Particle therapy for central nervous system tumors in pediatric and adult patients
Introduction
Despite the efficacy of radiation therapy (RT) in the treatment of central nervous system (CNS) tumors, substantial concerns exist regarding adverse radiation effects. Concerns regarding long-term radiation effects, including soft tissue or bone deformities, vascular damage, endocrine deficits, heart or lung damage, progressive cognitive decline, or even secondary malignancies, are further heightened as survival rates increase.
The central tenants of radiotherapy are to deliver tumoricidal doses of radiation accurately to target volumes while minimizing unnecessary exposure of normal tissues. Historically, the overwhelming majority of radiation treatments have been delivered using photon-based techniques. Diagnostic imaging, treatment planning, and tailored dose delivery have all advanced dramatically in recent decades, but the physical limitations of photon interactions within the body may now preclude further sparing of normal tissues.
Particle therapy is most commonly delivered using either protons or carbon ions. Both share the physical advantages of particle therapy in that beyond the Bragg peak, exit dose is essentially eliminated. In comparison with photon-based therapies, including intensity modulated radiation therapy (IMRT) or stereotactic treatments, radiation dose to normal tissues will virtually always be less, especially in the low dose regions. While both protons and carbon ions spare normal tissues, there are distinct biological differences between these particles. The concept of relative biological effectiveness (RBE) describes the cell killing capacity of various forms of radiation in relation to photons, leading to the creation of the term Gy(RBE) as the unit used to describe the physical dose (in Gy) times the determined RBE value. Carbon ions have a much higher RBE than protons, which could negatively impact exposed normal tissues adjacent to or within the target volume, as would be the case with craniospinal (CSI) irradiation, for example. Therefore, experience using proton therapy is rapidly expanding in the treatment of pediatric tumors, but greater caution applies to the use of carbon ions in this population. However, the higher RBE may be advantageous for treatment of tumors considered to be resistant to conventional radiation. Indeed, for adults, there are numerous ongoing studies in this area. In this review, we will highlight the potential advantages of particle therapy when used for central nervous system tumors in both adults and children. We will present selected dosimetric studies, but focus on published clinical data supporting the use of particle treatments.
Particle therapy for pediatric brain tumors
Toxicities have been well-documented in long-term survivors of childhood cancers. The Childhood Cancer Survivor Study, funded by the National Cancer Institute, has systematically documented the long-term effects of treatment for childhood cancers in more than 14,000 survivors. Among these, over 1,800 survivors of CNS malignancies were included. In patients treated with radiotherapy, the cumulative incidence of secondary CNS malignancies was 7.1% at 25 years vs. 1% in patients not receiving radiation (1,2). Secondary malignancies include such tumors as meningiomas, thyroid tumors, and sarcomas, and these may contribute to the excess late mortality observed as compared with the general population (2). Therapeutic radiation exposure also contributed to endocrine, neurologic and neurocognitive deficits (3). Notably, a documented, dose-dependent relationship was also found between radiation and increased unemployment rates and decreased rates of marriage (4).
Medulloblastoma
Medulloblastomas represent one of the most common malignant CNS tumors in children. The high potential for subclinical dissemination throughout the brain and spinal column necessitates larger-field irradiation. In the United States, children over the age of 3 are typically treated with CSI, with dose selected based on set risk factors, including documented metastatic disease or residual tumor measuring >1.5 cm2. Following CSI, an additional boost is delivered to the tumor bed plus margin or to the entire posterior fossa. Although there are ongoing attempts to reduce radiotherapy doses and volumes for medulloblastoma treatment, omission of radiotherapy has been associated with increased rates of recurrence and decreased survival (5).
As exposure of normal tissues to exit dose is unavoidable using photon therapy, many practitioners view CSI as a clear indication for proton therapy (PT). Comparative treatment plans demonstrate the complete sparing of structures distal to the spinal field using PT (Figure 1A). The potential adverse effects of irradiating these distal tissues are only now being realized. A retrospective study of 4,122 5-year childhood-cancer survivors found that individuals receiving an average of 5 Gy to the heart had a 12.5 relative risk over the general population for developing cardiovascular disease (6). Although clinical studies examining long-term clinical outcomes in patients treated with CSI using particles have yet to be performed, there have been several dosimetric studies. Lee et al. compared dose volume histograms (DVHs) in a prototypical patient receiving CSI with different treatment modalities, and found that the percentage of the heart receiving at least 10 Gy(RBE) was 65% with photons, 10% with electrons and 0% with protons (7).
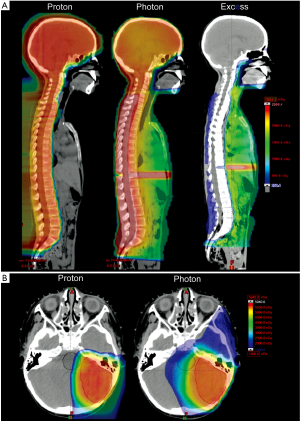
Several studies have shown that PT, when utilized for the boost treatment following CSI, can significantly reduce dose to non-target structures (Figure 1B) (8). Comparative DVH’s looking at the volume of cochlea receiving more than 20 Gy(RBE) during posterior fossa boost irradiation were 34% for PT, 87% for IMRT, and 89% for 3D-conformal radiotherapy (3D-CRT). Similarly, the volume of the hypothalamic-pituitary axis receiving more than 10 Gy(RBE) was 21% for protons, 81% for IMRT, and 91% for 3D-CRT. Dosimetric comparisons in another pediatric medulloblastoma case at MGH showed similar dose-sparing to the cochlea and pituitary when using proton therapy (9). Early clinical data from MD Anderson evaluating ototoxicity corroborates the clinical significance of these dosimetric studies (10). Nineteen patients with grossly intact hearing were evaluated 1 year following PT with only a single patient experiencing greater than grade 2 ototoxicity; more than half had no measurable hearing deficit. These clinical results compare favorably with ototoxicity after CSI using photons, including IMRT (11,12).
Given the large volumes irradiated with CSI and the high cure rates for medulloblastoma, secondary malignancies are a significant concern in long-term survivors. Using data from the International Commission on Radiologic Protection, Publication 60, to create a model for risk of cancer induction and normal tissue complication (13), two studies have shown the potential to reducing secondary malignancies using particle therapy. Miralbell et al. found PT was able to reduce the expected incidence of secondary malignancies by 8 to 15 fold compared with either IMRT or conventional photon therapy (14).
Similarly, a review of comparative treatment plans for five children with medulloblastoma found that the risk for secondary malignancy was lowest using intensity-modulated proton therapy (IMPT) (4%), followed by intensity-modulated electron therapy (IMET) (15%), conventional photon therapy (20%), and electron therapy (21%) (15). Investigators at MGH presented preliminary results suggesting a reduced incidence of secondary cancers in pediatric patients treated with protons at the Harvard Cyclotron, based upon a retrospective study compared with photon patients extracted from the SEER database (16).
Ependymomas
Radiation also plays a crucial role in the management of ependymomas, but with typically much more limited field sizes restricted to those areas at highest risk for disease recurrence (i.e., the tumor bed). Nonetheless, cognitive deficits remain a challenge. Merchant et al. analyzed data from 88 patients who received the standard treatment for ependymomas to create a model to predict IQ, finding that increased doses to the total brain and to supratentorial volumes were predictive of lower intelligence (17). MacDonald et al. have published data on the clinical outcomes of 17 patients treated with proton therapy, in addition to performing a dosimetric comparison of IMPT, passive-scatter proton therapy (PSPT) and IMRT (18). At a median of 26 months after radiation, local control, progression free survival, and overall survival were 86%, 80%, and 89%, respectively, comparable to traditional photon-based treatments but without any significant toxicity yet observed (19,20). In comparing dose distributions in a patient with an infratentorial ependymoma, advantages of proton therapy were clearly demonstrated. The dose received by 50% of the whole brain was <0.1 Gy(RBE) for IMPT, <0.1 Gy(RBE) for PSPT, and 2 Gy for IMRT; the temporal lobe received 2 Gy(RBE) using IMPT, 4 Gy(RBE) with PSPT, and 16 Gy from IMRT. According to Merchant’s model (17), these dose reductions seen in PSPT and IMPT for whole brain and temporal lobes suggest reduced adverse effects on IQ and reading ability, respectively. MacDonald’s analysis also showed that PT was able to reduce cochlea doses significantly [0.1 Gy(RBE) for IMPT, 2 Gy(RBE) for PSPT, 37 Gy for IMRT], indicating that PT would be expected to preserve hearing (21). Similarly, dose sparing to the hypothalamus observed with PT may potentially avoid life-long endocrine deficits.
A small percentage of pediatric ependymomas arise within the spine, where surgical resection is the standard of care, yielding long-term control rates of >84% after complete resection (22). The management of incompletely resected spinal ependymomas is less certain, perhaps resulting from the low incidence of spinal ependymomas leading to less available data. Several studies have reported excellent control with subtotal resection alone, thereby recommending deferring adjuvant RT in most cases to avoid toxicities associated with spinal radiation (22,23). Other studies, however, have reported significantly improved control rates with adjuvant RT (24-26).
PT may offer a potential solution for incompletely resected spinal ependymomas. In eight cases of spinal ependymoma treated with adjuvant proton therapy at MD Anderson, Amsbaugh et al. reported 100% rates of local control, progression-free survival and overall survival at mean follow-up of 26 months, and with no Grade 3 or higher side-effects thus far observed (27).
Craniopharyngiomas
Although craniopharyngiomas are histologically benign lesions of the sellar region, they have a high propensity for local recurrence. Attempting to avoid RT by obtaining a gross total resection, however, often results in significant surgical morbidity and mortality (28,29). Consequently, the standard treatment has typically been maximal safe resection followed by adjuvant RT. Neurocognitive and endocrine deficits are the most common sequelae of radiotherapy in this location (30).
Boehling et al. studied the dosimetric advantages of proton therapy, highlighting the potential for improving cognitive outcomes by sparing of the hippocampal formations with IMPT (31). Given that these tumors are known to undergo cystic changes during the course of radiotherapy, however, caution must be utilized with employing modalities such as IMPT (32).
Two studies have published clinical outcomes of pediatric craniopharyngiomas treated with PSPT. Luu et al. retrospectively analyzed 16 patients treated with fractionated PT at Loma Linda, 12 of which were undergoing salvage therapy for tumor recurrence (33). At a mean follow-up of 60.2 months, local control was achieved in 14 patients (93.3%). Toxicities included one patient with pan-hypopituitarism after repeat resection and PT, another suffered from a cerebrovascular accident but fully recovered, and a third developed a posterior fossa meningioma 59 months after PT. Although cognitive function was not specifically reported in this study, neurocognitive deficits associated with radiotherapy of craniopharyngiomas are expected to decrease with PT based on lower doses to the supratentorial brain (34). Fitzek et al. have also reported promising functional outcomes on pediatric craniopharyngiomas treated using combined photon and proton therapy (35). All five patients in this series finished high school and were leading independent lives; three (treated at age 8.2, 16.7 and 16.8) were attending or had completed college. Only one had learning difficulties, but these were comparable to his pre-radiotherapy status.
Germ cell tumors
Germ cell tumors can be subdivided histologically into germinomas or non-germinatous germ-cell tumors (NGGCT). Germinomas are more sensitive to radiotherapy and were historically treated with CSI with >90% durable response rate (36). However, due to considerable morbidity associated with CSI, whole-ventricle radiotherapy (WVRT) followed with a boost dose to the involved field has become the standard of treatment for localized, midline tumors. There is also the potential for reduction of radiation doses using pre-radiotherapy chemotherapy (37). In contrast to germinomas where disease control rates are high, NGGCTs carry a less favorable prognosis with recurrences and dissemination throughout the craniospinal axis being common. As such, a multimodality approach for NGGCTs is commonly employed with chemotherapy, CSI, and surgical resection all playing important roles (38).
A study from MGH has provided initial clinical data on the utility of proton therapy for treatment of germ cell tumors (39). Twenty-two patients, 13 with germinomas and 9 with NGGCT, were treated using 3D-CPT. At a median follow-up of 28 months, there was only one recurrence found in the peritoneum in a patient who did not have a complete response to chemotherapy. No new neurocognitive or auditory deficits were identified post-radiotherapy. Using a patient that received WVRT as a representative case to compare DVHs, proton therapy demonstrated dose-sparing: the left temporal lobe received 12.9 Gy(RBE) with IMPT, 20.5 Gy with IMRT, and 13.8 Gy with 3D-CPT. Although the lens dose using IMRT was low, no measurable lens dose was received using either 3D-CPT or IMPT, suggesting a further benefit of lowered risk of cataracts formation.
Gliomas
Pediatric gliomas comprise a clinically and histologically diverse group of tumors. Fortunately, the majority of pediatric gliomas are low-grade pilocytic astrocytomas which are typically managed with maximal safe surgical resection. If disease progression is documented post-operatively, chemotherapy is often utilized as a temporizing measure, thereby delaying radiotherapy in younger children. Still, RT is necessary for certain patients, including unresectable lesions or in cases of tumor progression after resection.
A study from Loma Linda has shown proton therapy to be an attractive option for treatment of pilocytic astrocytomas (40). Twenty-seven patients with low-grade astrocytomas were treated using fractionated proton therapy, with 25 of them being treated after sub-total resection. At mean follow-up of 39 months, 6 cases had failed locally [local control (LC) =78%], and 4 patients died from tumor progression [overall survival (OS) =85%]. For unresectable, centrally located astrocytomas (n=15), LC was achieved in 87%. With substantial dose-sparing to the cochlea, temporal lobe, pituitary, and contralateral optic nerve, the investigators found no significant short-term complications after proton therapy.
Optic pathway gliomas (OPGs) represent another area where PT could be particularly useful. The current trend is to utilize radiotherapy for older patients and chemotherapy for younger patients, again, in an attempt to delay radiotherapy and mitigate radiation-related toxicities (41,42). However, children treated with chemotherapy prior to radiotherapy may have worse visual outcomes compared to children treated primarily with radiotherapy (43). A study at Loma Linda demonstrated dosimetric advantage of PT in the treatment of seven pediatric OPGs by reducing doses to critical structures (44). PT reduced relative doses to the optic chiasm (by 11%), pituitary gland (by 13%), and bilateral temporal lobes (by 39%) compared to 3D-CRT. Additionally, PT was able to reduce the dose to the contralateral optic nerve by 47%. Accordingly, PT may make radiotherapy a more attractive option for younger OPG patients when radiation is necessary.
High-grade gliomas represent 6.5% of all childhood CNS neoplasms (45). In a study at Loma Linda consisting of 28 children with various brain tumors treated with proton therapy, 4 exhibited tumor progression (46). Two patients with high-grade (Grade 3 or 4) gliomas died after a mean of 13 months. Due to the poor control rates with both photon and PT for high-grade gliomas, future PT plans may use dose-escalation to attempt improved control rates.
Atypical tertatoid/rhabdoid tumors
Atypical teratoid/rhabdoid tumors (AT/RT) are estimated to represent 2-3% of primary pediatric CNS tumors. AT/RT is a relatively new histologic identity with an aggressive course and poor outcomes (47-49). Several studies have used aggressive chemotherapy regimens utilizing increased dose-intensity and intrathecal administration with promising response rates (50,51). Given that the incidence of AT/RTs is highest in patients under three, practitioners often attempt to avoid radiation in fear of potential toxicities. However, delaying the initiation of radiation may lead to poor outcomes. A study in Taiwan (52) involving 17 patients who were managed with primary surgery with subsequent radiotherapy demonstrated that patients with increased latency between surgery and radiation did not fare as well as those that received radiation earlier. In another study of 22 cases of AT/RT (53), there were only 2 long-term survivors, both of whom received radiotherapy as part of the primary treatment. Based on an extensive literature review on AT/RT, Squire et al. (54) suggests that radiation should be employed as part of initial therapy in select cases, and there is currently a multi-institutional protocol in place for AT/RT that includes risk-adapted RT in the initial therapy for selected children under three (55). With the current trend towards using radiotherapy in the primary treatment in younger and therefore more radiosensitive patients, limiting the radiation doses becomes even more essential.
Particle therapy for adult brain tumors
Adults receiving radiotherapy for CNS tumors generally face many of the same side-effects as does the pediatric population, such as visual deficits, ototoxicity, cerebrovascular accidents, neurocognitive impairment, and increased risk of secondary malignancies (56). Although many consider the adult CNS to be more radiation resistant than that of children, radiation-induced cognitive impairment is known to occur in up to 50-90% of adults within 6-months after radiation for brain tumors (57-59). Adults that receive radiotherapy for CNS tumors face significant effects on quality of life with the most commonly reported symptoms being fatigue, mood changes, and cognitive dysfunction (60,61). Therefore, reducing doses of radiation to the normal tissues in the adult brain should also be a priority.
Pituitary adenomas
Pituitary adenomas are benign tumors found in the sella turcica, three quarters of which present with functional symptoms resulting from hormone over-secretion. Radiotherapy offers the potential for cure even if the lesion is unresectable, and is typically used after medical and surgical therapies have failed. Moreover, medical therapy may require life-long treatment, and tumors may become refractory to medical management.
Two primary dose schedules are commonly used in radiotherapy for pituitary adenomas. Stereotactic radiosurgery (SRS) delivers a high-dose single radiation treatment (typically 15 to 20 Gy), whereas fractionated schedules deliver 45-54 Gy over 5-6 weeks. SRS may normalize hormone levels of functional adenomas faster than does conventional fractionated RT (62). However, the use of SRS may be limited in larger tumors located in close proximity to critical structures, such as the optic chiasm, because of the high fractional doses prescribed. Several institutions have started performing proton stereotactic radiosurgery (PSRS) to take advantage of the favorable dose-distribution properties to achieve decreased risk of toxicity.
MGH has studied PSRS in the treatment of GH- and ACTH-secreting tumors. Of 22 patients with residual GH-secreting tumors after trans-sphenoidal resection who were then treated using PSRS with a median dose of 20 Gy(RBE), a complete response (CR), defined as sustained (≥3 months) normalization of IGF-1, was seen in 59% of patients after a median of 42 months post-radiotherapy (63). In another study, 38 patients with Cushing’s disease or Nelson’s syndrome were treated with PSRS for persistence of symptoms and elevated cortisol levels following trans-sphenoidal resection (64). At median follow-up of 62 months, CR was achieved in all 5 cases of Nelson’s syndrome, and 52% (17/33) of Cushing’s disease cases, with median time to CR being 18 months after PSRS. In both studies, the CR rate and time to CR was comparable to previous SRS studies (65-73). There were no cases of visual disturbance, seizure activity, or other clinical signs of brain injury, but both studies showed slightly higher rates of hypopituitarism after PSRS when compared with other SRS studies.
Meningiomas
Meningiomas are the most common benign CNS tumors in adults and portend a generally favorable prognosis, with 90% classified as WHO Class I. Surgery is the mainstay of the therapy, but radiotherapy is used as adjuvant therapy in cases of partial resection, or with high-grade or recurrent lesions. Radiotherapy can also be utilized as definitive treatment for lesions in locations where resection is not possible. Long-term control rates with current radiotherapy techniques are >90% (74). With the expected long-term survival, improving functional status and limiting toxicities are objectives of treatment.
Given the proximity of skull base meningiomas to critical structures, particle therapy provides an opportunity to reduce toxicities. Wenkel et al. studied 46 patients with benign base-of-skull meningiomas treated with a combination of photon and proton therapy, reporting a recurrence-free rate of 100% and 88% at 5 and 10 years, respectively (75). Four patients in this series experienced ophthalmic toxicities. In retrospect, doses to the optic nerve in these four patients were found to have exceeded the threshold of 54 Gy(RBE) after the doses were recalculated following a calibration error. Patients that did not receive >54 Gy(RBE) to the optic nerve did not experience any ophthalmic toxicity. This highlights the need for high quality physics support given the increased complexity of particle therapy.
Noël et al. studied functional outcomes of 51 patients with skull base meningiomas treated with a combination of photons and proton therapy (76). Four-year LC and OS rates were 98% and 100% respectively. Although two patients (3.9%) suffered from Grade 3 side effects, 68.8% of eye-related symptoms improved after radiotherapy, and 67% of other miscellaneous symptoms improved, comparing favorably with photon studies reporting functional outcomes (77-80). Weber et al. from the Paul Scherrer Institute studied 39 cases treated only with protons, using a pencil-beam scanning (PBS) technique (81). At least 10 patients in this series had WHO Grade II/III meningiomas, and the average tumor volumes were larger than most other series. LC and OS at 5 years were 84.8% and 81.8%, respectively, for all histology types, and were 100% for benign histology. The 5-year Grade 3/4 toxicity-free survival was 84.5%. Those patients that experienced higher-grade toxicities were those who presented with large tumor volumes and/or with meningiomas of the optic tract. These results appear to support the use of particle therapy for meningiomas, especially for lesions in close proximity to critical structures.
Vestibular schwannoma
Vestibular schwannomas are benign intracranial tumors of the myelin-forming cells of the vestibulocochlear nerve. As many vestibular schwannomas are found incidentally on imaging studies, and only 43-46% of tumors show any growth (with an average rate of 1.2-1.9 mm per year), observation is a reasonable option for many patients (82). For tumors that require treatment, surgery and radiotherapy can both be used as first-line modalities. Surgery offers excellent control rates, but definitive radiotherapy is also a therapeutic option that offers excellent tumor control rates of greater than 90%. While vestibular schwannomas treated with radiotherapy may have a reported lower incidence of adverse effects, including hearing loss and facial nerve palsies, compared with microsurgery, direct comparison is difficult to make as tumors treated with microsurgery tend to be larger in volume (83).
Harsh et al. used a PSRS protocol prescribing 12 Gy(RBE) to the tumor and limiting brainstem dose to 12 Gy(RBE). They found LC rates of 94%, trigeminal and facial nerve preservation in 95.3%, and hearing preservation in 33.3% (84). Low rates of hearing preservation were thought to be due to an older patient population (mean age =67) and surgical resections prior to radiotherapy, which may have increased susceptibility to cranial nerve damage. Bush et al. used a fractionated protocol prescribing 54-60 Gy(RBE) in 30 to 33 fractions (85). At mean follow-up of 34 months, LC was 100%, and no trigeminal or facial nerve toxicities were observed, with 31% maintaining useful hearing. Using the α-β model to compare doses of FSRT and SRS studies, this fractionated protocol prescribed roughly 40% more dose than the PSRS regimen, suggesting that a lower fractionated dose might resulted in better preservation of hearing while maintaining good LC. Vernimenn et al. used a hypo-fractionated proton therapy [26 Gy(RBE) over 3 fractions] to treat a group of patients with an average tumor volume of 5.3 cm3, which is among the largest studied in this group of tumors (86). At a mean follow-up of 72 months, hearing preservation was 42%, trigeminal and facial nerve preservation rates were 93% and 90.5%, respectively, and the 5-year LC rate was 98%, suggesting that this hypo-fractionated protocol was a good option for large, inoperable tumors. Baummert et al. compared dose distributions of photon and particle therapy and found that high-dose conformality was equal, but that proton therapy lowered the integral dose, concluding that particle therapy may be particularly useful for larger lesions.
Glioblastoma mutliforme
Glioblastoma multiforme (GBM) is the most common primary malignant brain tumor in adults. Early studies dating from 1978 demonstrated that radiotherapy after resection more than doubled overall survival to 8.0 months compared to 3.2 months with observation alone, establishing the effectiveness of radiotherapy in management of GBM (87). With the current standard, post-surgical, teomozolamide-based chemoradiotherapy, overall median progression-free survival is approximately 7 months, with OS of 15 months (88). Current recommendations for RT include dosages up to 60 Gy given in daily fractions of 2 Gy to the enhancing area of the tumor with 1-2 cm margins. Using this standard radiotherapy regimen, however, 80-90% of tumors recur within 2 cm of the original lesion.
In an effort to improve tumor control, several studies have used proton therapy to escalate doses up to 90 Gy(RBE). Using a combination of photons and protons, MGH treated 23 GBM patients to a total dose of 90 Gy(RBE) to the gross tumor volume, 64.8 Gy(RBE) to the 2-cm margin encompassing the gross tumor volume, and 50.4 Gy(RBE) to area of surrounding edema plus 2-cm margins using accelerated fractionation (89). Stratified by RTOG prognostic classes, this plan consistently increased median survival time to 23, 17, and 14 months for RTOG Classes III, IV and V, respectively. This compares with the 17.9, 11.1, and 8.9 months median survival for respective classes seen in previous RTOG trials using standard doses of RT with chemotherapy (90). Of the 23 patients treated to these high doses, only one had tumor recurrence within the dose-escalated region. Despite the better control in the high-dose region and increased median survival, all patients in whom tissue was obtained (n=7) developed radiation necrosis, and neurological deterioration was observed in most patients.
A more recent study from Tsukuba also dose-escalated to 96.6 Gy(RBE) over 56 fractions, obtaining a median survival of 21.6 months in 21 patients, most having RTOG Class IV GBM (91). Stratifying by size of the enhancing tumor, it was found that acute side-effects were tolerable with smaller tumor volumes. However, this study was not able to comment on late-effects of the radiation, because it was difficult for imaging to distinguish between tumor recurrence and necrosis. These studies demonstrate dose-escalation up to 96 Gy(RBE) with proton therapy provides effective LC and offers some increase in median survival. At such high doses, however, the radiation necrosis experienced represents a significant toxicity and may limit quality of life benefits. Nonetheless, when combined with concurrent chemotherapy, particle therapy at intermediate doses may yet play an important role in the treatment of these aggressive tumors. Mizoe et al. used carbon ion RT to provide a boost of 16.8-24.8 Gy(RBE) to the tumor volume with concurrent nimustine hydrochloride in 32 patients. Median survival was 17 months and progression-free survival ranged from 7-19 months, depending on the dose of carbon-ion boost to the tumor. The incidence of late-toxicities appears low, with only four patients experiencing Grade 2 brain reactions. European investigators are also actively studying the potential utility of carbon ions, utilizing their inherently higher RBE in an attempt to overcome radiation resistance for gliomas and other brain tumors (92,93).
Discussion and future directions
Particle therapy promises to expand the therapeutic ratio for radiotherapy. The central tenants of radiation therapy remain treatment of the tumor and sparing of normal tissues. Given the high implementation costs, however, substantial controversy remains. In calculating the cost effectiveness of various radiation modalities, it is of great importance to consider the potential implications of late adverse effects. Indeed, several studies have used models to demonstrate the potential cost-benefits of particle therapy (94-97). These studies concluded that, when used to treat carefully selected patients, particle therapy has the potential to be cost saving through decreased toxicities and increased quality of life.
Another major criticism of particle therapy is the lack of clinical evidence showing benefit in efficacy and toxicity compared with the best photon therapy. For many years, the number of trials will be limited due to the lack of proton treatment facilities and the small number of patients at each site. In 2002, only two proton therapy facilities were in operation in the US. Currently, there are only ten proton centers operational in the US. As clinical evidence accumulates, it is hoped that the long-term cost benefits of normal tissue sparing will be realized.
There are several developing areas in particle therapy which may offer even greater benefit. To date, the majority of published studies on particle therapy for CNS tumors have used PSPT. Active-scanning techniques, including IMPT, may offer improved conformity while maintaining dose sparing of normal tissues. Active scanning, by not requiring the scattering foils used for passive scattering, creates fewer neutrons at beam delivery, thereby further minimizing the risk of inducing secondary malignancies. As scanning-beam treatments are implemented, there may also be an opportunity for biologic treatment planning with proton therapy, as the RBE of proton beams may increase at the most distal edge, although to a much lesser extent than is observed with heavier particles (98,99). Although carbon-ion RT may also offer increased efficacy for radiation-resistant tumors, there are currently only a few institutions worldwide offering carbon-ion RT, and none in the US.
As more institutions adopt particle therapy, the potential of particle therapy is expected to translate into improved outcomes over photon therapy. Published data are quite promising, but much still remains to be learned about the role of particle therapy.
Acknowledgments
Funding: None.
Footnote
Provenance and Peer Review: This article was commissioned by the Guest Editors (Huan Giap and Eric Y Chuang) for the series “Particle Beam Therapy I” published in Translational Cancer Research. The article has undergone external peer review.
Conflicts of Interest: All authors have completed the ICMJE uniform disclosure form (available at http://dx.doi.org/10.3978/j.issn.2218-676X.2012.10.02). The series “Particle Beam Therapy I” was commissioned by the editorial office without any funding or sponsorship. The authors have no other conflicts of interest to declare.
Ethical Statement: The authors are accountable for all aspects of the work in ensuring that questions related to the accuracy or integrity of any part of the work are appropriately investigated and resolved.
Open Access Statement: This is an Open Access article distributed in accordance with the Creative Commons Attribution-NonCommercial-NoDerivs 4.0 International License (CC BY-NC-ND 4.0), which permits the non-commercial replication and distribution of the article with the strict proviso that no changes or edits are made and the original work is properly cited (including links to both the formal publication through the relevant DOI and the license). See: https://creativecommons.org/licenses/by-nc-nd/4.0/.
References
- Mertens AC, Yasui Y, Neglia JP, et al. Late mortality experience in five-year survivors of childhood and adolescent cancer: the Childhood Cancer Survivor Study. J Clin Oncol 2001;19:3163-72. [PubMed]
- Armstrong GT, Liu Q, Yasui Y, et al. Long-term outcomes among adult survivors of childhood central nervous system malignancies in the Childhood Cancer Survivor Study. J Natl Cancer Inst 2009;101:946-58. [PubMed]
- Armstrong GT, Stovall M, Robison LL. Long-term effects of radiation exposure among adult survivors of childhood cancer: results from the childhood cancer survivor study. Radiat Res 2010;174:840-50. [PubMed]
- Ellenberg L, Liu Q, Gioia G, et al. Neurocognitive status in long-term survivors of childhood CNS malignancies: a report from the Childhood Cancer Survivor Study. Neuropsychology 2009;23:705-17. [PubMed]
- Thomas PR, Deutsch M, Kepner JL, et al. Low-stage medulloblastoma: final analysis of trial comparing standard-dose with reduced-dose neuraxis irradiation. J Clin Oncol 2000;18:3004-11. [PubMed]
- Tukenova M, Guibout C, Oberlin O, et al. Role of cancer treatment in long-term overall and cardiovascular mortality after childhood cancer. J Clin Oncol 2010;28:1308-15. [PubMed]
- Hein PA, Gladstone DJ, Bellerive MR, Hug EB. Importance of protocol target definition on the ability to spare normal tissue:an IMRT and 3D-CRT planning comparison for intraorbital tumors. Int J Radiat Oncol Biol Phys. 2005;62:1540-8. [PubMed]
- Lee CT, Bilton SD, Famiglietti RM, et al. Treatment planning with protons for pediatric retinoblastoma, medulloblastoma, and pelvic sarcoma: how do protons compare with other conformal techniques? Int J Radiat Oncol Biol Phys 2005;63:362-72. [PubMed]
- St Clair WH, Adams JA, Bues M, et al. Advantage of protons compared to conventional X-ray or IMRT in the treatment of a pediatric patient with medulloblastoma. Int J Radiat Oncol Biol Phys 2004;58:727-34. [PubMed]
- Moeller BJ, Chintagumpala M, Philip JJ, et al. Low early ototoxicity rates for pediatric medulloblastoma patients treated with proton radiotherapy. Radiat Oncol 2011;6:58. [PubMed]
- Huang E, Teh BS, Strother DR, et al. Intensity-modulated radiation therapy for pediatric medulloblastoma: early report on the reduction of ototoxicity. Int J Radiat Oncol Biol Phys 2002;52:599-605. [PubMed]
- Polkinghorn WR, Dunkel IJ, Souweidane MM, et al. Disease control and ototoxicity using intensity-modulated radiation therapy tumor-bed boost for medulloblastoma. Int J Radiat Oncol Biol Phys 2011;81:e15-20. [PubMed]
- ICRP Publication 60: 1990 Recommendations of the International Commission on Radiological Protection 1991.
- Miralbell R, Lomax A, Cella L, et al. Potential reduction of the incidence of radiation-induced second cancers by using proton beams in the treatment of pediatric tumors. Int J Radiat Oncol Biol Phys 2002;54:824-9. [PubMed]
- Mu X, Björk-Eriksson T, Nill S, et al. Does electron and proton therapy reduce the risk of radiation induced cancer after spinal irradiation for childhood medulloblastoma? A comparative treatment planning study. Acta Oncol 2005;44:554-62. [PubMed]
- Chung CS, Keating N, Yock TY, et al. Comparative analysis of second malignancy risk in patients treated with proton therapy versus conventional photon therapy. Int J Radiat Oncol Biol Phys 2008;72:8.
- Merchant TE, Kiehna EN, Li C, et al. Radiation dosimetry predicts IQ after conformal radiation therapy in pediatric patients with localized ependymoma. Int J Radiat Oncol Biol Phys 2005;63:1546-54. [PubMed]
- MacDonald SM, Safai S, Trofimov A, et al. Proton radiotherapy for childhood ependymoma: initial clinical outcomes and dose comparisons. Int J Radiat Oncol Biol Phys 2008;71:979-86. [PubMed]
- Weber DC, Zilli T, Do HP, et al. Intensity modulated radiation therapy or stereotactic fractionated radiotherapy for infratentorial ependymoma in children: a multicentric study. J Neurooncol 2011;102:295-300. [PubMed]
- Schroeder TM, Chintagumpala M, Okcu MF, et al. Intensity-modulated radiation therapy in childhood ependymoma. Int J Radiat Oncol Biol Phys 2008;71:987-93. [PubMed]
- Merchant TE, Gould CJ, Xiong X, et al. Early neuro-otologic effects of three-dimensional irradiation in children with primary brain tumors. Int J Radiat Oncol Biol Phys 2004;58:1194-207. [PubMed]
- Benesch M, Weber-Mzell D, Gerber NU, et al. Ependymoma of the spinal cord in children and adolescents: a retrospective series from the HIT database. J Neurosurg Pediatr 2010;6:137-44. [PubMed]
- Lonjon M, Goh KY, Epstein FJ. Intramedullary spinal cord ependymomas in children: treatment, results and follow-up. Pediatr Neurosurg 1998;29:178-83. [PubMed]
- O’Sullivan C, Jenkin RD, Doherty MA, et al. Spinal cord tumors in children: long-term results of combined surgical and radiation treatment. J Neurosurg 1994;81:507-12. [PubMed]
- Merchant TE, Kiehna EN, Thompson SJ, et al. Pediatric low-grade and ependymal spinal cord tumors. Pediatr Neurosurg 2000;32:30-6. [PubMed]
- Al-Halabi H, Montes JL, Atkinson J, et al. Adjuvant radiotherapy in the treatment of pediatric myxopapillary ependymomas. Pediatr Blood Cancer 2010;55:639-43. [PubMed]
- Amsbaugh MJ, Grosshans DR, McAleer MF, et al. Proton therapy for spinal ependymomas: planning, acute toxicities, and preliminary outcomes. Int J Radiat Oncol Biol Phys 2012;83:1419-24. [PubMed]
- Steňo J, Bízik I, Steňo A, et al. Craniopharyngiomas in children: how radical should the surgeon be? Childs Nerv Syst 2011;27:41-54. [PubMed]
- Becker KL. Craniopharyngiomas. In: Becker KL. eds. Principles and Practice of Endocrinology and Metabolism. Philadelphia, PA: Lippincott Williams & Wilkins, 2000:957.
- Crom DB, Smith D, Xiong Z, et al. Health status in long-term survivors of pediatric craniopharyngiomas. J Neurosci Nurs 2010;42:323-8; quiz 329-30. [PubMed]
- Boehling NS, Grosshans DR, Bluett JB, et al. Dosimetric comparison of three-dimensional conformal proton radiotherapy, intensity-modulated proton therapy, and intensity-modulated radiotherapy for treatment of pediatric craniopharyngiomas. Int J Radiat Oncol Biol Phys 2012;82:643-52. [PubMed]
- Beltran C, Roca M, Merchant TE. On the benefits and risks of proton therapy in pediatric craniopharyngioma. Int J Radiat Oncol Biol Phys 2012;82:e281-7. [PubMed]
- Luu QT, Loredo LN, Archambeau JO, et al. Fractionated proton radiation treatment for pediatric craniopharyngioma: preliminary report. Cancer J 2006;12:155-9. [PubMed]
- Merchant TE, Hua CH, Shukla H, et al. Proton versus photon radiotherapy for common pediatric brain tumors: comparison of models of dose characteristics and their relationship to cognitive function. Pediatr Blood Cancer 2008;51:110-7. [PubMed]
- Fitzek MM, Linggood RM, Adams J, et al. Combined proton and photon irradiation for craniopharyngioma: long-term results of the early cohort of patients treated at Harvard Cyclotron Laboratory and Massachusetts General Hospital. Int J Radiat Oncol Biol Phys 2006;64:1348-54. [PubMed]
- Maity A, Shu HK, Janss A, et al. Craniospinal radiation in the treatment of biopsy-proven intracranial germinomas: twenty-five years’ experience in a single center. Int J Radiat Oncol Biol Phys 2004;58:1165-70. [PubMed]
- Aoyama H, Shirato H, Ikeda J, et al. Induction chemotherapy followed by low-dose involved-field radiotherapy for intracranial germ cell tumors. J Clin Oncol 2002;20:857-65. [PubMed]
- Jennings MT, Gelman R, Hochberg F. Intracranial germ-cell tumors: natural history and pathogenesis. J Neurosurg 1985;63:155-67. [PubMed]
- MacDonald SM, Trofimov A, Safai S, et al. Proton radiotherapy for pediatric central nervous system germ cell tumors: early clinical outcomes. Int J Radiat Oncol Biol Phys 2011;79:121-9. [PubMed]
- Hug EB, Muenter MW, Archambeau JO, et al. Conformal proton radiation therapy for pediatric low-grade astrocytomas. Strahlenther Onkol 2002;178:10-7. [PubMed]
- Laithier V, Grill J, Le Deley MC, et al. Progression-free survival in children with optic pathway tumors: dependence on age and the quality of the response to chemotherapy--results of the first French prospective study for the French Society of Pediatric Oncology. J Clin Oncol 2003;21:4572-8. [PubMed]
- Petronio J, Edwards MS, Prados M, et al. Management of chiasmal and hypothalamic gliomas of infancy and childhood with chemotherapy. J Neurosurg 1991;74:701-8. [PubMed]
- Awdeh RM, Kiehna EN, Drewry RD, et al. Visual outcomes in pediatric optic pathway glioma after conformal radiation therapy. Int J Radiat Oncol Biol Phys 2012;84:46-51. [PubMed]
- Fuss M, Hug EB, Schaefer RA, et al. Proton radiation therapy (PRT) for pediatric optic pathway gliomas: comparison with 3D planned conventional photons and a standard photon technique. Int J Radiat Oncol Biol Phys 1999;45:1117-26. [PubMed]
- Dohrmann GJ, Farwell JR, Flannery JT. Astrocytomas in childhood: a population-based study. Surg Neurol 1985;23:64-8. [PubMed]
- McAllister B, Archambeau JO, Nguyen MC, et al. Proton therapy for pediatric cranial tumors: preliminary report on treatment and disease-related morbidities. Int J Radiat Oncol Biol Phys 1997;39:455-60. [PubMed]
- Packer RJ, Biegel JA, Blaney S, et al. Atypical teratoid/rhabdoid tumor of the central nervous system: report on workshop. J Pediatr Hematol Oncol 2002;24:337-42. [PubMed]
- Burger PC, Yu IT, Tihan T, et al. Atypical teratoid/rhabdoid tumor of the central nervous system: a highly malignant tumor of infancy and childhood frequently mistaken for medulloblastoma: a Pediatric Oncology Group study. Am J Surg Pathol 1998;22:1083-92. [PubMed]
- Rorke LB, Packer RJ, Biegel JA. Central nervous system atypical teratoid/rhabdoid tumors of infancy and childhood: definition of an entity. J Neurosurg 1996;85:56-65. [PubMed]
- Weinblatt M, Kochen J. Rhabdoid tumor of the central nervous system. Med Pediatr Oncol 1992;20:258. [PubMed]
- Olson TA, Bayar E, Kosnik E, et al. Successful treatment of disseminated central nervous system malignant rhabdoid tumor. J Pediatr Hematol Oncol 1995;17:71-5. [PubMed]
- Chen YW, Wong TT, Ho DM, et al. Impact of radiotherapy for pediatric CNS atypical teratoid/rhabdoid tumor (single institute experience). Int J Radiat Oncol Biol Phys 2006;64:1038-43. [PubMed]
- Tekautz TM, Fuller CE, Blaney S, et al. Atypical teratoid/rhabdoid tumors (ATRT): improved survival in children 3 years of age and older with radiation therapy and high-dose alkylator-based chemotherapy. J Clin Oncol 2005;23:1491-9. [PubMed]
- Squire SE, Chan MD, Marcus KJ. Atypical teratoid/rhabdoid tumor: the controversy behind radiation therapy. J Neurooncol 2007;81:97-111. [PubMed]
- Kieran MW. A Single-Arm, Open Label Limited-Institutional Phase II Study of Multi-Agent Intrathecal and Systemic Chemotherapy With Radiation Therapy for Children < or = 18 Years With Newly Diagnosed Central Nervous System Atypical Teratoid/Rhabdoid Tumor. 2004: DFCI.
- al-Mefty O, Kersh JE, Routh A, et al. The long-term side effects of radiation therapy for benign brain tumors in adults. J Neurosurg 1990;73:502-12. [PubMed]
- Meyers CA, Brown PD. Role and relevance of neurocognitive assessment in clinical trials of patients with CNS tumors. J Clin Oncol 2006;24:1305-9. [PubMed]
- Johannesen TB, Lien HH, Hole KH, et al. Radiological and clinical assessment of long-term brain tumour survivors after radiotherapy. Radiother Oncol 2003;69:169-76. [PubMed]
- Crossen JR, Garwood D, Glatstein E, et al. Neurobehavioral sequelae of cranial irradiation in adults: a review of radiation-induced encephalopathy. J Clin Oncol 1994;12:627-42. [PubMed]
- Gleason JF Jr, Case D, Rapp SR, et al. Symptom clusters in patients with newly-diagnosed brain tumors. J Support Oncol 2007;5:427-33, 436. [PubMed]
- Meyers CA, Weitzner MA, Valentine AD, et al. Methylphenidate therapy improves cognition, mood, and function of brain tumor patients. J Clin Oncol 1998;16:2522-7. [PubMed]
- Kong DS, Lee JI. The efficacy of fractionated radiotherapy and stereotactic radiosurgery for pituitary adenomas: long-term results of 125 consecutive patients treated in a single institution. Cancer 2007;110:854-60. [PubMed]
- Petit JH, Biller BM, Coen JJ, et al. Proton stereotactic radiosurgery in management of persistent acromegaly. Endocr Pract 2007;13:726-34. [PubMed]
- Petit JH, Biller BM, Yock TI, et al. Proton stereotactic radiotherapy for persistent adrenocorticotropin-producing adenomas. J Clin Endocrinol Metab 2008;93:393-9. [PubMed]
- Castinetti F, Nagai M, Dufour H, et al. Gamma knife radiosurgery is a successful adjunctive treatment in Cushing’s disease. Eur J Endocrinol 2007;156:91-8. [PubMed]
- Devin JK, Allen GS, Cmelak AJ, et al. The efficacy of linear accelerator radiosurgery in the management of patients with Cushing’s disease. Stereotact Funct Neurosurg 2004;82:254-62. [PubMed]
- Cho CB, Park HK, Joo WI, et al. Stereotactic Radiosurgery with the CyberKnife for Pituitary Adenomas. J Korean Neurosurg Soc 2009;45:157-63. [PubMed]
- Höybye C, Grenbäck E, Rähn T, et al. Adrenocorticotropic hormone-producing pituitary tumors: 12- to 22-year follow-up after treatment with stereotactic radiosurgery. Neurosurgery 2001;49:284-91; discussion 291-2. [PubMed]
- Castinetti F, Taieb D, Kuhn JM, et al. Outcome of gamma knife radiosurgery in 82 patients with acromegaly: correlation with initial hypersecretion. J Clin Endocrinol Metab 2005;90:4483-8. [PubMed]
- Attanasio R, Epaminonda P, Motti E, et al. Gamma-knife radiosurgery in acromegaly: a 4-year follow-up study. J Clin Endocrinol Metab 2003;88:3105-12. [PubMed]
- Pollock BE, Nippoldt TB, Stafford SL, et al. Results of stereotactic radiosurgery in patients with hormone-producing pituitary adenomas: factors associated with endocrine normalization. J Neurosurg 2002;97:525-30. [PubMed]
- Voges J, Kocher M, Runge M, et al. Linear accelerator radiosurgery for pituitary macroadenomas: a 7-year follow-up study. Cancer 2006;107:1355-64. [PubMed]
- Jezková J, Marek J, Hána V, et al. Gamma knife radiosurgery for acromegaly--long-term experience. Clin Endocrinol (Oxf) 2006;64:588-95. [PubMed]
- Minniti G, Amichetti M, Enrici RM. Radiotherapy and radiosurgery for benign skull base meningiomas. Radiat Oncol 2009;4:42. [PubMed]
- Wenkel E, Thornton AF, Finkelstein D, et al. Benign meningioma: partially resected, biopsied, and recurrent intracranial tumors treated with combined proton and photon radiotherapy. Int J Radiat Oncol Biol Phys 2000;48:1363-70. [PubMed]
- Noël G, Bollet MA, Calugaru V, et al. Functional outcome of patients with benign meningioma treated by 3D conformal irradiation with a combination of photons and protons. Int J Radiat Oncol Biol Phys 2005;62:1412-22. [PubMed]
- Debus J, Wuendrich M, Pirzkall A, et al. High efficacy of fractionated stereotactic radiotherapy of large base-of-skull meningiomas: long-term results. J Clin Oncol 2001;19:3547-53. [PubMed]
- Dufour H, Muracciole X, Métellus P, et al. Long-term tumor control and functional outcome in patients with cavernous sinus meningiomas treated by radiotherapy with or without previous surgery: is there an alternative to aggressive tumor removal? Neurosurgery 2001;48:285-94; discussion 294-6. [PubMed]
- Takanashi M, Fukuoka S, Hojyo A, et al. Gamma knife radiosurgery for skull-base meningiomas. Prog Neurol Surg 2009;22:96-111. [PubMed]
- Shin M, Kurita H, Sasaki T, et al. Analysis of treatment outcome after stereotactic radiosurgery for cavernous sinus meningiomas. J Neurosurg 2001;95:435-9. [PubMed]
- Weber DC, Schneider R, Goitein G, et al. Spot scanning-based proton therapy for intracranial meningioma: long-term results from the Paul Scherrer Institute. Int J Radiat Oncol Biol Phys 2012;83:865-71. [PubMed]
- Smouha EE, Yoo M, Mohr K, et al. Conservative management of acoustic neuroma: a meta-analysis and proposed treatment algorithm. Laryngoscope 2005;115:450-4. [PubMed]
- Arthurs BJ, Fairbanks RK, Demakas JJ, et al. A review of treatment modalities for vestibular schwannoma. Neurosurg Rev 2011;34:265-77; discussion 277-9. [PubMed]
- Harsh GR, Thornton AF, Chapman PH, et al. Proton beam stereotactic radiosurgery of vestibular schwannomas. Int J Radiat Oncol Biol Phys 2002;54:35-44. [PubMed]
- Bush DA, McAllister CJ, Loredo LN, et al. Fractionated proton beam radiotherapy for acoustic neuroma. Neurosurgery 2002;50:270-3; discussion 273-5. [PubMed]
- Vernimmen FJ, Mohamed Z, Slabbert JP, et al. Long-term results of stereotactic proton beam radiotherapy for acoustic neuromas. Radiother Oncol 2009;90:208-12. [PubMed]
- Walker MD, Alexander E Jr, Hunt WE, et al. Evaluation of BCNU and/or radiotherapy in the treatment of anaplastic gliomas. A cooperative clinical trial. J Neurosurg 1978;49:333-43. [PubMed]
- Stupp R, Mason WP, van den Bent MJ, et al. Radiotherapy plus concomitant and adjuvant temozolomide for glioblastoma. N Engl J Med 2005;352:987-96. [PubMed]
- Fitzek MM, Thornton AF, Rabinov JD, et al. Accelerated fractionated proton/photon irradiation to 90 cobalt gray equivalent for glioblastoma multiforme: results of a phase II prospective trial. J Neurosurg 1999;91:251-60. [PubMed]
- Curran WJ Jr, Scott CB, Horton J, et al. Recursive partitioning analysis of prognostic factors in three Radiation Therapy Oncology Group malignant glioma trials. J Natl Cancer Inst 1993;85:704-10. [PubMed]
- Mizumoto M, Tsuboi K, Igaki H, et al. Phase I/II trial of hyperfractionated concomitant boost proton radiotherapy for supratentorial glioblastoma multiforme. Int J Radiat Oncol Biol Phys 2010;77:98-105. [PubMed]
- Combs SE, Kieser M, Rieken S, et al. Randomized phase II study evaluating a carbon ion boost applied after combined radiochemotherapy with temozolomide versus a proton boost after radiochemotherapy with temozolomide in patients with primary glioblastoma: the CLEOPATRA trial. BMC Cancer 2010;10:478. [PubMed]
- Rieken S, Habermehl D, Haberer T, et al. Proton and carbon ion radiotherapy for primary brain tumors delivered with active raster scanning at the Heidelberg Ion Therapy Center (HIT): early treatment results and study concepts. Radiat Oncol 2012;7:41. [PubMed]
- Lundkvist J, Ekman M, Ericsson SR, et al. Economic evaluation of proton radiation therapy in the treatment of breast cancer. Radiother Oncol 2005;75:179-85. [PubMed]
- Lundkvist J, Ekman M, Ericsson SR, et al. Proton therapy of cancer: potential clinical advantages and cost-effectiveness. Acta Oncol 2005;44:850-61. [PubMed]
- Lundkvist J, Ekman M, Ericsson SR, et al. Cost-effectiveness of proton radiation in the treatment of childhood medulloblastoma. Cancer 2005;103:793-801. [PubMed]
- Pommier P, Lievens Y, Feschet F, et al. Simulating demand for innovative radiotherapies: an illustrative model based on carbon ion and proton radiotherapy. Radiother Oncol 2010;96:243-9. [PubMed]
- Paganetti H, Olko P, Kobus H, et al. Calculation of relative biological effectiveness for proton beams using biological weighting functions. Int J Radiat Oncol Biol Phys 1997;37:719-29. [PubMed]
- Calugaru V, Nauraye C, Noël G, et al. Radiobiological characterization of two therapeutic proton beams with different initial energy spectra used at the Institut Curie Proton Therapy Center in Orsay. Int J Radiat Oncol Biol Phys 2011;81:1136-43. [PubMed]