulated by drugs. Guo et al. confirmed that quercetin downregulates the viability of miR-199a Sertoli cells and inhibits cardiomyocyte apoptosis (35). Similarly, propofol induces apoptosis in hepatocellular carcinoma cells by upregulating the expression of miRNA-199a (36). On the other hand, miR-199a can play a role in cancer or breast cancer by targeting mRNA, and studies by Ma et al. confirmed that miR-199a inhibits the progression of papillary thyroid cancer by targeting SNAI1 (37). In breast cancer, miR-199a regulates MRP1 to inhibit drug resistance in breast cancer cells (16). In breast cancer, miR-29b-3p promotes the activity of breast cancer cells by downregulating TRAF3 (38). HIF-1α is a transcriptional regulatory factor that exists widely in mammalian and human cells. Studies have confirmed that HIF-1α is highly expressed in many human tumors, which is closely related to tumor growth, reproduction, invasion and metastasis, neovascularization, apoptosis (39). On the other hand, Cao et al. have confirmed that HIF-1α can promote the activity of breast cancer cells (19). Some studies have also confirmed that HIF-1α is regulated by miRNA in cancer. Wu et al. confirmed that miR-49 inhibits angiogenesis in breast cancer by targeting HIF-1α (40). Previous studies confirmed that miR-199a protects PC12 cells from oxidative damage by regulating HIF-1α (17). In this study, it was found that downregulation of miR-199a expression could upregulate the expression of HIF-1α, and DMED could upregulate the expression of HIF-1α. Further experiments confirmed that HIF-1α was the direct target of miR-199a and could partially reverse the inhibitory effect of miR-199a on apoptosis of breast cancer cells. Therefore, we speculate that in breast cancer, miR-199a can target HIF-1α and inhibit the progression of breast cancer.
Conclusions
This study found that DMED could inhibit the progression of MCF-7 and MDA-MB-231 cells by regulating the miR-199a/HIF-1α axis. However, this study has shortcomings. Animal experiments need to further explore the role of DMED regulating miR-199a in the activity of breast cancer cells. In addition, it needs to be assessed whether DMED can regulate other miRNAs to play a role in the activity of breast cancer cells. In short, the findings of this study can provide guidance for the treatment of DMED and molecular targeted therapy in breast cancer.
Acknowledgments
Funding: None.
Footnote
Reporting Checklist: The authors have completed the MDAR reporting checklist. Available at https://dx.doi.org/10.21037/tcr-21-1937
Data Sharing Statement: Available at https://dx.doi.org/10.21037/tcr-21-1937
Conflicts of Interest: Both authors have completed the ICMJE uniform disclosure form (available at https://dx.doi.org/10.21037/tcr-21-1937). The authors have no conflicts of interest to declare.
Ethical Statement: The authors are accountable for all aspects of the work in ensuring that questions related to the accuracy or integrity of any part of the work are appropriately investigated and resolved.
Open Access Statement: This is an Open Access article distributed in accordance with the Creative Commons Attribution-NonCommercial-NoDerivs 4.0 International License (CC BY-NC-ND 4.0), which permits the non-commercial replication and distribution of the article with the strict proviso that no changes or edits are made and the original work is properly cited (including links to both the formal publication through the relevant DOI and the license). See: https://creativecommons.org/licenses/by-nc-nd/4.0/.
References
- Coker-Gurkan A, Can E, Sahin S, et al. Atiprimod triggered apoptotic cell death via acting on PERK/eIF2α/ATF4/CHOP and STAT3/NF-KB axis in MDA-MB-231 and MDA-MB-468 breast cancer cells. Mol Biol Rep 2021;48:5233-47. [Crossref] [PubMed]
- Depboylu B. Treatment and patient related quality of life issues in elderly and very elderly breast cancer patients. Transl Cancer Res 2020;9:S146-53. [Crossref]
- Pellicciaro M, Materazzo M, Buonomo C, et al. Feasibility and oncological safety of axillary reverse mapping in patients with locally advanced breast cancer and partial response after neoadjuvant chemotherapy. In Vivo 2021;35:2489-94. [Crossref] [PubMed]
- Zhou Y, Yue Y, Fan S, et al. Advances in pathophysiology of triple-negative breast cancer: the potential of lncRNAs for clinical diagnosis, treatment, and prognostic monitoring. Mol Biotechnol 2021; Epub ahead of print. [Crossref] [PubMed]
- Ezzati M, Yousefi B, Velaei K, et al. A review on anti-cancer properties of Quercetin in breast cancer. Life Sci 2020;248:117463. [Crossref] [PubMed]
- Qiu J, Zhang T, Zhu X, et al. Hyperoside induces breast cancer cells apoptosis via ROS-mediated NF-κB signaling pathway. Int J Mol Sci 2019;21:131. [Crossref] [PubMed]
- Keating GM. Dexmedetomidine: A Review of Its Use for Sedation in the Intensive Care Setting. Drugs 2015;75:1119-30. [Crossref] [PubMed]
- Zhai M, Liu C, Li Y, et al. Dexmedetomidine inhibits neuronal apoptosis by inducing Sigma-1 receptor signaling in cerebral ischemia-reperfusion injury. Aging (Albany NY) 2019;11:9556-68. [Crossref] [PubMed]
- Lavon H, Matzner P, Benbenishty A, et al. Dexmedetomidine promotes metastasis in rodent models of breast, lung, and colon cancers. Br J Anaesth 2018;120:188-96. [Crossref] [PubMed]
- Zhang P, He H, Bai Y, et al. Dexmedetomidine suppresses the progression of esophageal cancer via miR-143-3p/epidermal growth factor receptor pathway substrate 8 axis. Anticancer Drugs 2020;31:693-701. [Crossref] [PubMed]
- Chi M, Shi X, Huo X, et al. Dexmedetomidine promotes breast cancer cell migration through Rab11-mediated secretion of exosomal TMPRSS2. Ann Transl Med 2020;8:531. [Crossref] [PubMed]
- Xia M, Ji NN, Duan ML, et al. Dexmedetomidine regulate the malignancy of breast cancer cells by activating α2-adrenoceptor/ERK signaling pathway. Eur Rev Med Pharmacol Sci 2016;20:3500-6. [PubMed]
- Debnath T, Deb Nath NC, Kim EK, et al. Role of phytochemicals in the modulation of miRNA expression in cancer. Food Funct 2017;8:3432-42. [Crossref] [PubMed]
- Fridrichova I, Zmetakova I. MicroRNAs Contribute to Breast Cancer Invasiveness. Cells 2019;8:1361. [Crossref] [PubMed]
- Hu D, Li M, Su J, et al. Dual-targeting of miR-124-3p and ABCC4 promotes sensitivity to Adriamycin in breast cancer cells. Genet Test Mol Biomarkers 2019;23:156-65. [Crossref] [PubMed]
- Chang L, Hu Z, Zhou Z, et al. Linc00518 contributes to multidrug resistance through regulating the miR-199a/MRP1 axis in breast cancer. Cell Physiol Biochem 2018;48:16-28. [Crossref] [PubMed]
- Wu L, Xi Y, Kong Q. Dexmedetomidine protects PC12 cells from oxidative damage through regulation of miR-199a/HIF-1α. Artif Cells Nanomed Biotechnol 2020;48:506-14. [Crossref] [PubMed]
- Zhu Y, Zhao H, Zhang W, et al. Dexmedetomidine attenuates neuronal injury induced by cerebral ischemia-reperfusion by regulating miR-199a. Mol Med Rep 2021;24:574. [Crossref] [PubMed]
- Cao L, Wang M, Dong Y, et al. Circular RNA circRNF20 promotes breast cancer tumorigenesis and Warburg effect through miR-487a/HIF-1α/HK2. Cell Death Dis 2020;11:145. [Crossref] [PubMed]
- Zhao W, Geng D, Li S, et al. LncRNA HOTAIR influences cell growth, migration, invasion, and apoptosis via the miR-20a-5p/HMGA2 axis in breast cancer. Cancer Med 2018;7:842-55. [Crossref] [PubMed]
- Shi XY, Wang H, Wang W, et al. MiR-98-5p regulates proliferation and metastasis of MCF-7 breast cancer cells by targeting Gab2. Eur Rev Med Pharmacol Sci 2020;24:10914. [PubMed]
- Choudhary GS, Al-Harbi S, Almasan A. Caspase-3 activation is a critical determinant of genotoxic stress-induced apoptosis. Methods Mol Biol 2015;1219:1-9. [Crossref] [PubMed]
- Sopik V. International variation in breast cancer incidence and mortality in young women. Breast Cancer Res Treat 2021;186:497-507. [Crossref] [PubMed]
- González-González A, Mediavilla MD, Sánchez-Barceló EJ. Melatonin: a molecule for reducing breast cancer risk. Molecules 2018;23:336. [Crossref] [PubMed]
- Li J, Chen Q, He X, et al. Dexmedetomidine attenuates lung apoptosis induced by renal ischemia-reperfusion injury through α2AR/PI3K/Akt pathway. J Transl Med 2018;16:78. [Crossref] [PubMed]
- Sun YB, Zhao H, Mu DL, et al. Dexmedetomidine inhibits astrocyte pyroptosis and subsequently protects the brain in in vitro and in vivo models of sepsis. Cell Death Dis 2019;10:167. [Crossref] [PubMed]
- Yi XL, Wang JT, Chu CQ, et al. Cardiocerebral protective effects of dexmedetomidine as anesthetic in colorectal cancer surgery. Eur Rev Med Pharmacol Sci 2018;22:3570-6. [PubMed]
- Nair AS, Saifuddin MS, Naik V, et al. Dexmedetomidine in cancer surgeries: Present status and consequences with its use. Indian J Cancer 2020;57:234-8. [Crossref] [PubMed]
- Bao Y, Zhu Y, He G, et al. Dexmedetomidine attenuates neuroinflammation in LPS-stimulated BV2 microglia cells through upregulation of miR-340. Drug Des Devel Ther 2019;13:3465-75. [Crossref] [PubMed]
- Guo Y, Wu Y, Li N, et al. Up-regulation of miRNA-151-3p enhanced the neuroprotective effect of dexmedetomidine against β-amyloid by targeting DAPK-1 and TP53. Exp Mol Pathol 2021;118:104587. [Crossref] [PubMed]
- Tian H, Hou L, Xiong Y, et al. Dexmedetomidine upregulates microRNA-185 to suppress ovarian cancer growth via inhibiting the SOX9/Wnt/β-catenin signaling pathway. Cell Cycle 2021;20:765-80. [Crossref] [PubMed]
- Wang D, Zheng Y. Silencing long non-coding RNAs nicotinamide nucleotide transhydrogenase antisense RNA 1 inhibited papillary thyroid cancer cell proliferation, migration and invasion and promoted apoptosis via targeting miR-199a-5p. Endocr J 2021;68:583-97. [Crossref] [PubMed]
- You F, Luan H, Sun D, et al. miRNA-106a promotes breast cancer cell proliferation, clonogenicity, migration, and invasion through inhibiting apoptosis and chemosensitivity. DNA Cell Biol 2019;38:198-207. [Crossref] [PubMed]
- Xu ST, Xu JH, Zheng ZR, et al. Long non-coding RNA ANRIL promotes carcinogenesis via sponging miR-199a in triple-negative breast cancer. Biomed Pharmacother 2017;96:14-21. [Crossref] [PubMed]
- Guo G, Gong L, Sun L, et al. Quercetin supports cell viability and inhibits apoptosis in cardiocytes by down-regulating miR-199a. Artif Cells Nanomed Biotechnol 2019;47:2909-16. [Crossref] [PubMed]
- Zhang J, Wu GQ, Zhang Y, et al. Propofol induces apoptosis of hepatocellular carcinoma cells by upregulation of microRNA-199a expression. Cell Biol Int 2013;37:227-32. [Crossref] [PubMed]
- Ma S, Jia W, Ni S. miR-199a-5p inhibits the progression of papillary thyroid carcinoma by targeting SNAI1. Biochem Biophys Res Commun 2018;497:181-6. [Crossref] [PubMed]
- Zhang B, Shetti D, Fan C, et al. miR-29b-3p promotes progression of MDA-MB-231 triple-negative breast cancer cells through downregulating TRAF3. Biol Res 2019;52:38. [Crossref] [PubMed]
- Wei TT, Lin YT, Tang SP, et al. Metabolic targeting of HIF-1α potentiates the therapeutic efficacy of oxaliplatin in colorectal cancer. Oncogene 2020;39:414-27. [Crossref] [PubMed]
- Wu Z, Cai X, Huang C, et al. miR-497 suppresses angiogenesis in breast carcinoma by targeting HIF-1α. Oncol Rep 2016;35:1696-702. [Crossref] [PubMed]
(English Language Editor: B. Meiser)