Particle therapy for pancreatic cancer
Introduction
In 2015, approximately 22,000 patients in the United States will be diagnosed with pancreas cancer without evidence of distant metastasis (1). Half of these patients will be categorized as having unresectable disease and the other half as resectable (1). Unfortunately, the long-term survival expectation for the former group is less than 5% and, for the latter group, approximately 20% (2). Theoretically, since local progression is a problem for these patients, both groups should benefit from radiotherapy. Unfortunately, for patients with unresectable disease, level one evidence from the LAP-07 trial suggests that conventionally delivered X-ray-based radiotherapy may fail to offer an improvement in survival (3). For patients receiving surgery and postoperative radiotherapy, level one evidence from the ESPAC trial likewise argues that radiotherapy does not offer improved survival and may in fact be associated with a nominal, albeit statistically insignificant, survival decrement (4,5). A consequence of these recent publications is that radiotherapy, in many centers, is not being offered to patients with pancreatic cancer.
While valid criticisms of the methodology surrounding the ESPAC trial exist (6), it is understandable why conventional X-ray-based radiotherapy may be of limited use in the treatment of upper-abdominal malignancies such as pancreas cancer. This limitation is primarily due to the fact that the pancreas lies in intimate proximity to exquisitely radiosensitive normal tissues such as the duodenum, stomach, jejunum, liver, and kidneys.
Particle therapy, utilizing protons or carbon ions, has been explored in the treatment of pancreas cancer patients. Dosimetric studies indicate that particle therapy has the potential to improve the therapeutic index by delivering significant dose to tumor-related targets while simultaneously minimizing exposure to critical normal tissues. Preliminary clinical data appear to validate these findings.
Physics of particle therapy
Charged particles such as protons or carbon ions travel a finite distance into tissue as determined by their energy. Unlike X-rays, which deliver dose along their entire path through tissue, charged particles release most of their energy in a tightly defined region called the “Bragg peak.” By utilizing a range of energies, it is possible to create a dose distribution at the tumor depth, which is referred to as the “spread-out Bragg peak” (Figure 1). As such, proton therapy allows for the modulation of radiation dose intensity along the beam path, allowing for a relative increase in dose to the tumor target relative to the dose to normal tissues. This concept is visually demonstrated in Figure 2.
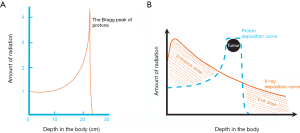
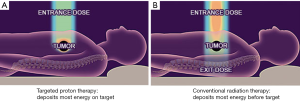
Physical and radiobiological differences between proton and carbon ion therapy
While both protons and carbon ions share physical characteristics, such as the Bragg peak, they differ with respect to some physical beam characteristics. Their radiobiological properties also differ. In terms of physical dose distribution, carbon ions have a smaller (sharper) lateral penumbra compared with protons. While this would suggest dosimetric superiority of carbon ions over protons, carbon ion dose distributions are complicated by a “fragmentation tail”—caused by the creation of lower atomic weight particles—which results in the delivery of dose beyond the Bragg peak. The presence of the fragmentation tail also may increase uncertainty about the relative biologic effect (RBE) of carbon ions in the clinical setting—particularly at the distal edge of the spread out Bragg peak—although similar uncertainties are recognized to a lesser degree with protons in that region.
Radiobiologically, the most critical difference between proton and carbon ion beams concerns their linear energy transfer (LET) properties. Specifically, proton beams are categorized as having low LET (similar to X-rays) while carbon ion beams are characterized as having high LET. Although the radiobiology associated with low LET radiation is fairly well understood, less clinical data are available to characterize the RBEs of high LET radiation. For example, with protons, the RBE compared with megavoltage X-rays is generally accepted to be 1.1. In other words, for equivalent physical doses delivered, proton beams offer a 10% higher biologic effect compared to X-rays. The RBE for carbon ions is less well-defined. Studies have indicated it to be in a range between 2.5 and 3.5. The RBE also may differ between tumor and normal tissues. Other things being equal, it is argued that carbon ions will have a greater effect on hypoxic tumors compared with protons or X-rays. Similarly, it may be argued that carbon ions would be associated with a greater risk of damage to exposed normal tissues (7).
Dosimetric studies suggesting that particle therapy may improve the therapeutic index
The eight studies in the current radiotherapy literature discussed below indicate that particle therapy may improve the therapeutic index for patients receiving radiotherapy for a pancreatic malignancy. While all of these studies are based on proton dose distributions, it is reasonable to believe that these dosimetric benefits are also applicable to the treatment of patients with carbon ions.
Hsiung-Stripp et al. (8) reported a dosimetric study using protons to treat unresectable pancreas cancers. Compared with X-ray-based plans, the protons demonstrated significantly reduced doses to the spinal cord, left kidney, right kidney, and liver.
Kozak et al. (9) compared protons with intensity-modulated radiation therapy (IMRT) using a hypofractionated regimen as preoperative therapy for 9 patients with resectable pancreas cancer. In this series, protons demonstrated a significant reduction in dose to the liver, kidneys, and small bowel, particularly in the low-dose regions.
Bouchard et al. (10) compared proton plans delivering 72 Gy (RBE) to unresectable pancreatic tumors with similarly effective 3-dimensional conformal radiotherapy (3DCRT) and IMRT plans. For patients with anteriorly located small bowel, the study concluded that protons were superior to the X-ray-based treatment approaches.
Nichols et al. (11) generated passively scattered proton plans for 8 patients previously planned and treated with IMRT at an outside institution. The team generating the proton plans did not have access to the dose distributions achieved by the IMRT plans. All patients were planned to receive 50.4 Gy (RBE). The proton plans demonstrated significantly reduced normal-tissue exposure compared to the IMRT plans with respect to median small bowel V 20 Gy (RBE), median gastric V 20 Gy (RBE), and median right kidney V 18 Gy (RBE). A representative comparative dose distribution is shown in Figure 3.
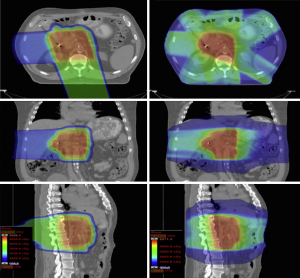
Lee et al. (12) compared proton plans in the neoadjuvant setting covering gross disease plus high-risk regional lymph nodes with plans treating gross disease alone. The investigation showed a minimal increase in normal tissue exposure despite an almost twofold increase in the planning target volume (PTV). The authors argued that treating a similar increase in target volume with X-rays would be more difficult due to potential normal-tissue exposure.
Ding et al. (13) compared proton plans with 3DCRT, 5-field IMRT, and 2-arc volumetric modulated radiation therapy plans. Proton plans demonstrated lower doses to the kidneys, stomach, liver, and bowel.
Thompson et al. (14) compared passively scattered and pencil-beam proton plans with IMRT plans for 13 patients with unresectable pancreatic head cancers. Although proton plans showed decreased gastric duodenal and small bowel dose in the low-dose regions compared to IMRT, the IMRT plans were superior with regard to dose distribution in the mid- to high-dose regions.
Ling et al. (15) evaluated radiation dose distributions for ten patients with pancreatic head adenocarcinoma. Planning volumes were created using the guidelines of the Radiation Therapy Oncology Group 0848 protocol. Proton, IMRT, and 3DCRT plans were evaluated. The proton plans demonstrated reduced mean liver dose and mean kidney dose compared to both the IMRT and 3DCRT plans.
Clinical outcome data for pancreas cancer patients treated with particle therapy
Numerous studies in the medical literature suggest that dosimetric improvements with particle therapy have translated into reduced radiotherapy toxicity rates, improved tumor control, or both. With regard to tumor control, although series are relatively small, the most impressive data are associated with the use of carbon ion therapy. These results are arguably consistent with the hypothesis that pancreatic tumors are intrinsically hypoxic and as such would be more responsive to treatment with high LET radiations such as carbon ions.
Proton studies
Hong et al. (16), building on the work of two prior studies (17,18), published the results of a phase I/II study of preoperative short-course chemoradiation with proton therapy and capecitabine followed by early surgery for resectable pancreatic ductal adenocarcinoma. Patients received 25 Gy (RBE) in 5 fractions over 2 weeks. A total of 50 patients were enrolled, 35 of whom were treated in phase 2 of the study. There were no grade 4 or 5 toxicities. Of the 35 patients, 2 experienced a grade 3 toxicity event. Of 48 patients eligible for analysis, 37 underwent pancreaticoduodenectomy. Of these patients, 81% had positive lymph nodes at surgery. The local-regional failure rate was 16.2% and distant recurrences occurred in 72.9%. The median overall survival was 17 months.
Terashima et al. (19) from the Hyogo Ion Beam Center in Japan published the results of a phase I/II study of aggressive chemoradiation for patients with locally advanced pancreatic cancer. All patients received gemcitabine at 800 mg/m2 weekly for 3 weeks with concurrent proton therapy. Most patients received a dose of 67.5 Gy (RBE) in 25 fractions. The 1-year freedom from local progression, progression-free survival, and overall survival rates were 81.7%, 64.3%, and 76.8% respectively. With a median follow-up of 12.5 months, 10% of patients experienced grade 3 or greater late gastric ulcer and/or hemorrhage. Takatori et al. (20) subsequently published results of post-treatment endoscopic examinations in 91 patients treated with this regimen. Overall, 45 (49.4%) demonstrated radiation-induced ulcers in the stomach and duodenum. While this report suggested that proton therapy might be associated with a high rate of gastrointestinal toxicity, a subsequent commentary (21) suggested that the toxicity was related to the aggressive treatment regimen offered rather than any problem intrinsically related to the use of proton therapy.
Nichols et al. (22) and published a report on the outcomes of 22 patients treated with proton therapy and concomitant capecitabine (1,000 mg by mouth twice daily) for resected (n=5), marginally resectable (n=5), and unresectable/inoperable (n=12) biopsy-proven pancreatic and ampullary adenocarcinoma. Doses ranged from 50.4 to 59.4 Gy (RBE). No patient demonstrated any grade 3 toxicity during treatment or follow-up. Three patients experienced grade 2 gastrointestinal toxicity, all of whom were treated early in the series with fields that included anterior and left lateral components. When field designs were modified to deliver the majority of the dose through a posterior field with a lightly weighted right-lateral field, grade 2 gastrointestinal toxicity was eliminated.
Sachsman et al. (23) reported the outcomes of a phase II clinical trial for patients with unresectable pancreatic cancer. Eleven patients received 59.4 Gy (RBE) at 1.8 gray (RBE) per fraction with concomitant oral capecitabine. The 2-year overall survival rate was 31%. The median survival rate was 18.4 months. The 2-year freedom from local progression rate was 69%. No patient experienced grade 2 or higher gastrointestinal toxicity. Four patients demonstrated an adequate radiographic response to radiation therapy justifying surgical exploration.
Carbon ion studies
In 2004, Ito et al. (24) reported on four patients treated with carbon ion therapy to a dose of 48 Gy equivalent at the Heavy Ion Medical Accelerator (Chiba, Japan). Treatment was delivered to the pancreatic area including the lymph nodes and nerve plexus. Severe cholangitis was observed in one patient, and there were no other reported complications or disorders caused by radiotherapy.
In 2010, Okada et al. (25) reported the results of an experience at the National Institute of Radiological Science (Chiba Japan). Twenty-two patients with resectable disease were enrolled in a trial using preoperative carbon ion radiotherapy. The local control rate was 100% at 1 year and 87% at 2 years. For the patients undergoing surgery, the 2-year survival rate was 36%. A trial was also initiated for patients with locally advanced pancreas cancer, on which 31 patients were enrolled. Doses were escalated from 38.4 Gy equivalent to 48.0 Gy (RBE) delivered in 12 fractions over 3 weeks. The local control rate at 1 year was 81% and the 1-year survival rate was 44%. The investigators reported acceptable toxicity in the treated patients. Although no specific data were provided in the publication, the authors suggested that carbon ion therapy allowed for the concomitant delivery of full-dose gemcitabine without a significant increase in toxicity.
More recently, Shinoto et al. (26) reported the results of a phase I trial of preoperative short-course carbon ion radiotherapy for patients with resectable pancreas cancer. Doses were escalated from 30 to 36.8 Gy (RBE) in 8 fractions over 2 weeks. Surgery was to be performed 2 to 4 weeks after the completion of carbon ion radiotherapy. In total, 26 patients were enrolled. Twenty-one of the 26 underwent surgery. No patient experienced a local recurrence. The 5-year survival rate for those who underwent surgery was 52%.
In 2013, Combs et al. (27) announced the opening of a phase 1 study evaluating carbon ion radiotherapy for patients with locally advanced pancreatic cancer (Phoenix-01). Patients will receive escalating radiotherapy doses from 40 Gy (RBE) up to 54 Gy (RBE) at 3 Gy (RBE) per fraction. All patients will receive weekly chemotherapy with gemcitabine at 300 mg/m2.
Durante et al. (28) recently published a sophisticated meta-analysis comparing particle therapy and X-ray-based therapy combined with chemotherapy for patients with locally advanced pancreas cancer. Based on the available data, the authors concluded that particle therapy was likely to significantly improve the 1-year overall survival expectation for this patient population.
Conclusions
Dosimetric and clinical data, evaluating either protons or carbon ions, suggest that particle therapy has the potential to improve the therapeutic index for pancreatic cancer patients treated with radiotherapy. While distant metastasis represents a common mechanism for treatment failure, many patients suffers morbidity and mortality from local and regional progression of disease. Given the recognized dosimetric shortcomings of X-ray-based therapies in the upper abdomen as well as the recognition that at least half of patients with nonmetastatic disease are inoperable, and that operable patients undergoing surgery have a high risk of local failure, it is reasonable to expect that particle therapy will play an important role in the treatment of this disease.
Acknowledgments
Funding: None.
Footnote
Provenance and Peer Review: This article was commissioned by the editorial office, Translational Cancer Research for the series “Pancreatic Cancer”. The article has undergone external peer review.
Conflicts of Interest: The author has completed the ICMJE uniform disclosure form (available at http://dx.doi.org/10.3978/j.issn.2218-676X.2015.11.02). The series “Pancreatic Cancer” was commissioned by the editorial office without any funding or sponsorship. RCN served as the unpaid Guest Editor of the series. The author has no other conflicts of interest to declare.
Ethical Statement: The author is accountable for all aspects of the work in ensuring that questions related to the accuracy or integrity of any part of the work are appropriately investigated and resolved.
Open Access Statement: This is an Open Access article distributed in accordance with the Creative Commons Attribution-NonCommercial-NoDerivs 4.0 International License (CC BY-NC-ND 4.0), which permits the non-commercial replication and distribution of the article with the strict proviso that no changes or edits are made and the original work is properly cited (including links to both the formal publication through the relevant DOI and the license). See: https://creativecommons.org/licenses/by-nc-nd/4.0/.
References
- National Cancer Institute. Pancreatic Cancer Treatment –for health professionals (PDQ®): Incidence and Mortality. Updated: July 10, 2015. Available online: http://www.cancer.gov/types/pancreatic/hp/pancreatic-treatment-pdq#link/_7_toc
- National Cancer Institute. Pancreatic Cancer Treatment –for health professionals (PDQ®): Prognosis and Survival. Updated: July 10, 2015. Available online: http://www.cancer.gov/types/pancreatic/hp/pancreatic-treatment-pdq#link/_49_toc
- Hammel P, Huguet F, Van Laethem JL, et al. Comparison of chemoradiotherapy (CRT) and chemotherapy (CT) in patients with a locally advanced pancreatic cancer (LAPC) controlled after 4 months of gemcitabine with or without erlotinib: Final results of the international phase III LAP 07 study. J Clin Oncol 2013;31:abstr LBA4003.
- Neoptolemos JP, Dunn JA, Stocken DD, et al. Adjuvant chemoradiotherapy and chemotherapy in resectable pancreatic cancer: a randomised controlled trial. Lancet 2001;358:1576-85. [PubMed]
- Neoptolemos JP, Stocken DD, Friess H, et al. A randomized trial of chemoradiotherapy and chemotherapy after resection of pancreatic cancer. N Engl J Med 2004;350:1200-10. [PubMed]
- Abrams RA, Lillemoe KD, Piantadosi S. Continuing controversy over adjuvant therapy of pancreatic cancer. Lancet 2001;358:1565-6. [PubMed]
- Suit H, DeLaney T, Goldberg S, et al. Proton vs carbon ion beams in the definitive radiation treatment of cancer patients. Radiother Oncol 2010;95:3-22. [PubMed]
- Hsiung-Stripp DC, McDonough J, Masters HM, et al. Comparative treatment planning between proton and X-ray therapy in pancreatic cancer. Med Dosim 2001;26:255-9. [PubMed]
- Kozak KR, Kachnic LA, Adams J, et al. Dosimetric feasibility of hypofractionated proton radiotherapy for neoadjuvant pancreatic cancer treatment. Int J Radiat Oncol Biol Phys 2007;68:1557-66. [PubMed]
- Bouchard M, Amos RA, Briere TM, et al. Dose escalation with proton or photon radiation treatment for pancreatic cancer. Radiother Oncol 2009;92:238-43. [PubMed]
- Nichols RC Jr, Huh SN, Prado KL, et al. Protons offer reduced normal-tissue exposure for patients receiving postoperative radiotherapy for resected pancreatic head cancer. Int J Radiat Oncol Biol Phys 2012;83:158-63. [PubMed]
- Lee RY, Nichols RC Jr, Huh SN, et al. Proton therapy may allow for comprehensive elective nodal coverage for patients receiving neoadjuvant radiotherapy for localized pancreatic head cancers. J Gastrointest Oncol 2013;4:374-9. [PubMed]
- Ding X, Dionisi F, Tang S, et al. A comprehensive dosimetric study of pancreatic cancer treatment using three-dimensional conformal radiation therapy (3DCRT), intensity-modulated radiation therapy (IMRT), volumetric-modulated radiation therapy (VMAT), and passive-scattering and modulated-scanning proton therapy (PT). Med Dosim 2014;39:139-45. [PubMed]
- Thompson RF, Mayekar SU, Zhai H, et al. A dosimetric comparison of proton and photon therapy in unresectable cancers of the head of pancreas. Med Phys 2014;41:081711 [PubMed]
- Ling TC, Slater JM, Mifflin R, et al. Evaluation of normal tissue exposure in patients receiving radiotherapy for pancreatic cancer based on RTOG 0848. J Gastrointest Oncol 2015;6:108-14. [PubMed]
- Hong TS, Ryan DP, Blaszkowsky LS, et al. Phase I study of preoperative short-course chemoradiation with proton beam therapy and capecitabine for resectable pancreatic ductal adenocarcinoma of the head. Int J Radiat Oncol Biol Phys 2011;79:151-7. [PubMed]
- Wo JY, Mamon HJ, Ferrone CR, et al. Phase I study of neoadjuvant accelerated short course radiation therapy with photons and capecitabine for resectable pancreatic cancer. Radiother Oncol 2014;110:160-4. [PubMed]
- Hong TS, Ryan DP, Borger DR, et al. A phase 1/2 and biomarker study of preoperative short course chemoradiation with proton beam therapy and capecitabine followed by early surgery for resectable pancreatic ductal adenocarcinoma. Int J Radiat Oncol Biol Phys 2014;89:830-8. [PubMed]
- Terashima K, Demizu Y, Hashimoto N, et al. A phase I/II study of gemcitabine-concurrent proton radiotherapy for locally advanced pancreatic cancer without distant metastasis. Radiother Oncol 2012;103:25-31. [PubMed]
- Takatori K, Terashima K, Yoshida R, et al. Upper gastrointestinal complications associated with gemcitabine-concurrent proton radiotherapy for inoperable pancreatic cancer. J Gastroenterol 2014;49:1074-80. [PubMed]
- Nichols RC Jr, Hoppe BS RE, Takatori K, Terashima K, Yoshida R, Horai A, Satake S, Ose T, Kitajima N, Kinoshita Y, Demizu Y, Fuwa N. Upper gastrointestinal complications associated with gemcitabine-concurrent proton radiotherapy for inoperable pancreatic cancer. J Gastroenterol. 2013; (E-pub only). J Gastrointest Oncol 2013;4:E33-4. [PubMed]
- Nichols RC Jr, George TJ, Zaiden RA Jr, et al. Proton therapy with concomitant capecitabine for pancreatic and ampullary cancers is associated with a low incidence of gastrointestinal toxicity. Acta Oncol 2013;52:498-505. [PubMed]
- Sachsman S, Nichols RC, Morris CG, et al. Proton Therapy and Concomitant Capecitabine for Non-Metastatic Unresectable Pancreatic Adenocarcinoma. Int J Particle Ther 2014;1:692-701.
- Ito H, Kimura F, Shimizu H, et al. Surgical resection for pancreatic cancer combined with preoperative carbon-ion beam irradiation. Gan To Kagaku Ryoho 2004;31:1879-81. [PubMed]
- Okada T, Kamada T, Tsuji H, et al. Carbon ion radiotherapy: clinical experiences at National Institute of Radiological Science (NIRS). J Radiat Res 2010;51:355-64. [PubMed]
- Shinoto M, Yamada S, Yasuda S, et al. Phase 1 trial of preoperative, short-course carbon-ion radiotherapy for patients with resectable pancreatic cancer. Cancer 2013;119:45-51. [PubMed]
- Combs SE, Habermehl D, Kieser M, et al. Phase I study evaluating the treatment of patients with locally advanced pancreatic cancer with carbon ion radiotherapy: the PHOENIX-01 trial. BMC Cancer 2013;13:419. [PubMed]
- Durante M, Tommasino F, Yamada S. Modeling Combined Chemotherapy and Particle Therapy for Locally Advanced Pancreatic Cancer. Front Oncol 2015;5:145. [PubMed]