The expanding role of stereotactic body radiation therapy for pancreatic cancer: a review of the literature
Introduction
In 2015 alone, an estimated 48,960 individuals will be diagnosed with pancreatic cancer and approximately 40,560 patients will die of the disease (1). While surgical resection with negative margins remains the only potentially curative treatment for this deadly disease, less than 20% of patients have resectable disease at presentation. Most patients are diagnosed with locally advanced pancreatic cancer (LAPC) or borderline resectable pancreatic cancer (BRPC). Treatment for these patients consists primarily of chemotherapy given the high incidence of occult metastatic disease.
The role of radiation therapy (RT) remains controversial for pancreatic cancer patients with unresectable disease (2,3). While many succumb to effects of distant metastases, others experience local disease progression for which RT may be helpful. An autopsy series from Johns Hopkins University suggests that up to one-third of pancreatic cancer patients die primarily of local tumor progression without significant distant metastatic disease (4). Although RT may benefit such patients, receiving daily treatment for at least 5 weeks using standard fractionation, with interruption from the delivery of full doses of systemic chemotherapy, is not ideal in patients given the high risk of systemic disease spread and limited life expectancy.
Stereotactic body radiation therapy (SBRT) has evolved into a standard option for early stage non-small cell lung cancer (5), and is now being tested for a variety of other malignancies (6). It has garnered significant interest for pancreatic cancer patients since it is completed quickly over 1-5 fractions, requires less time away from full doses of chemotherapy, and is generally much better tolerated than conventional RT as a result of more limited target volumes. Favorable results of SBRT for LAPC patients are now leading to the exploration of SBRT for other pancreatic cancer patients.
Here we review the published literature concerning pancreatic SBRT clinical outcomes, treatment planning considerations, and cost-effectiveness.
SBRT for locally advanced pancreas
The utility of pancreatic SBRT was established in the locally advanced patient population. Approximately 35% of patients with pancreatic cancer present with LAPC (7). Following publication of data from the Gastrointestinal Tumor Study Group in the 1980s, chemoradiation (CRT) became a standard option for LAPC (2). The addition of 5-fluorouracil (5FU) to RT substantially improved median survival (10 months) when compared to monotherapy with RT (5.5 months) or chemotherapy (7.4 months).
With the advent of gemcitabine-based (gem) chemotherapy, the role of RT for LAPC has become more precarious (8). Studies of up front 5FU-based CRT have shown that a significant proportion of LAPC patients experience disease progression and death within weeks of treatment (9). These factors have led many to employ a wait-and-see approach, with RT held until patients declare themselves stable after systemic therapy. A retrospective study by the European GERCOR team showed value in this approach (10). In patients deemed radiographically stable after induction chemotherapy, further treatment with CRT showed an improved median survival over chemotherapy alone (15 vs. 11.7 months). This strategy was tested prospectively in the European Phase III LAP 07 trial comparing induction gem followed by capecitabine-based CRT or additional gem-based chemotherapy (3). In contrast to the retrospective study, the LAP 07 trial showed a marginally greater median survival benefit with chemotherapy alone (16.5 vs. 15.3 months). Despite trial shortcomings such as inconsistent patient randomization, many physicians have inferred that chemotherapy alone should be the new standard for LAPC. This conclusion was supported by the European FFCD/SFRO Phase III trial comparing gem alone versus induction 5FU and cisplatin CRT, followed by maintenance gem (11). Overall survival (OS) was shortened in the CRT arm from 13 to 8.6 months. Grade 3 toxicity was greater with CRT during both induction (36% vs. 22%) and maintenance (32% vs. 18%) phases. Notably, the trial utilized a higher than normal conventionally fractionated 60 Gy dose.
Given the superior response rates with gem, an Eastern Cooperative Group (ECOG) study sought to evaluate the utility of gem-based CRT versus gem alone for LAPC (12). Although patients in the CRT arm developed greater grade 4-5 toxicity (41% vs. 9%), they still demonstrated improved OS from 9.2 to 11.1 months. A subsequent phase 2 study from Europe, the SCALOP trial, examined the impact of induction chemotherapy followed by either capecitabine- based or gem-based CRT (13). Results favored concurrent capecitabine plus radiation with a median survival of 15.2 vs. 13.4 months. One caveat of this trial is that patients received 300 mg/m2 of weekly gem with radiation, half the 600 mg/m2 dose used with RT in the ECOG study.
The recent success of more aggressive, but increasingly toxic, chemotherapy regimens such as FOLFIRINOX and gem plus nab-paclitaxel have spurred re-examination of local therapy (14,15). With improved systemic control, local progression may become a more serious issue for survival and quality of life. However, local control rates from standard external beam radiotherapy (EBRT) have been disappointing. Most series report a 1-year local progression rate of about 50% (13). Furthermore, with two-thirds of patients failing distantly within 1 year, a shorter course approach with minimal interruption to systemic therapy is desirable (13). These factors paved the way for the use of SBRT in pancreatic cancer patients, and initially those with LAPC.
The inception of SBRT for pancreatic cancers began with a phase I dose escalation study in a LAPC cohort at Stanford (16). The trial was stopped at a dose of 25 Gy since all patients achieved local control with distant metastasis as the first site of failure. The median survival for all patients was 11 months, with 100% local control at the 25 Gy dose level. Following publication of this study, additional institutional series have been reported using various fractionation schedules with and without systemic therapy. Table 1 provides a comparison of these institutional experiences. With rare exception, the local control rates in these selected patients treated with SBRT have been markedly higher than with standard EBRT regimens.
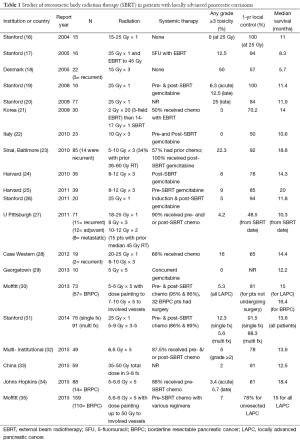
Full table
Stanford researchers followed their phase I report with another study assessing the utility of SBRT in combination with a standard 45 Gy dose of EBRT (17). While grade 3 toxicity increased from 0 to 12.5%, the median survival remained largely unchanged. The authors concluded that there was no benefit with the integration of SBRT and EBRT compared to SBRT alone. A later study from Korea showed that combination therapy was feasible with dose reductions in both EBRT and SBRT courses (21). This approach resulted in 14-month median survival, 70% 1-year local control, rate, and 3% grade 3 toxicity. Other groups have also utilized SBRT as boost radiotherapy after EBRT, and found it well tolerated (36).
Unfortunately, not all of the early experiences with SBRT proved positive. A group from Denmark reported their initial experience with 22 patients receiving a regimen of 45 Gy in 3 fractions for LAPC. Half of their patients developed grade 3 toxicities with only a 57% 1-year local control and 5.7 months median survival (18). Closer analysis suggests that these toxicities may have been related to the larger treatment volumes used in this study. Whereas the Stanford group utilized a 2-3 mm gross tumor volume (GTV) to planning target volume (PTV) expansion, the Danish group added 5-10 mm margins to GTV plus edema for PTV (18,31). However, despite smaller margins and less acute toxicity patients treated on the Stanford single fraction SBRT protocol experienced a high degree of late toxicities (25% grade >2) (20). This prompted a change to fractionated SBRT at Stanford and outcomes were recently compared with single fraction SBRT at that institution (31). Single fraction patients were treated to 25 Gy in 1 fraction whereas the hypofractionated-fraction cohort received a range of doses between 25-45 Gy in 5 fractions. The median dose fractionation schedule for the hypofractionated-fraction group was 6.6 Gy in 5 fractions to 33 Gy. This regimen was calculated to have an equivalent BED to 25 Gy in 1 fraction based on the universal survival curve reported by Park et al. (37). Despite larger PTV volumes (median 57 vs. 45 cc, P=0.004), the hypofractionated-fraction group showed reduced 1-year grade ≥2 toxicity from 26.1% to 7.8%. This reduction came without a compromise in disease control. The 1-year local control was 91.5% vs. 88.3% (P=0.8) for single vs. 5-fraction SBRT, and the median OS was 13.6 months for all patients. More contemporary SBRT series have also largely employed a fractionated approach, and confirmed tumor control stability with reduced toxicity (24,25,30,32,35). These institutional studies reveal a median survival of 14-15 months, 1-year local control rates of about 80%, and grade 3 toxicities below 10%.
As with EBRT, the sequencing of chemotherapy has had a measurable impact on patient outcome. The group from Beth Israel initially reported on a series of 36 patients treated with SBRT (24-36 Gy in 3 fractions) followed by adjuvant gem chemotherapy (24). This strategy yielded a 78% 1-year local control rate, 14.3 median OS, and 8% grade ≥3 toxicity rate. However, when they employed 2 cycles of induction gem, and selected only those patients for SBRT who demonstrated disease stability, their results improved significantly (25). This selected patient cohort demonstrated a median OS of 20 months with a 1-year local control rate of 85%. Using this approach, 17% of patients were spared RT. Given the rapid rate of disease progression, these patients would likely not have benefitted from local therapy.
Very recently, a few groups have reported that LAPC patients may have an increased likelihood of undergoing resection after aggressive induction chemotherapy regimens. Earlier this year, the group from Hopkins reported on 88 patients treated from 2010-14 with SBRT using gem-based or FOLFIRINOX regimens (34). SBRT doses ranged from 25-33 Gy in 5 fractions. The 1-year local control rate was 61%, but with a median OS of 18.4 months for LAPC patients. Notably, 20% of LAPC patients underwent surgery. Resected patients had a median OS of 20.2 months, compared to 12.3 months for unresected cases. Grade 3 toxicity was below 6%. Similar to the study from Hopkins, SBRT data from Moffitt also shows the possibility of downstaging for surgery (35). They reported a 24% surgical conversion rate for LAPC patients receiving FOLFIRINOX chemotherapy. All converted patients achieved an R0 (microscopic negative margin) resection. Any grade 3 or higher toxicity was 7%. Median OS was 34.2 months for patients who underwent resection, and 11.3 months for those who did not.
The improved local control with SBRT, decreased systemic progression with newer chemotherapies, and the possibility of conversion of unresectable to resectable disease with combined approaches has provided new hope to a disease with an overall dismal prognosis. There are several new trials looking at the combination of radiation and more aggressive chemotherapy for LAPC. CONKO-007, SCALOP-2, and NRG trials are assessing novel chemotherapy regimens with EBRT. A phase III trial from Stanford will compare FOLFIRINOX with or without SBRT (NCT01926197).
SBRT for borderline resectable pancreas
While pancreatic SBRT has been most extensively evaluated in LAPC patients, there are emerging data that SBRT may also benefit patients with BRPC. The SBRT literature for BRPC largely comes from the Moffitt Cancer Center. Following the initial publication of outcomes in 30 BRPC patients who received induction GTX (gem, docetaxel, and capecitabine) followed by SBRT with favorable surgical outcomes and minimal toxicity (38), Chuong et al. then reported on a larger series of 73 patients (57 BRPC, 16 LAPC) who received the same treatment regimen (30). Fiducial markers were routinely placed under endoscopic ultrasound guidance to assist with target volume delineation, pre-treatment motion assessment, and daily image guidance. Patients were treated with either respiratory gating or abdominal compression. SBRT was delivered using 5 consecutive daily fractions targeting the primary tumor with a 3-5 mm margin for setup uncertainty. While the primary tumor was prescribed a median dose of 30 Gy (range, 25-30 Gy), the region of vasculature involvement was prescribed a median dose of 35 Gy (range, 35-50 Gy) using a simultaneous integrated boost (SIB) to further increase the likelihood of tumor regression and R0 resection. The SIB dose and volume were determined for each patient on a case-by-case basis and were largely dictated by the proximity of the stomach, duodenum, and small bowel. The SIB volume was generally made to cover as much of the primary tumor as possible including the tumor-vessel interface while respecting normal tissue constraints. After restaging, 56.1% of the BRPC patients underwent surgical resection with all except for one (96.9%) having negative margins. Portal vein (PV) or superior mesenteric vein (SMV) resection and reconstruction was performed in 18.8%. Resected patients had significantly improved median OS (19.3 vs. 12.3 months; P=0.03) and median PFS (12.7 vs. 5 months; P<0.0001). No acute ≥ grade 3 toxicities were reported and the most common acute toxicities were grade 1-2 fatigue and/or nausea. Another update of the Moffitt experience using the same treatment strategy was recently published that included 159 patients (110 BRPC, 49 LAPC) (35). Similar favorable surgical outcomes were reported. Surgical resection was performed on 51% of the BRPC patients and R0 resection was achieved in 96%. PV or SMV resection and reconstruction was performed in 34% of BRPC patients. Median OS was significantly higher among patients who had surgery compared to those who did not (34.2 vs. 14.0 months; P<0.001). Finally, while the prescription doses generally increased compared to the previous publication (primary tumor: median 30 vs. 35 Gy; tumor-vessel interface: median 35 vs. 40 Gy), the incidence of late grade 3 radiation-related toxicity remained consistently low (~5%).
The feasibility of using SBRT for BRPC is also supported by other studies with more limited numbers of BRPC patients. A study from Johns Hopkins included 88 patients (74 LAPC, 14 BRPC) who received 5-fraction SBRT and reported favorable surgical and SBRT-related toxicity outcomes (39). Investigators from the University of Pittsburgh published their experience of 12 patients (7 BRPC, 5 LAPC) who received chemotherapy followed by SBRT prescribed to 36 Gy in 3 fractions (n=7) or 24 Gy in a single fraction (n=5) and then had surgery (40). A high rate of R0 resection was achieved (92%) with minimal toxicity. Pathologic complete response (pCR) was achieved in 25%, which is higher than would be expected with standard EBRT and perhaps signaling that SBRT may have unique histopathologic effects. In contrast, the pCR rate was 7% in the most recent Moffitt publication. Despite smaller patient numbers in the Pittsburgh study, it is plausible that a higher rate of pCR may be achieved using dose fractionation schedules with a higher biologically effective dose assuming an α/β ratio of 10 (BED10): 36 Gy in 3 fractions, BED10 =79.2 Gy; 24 Gy × 1, BED10 =81.6 Gy; 6 Gy × 5, BED10 =48 Gy (41). However, the potential benefit of using dose fractionation schedules with a higher BED must be carefully balanced against the corresponding risk of normal tissue injury. Lastly, the degree of tumor regression after induction chemotherapy and 5-fraction SBRT was formally evaluated by Chuong et al. using two grading system who found that most patients had marked tumor response (42). However, the specific histopathologic effects compared to CRT are not clear and further studies are needed.
Despite the favorable surgical outcomes after SBRT and CRT, the use of chemotherapy alone is preferred at some institutions. However, the efficacy of treatment regimens that do not include radiation should be questioned. He et al. compared surgical outcomes among BRPC/LAPC patients who received SBRT (n=29), CRT (n=82), or chemotherapy alone (n=26) and reported R0 resection rates of 90%, 84%, and 62%, respectively (P=0.02) (43). The pCR rate was notably higher among patients who received SBRT (21% vs. 4% vs. 0%; P<0.001). On the other hand, an analysis of 43 BRPC/LAPC patients from The Ohio State University included an 85.7% R0 resection rate after modified FOLFIRINOX; the clinical significance of this is limited by the small number of patients who had surgery (n=21) (44).
In conclusion, while various neoadjuvant treatment regimens are commonly used for BRPC including standard fractionation CRT, increasing consideration should be given to SBRT based on favorable outcomes as reported above. Several phase I trials (NCT01992705, NCT02308722, NCT01446458) are ongoing and a multi-institutional randomized trial for BRPC that includes SBRT is under development.
SBRT in the adjuvant setting
As in LAPC, the potential risk of both locoregional and distant disease recurrence in the adjuvant setting is significant. Utilization of an abbreviated radiation course with SBRT may allow more patients to receive systemic therapy with minimal delay. The tolerability of adjuvant SBRT as well as its efficacy in achieving locoregional control compared to conventional fractionation currently remains under investigation. In a single institution series by Rwigema et al., 24 patients with evidence of close or positive margins after pancreaticoduodenectomy received SBRT, most commonly with a single fraction of 24 Gy (45). Nearly 60% of patients received chemotherapy prior to SBRT and almost 90% received chemotherapy following SBRT. Radiation treatment volumes were contoured with the assistance of fiducial markers and surgical oncology input with regards to sites at highest risk of harboring residual microscopic disease. With median follow up of 12.5 months (range, 1.4-39.5 months) clinical outcomes were promising including 1-year freedom from local progression rate of 66% and median survival of 26.7 months. There were no reported ≥ acute or late grade 3 toxicities.
While there are no other published reports of pancreatic SBRT in the adjuvant setting, investigators from Johns Hopkins are prospectively evaluating the combination of adjuvant SBRT, FOLFIRINOX, a pancreatic cancer vaccine (GVAX), and immunomodulating doses of cyclophosphamide (NCT01595321). The initial results of this trial will be presented later this year.
While the merits of using adjuvant SBRT versus standard CRT are not yet known, it is imperative to remain cognizant that not all resected pancreatic cancer patients are ideal candidates for SBRT. First, because of the limited nature of SBRT treatment volumes the region at highest risk for microscopic residual disease should be clearly identified through the placement of surgical clips or other fiducial markers, as well as the surgeon’s input when possible, as was done in the studies mentioned above. Also, patients in whom high dose per fraction would be delivered to the jejunal reconstruction are likely better suited receiving standard CRT. Finally, consideration should also be given to the higher volume of small bowel that is likely to overlap with the target volume in the postoperative versus neoadjuvant or definitive settings.
SBRT re-irradiation or boost after conventional fractionation RT
Modern adjuvant CRT series demonstrate that locoregional relapse is the first site of treatment failure in 25-30% of patients (46). Similarly, data from the SCALOP study shows that local relapse can occur in ~30% of LAPC patients receiving definitive CRT with 5FU or gem (13). Autopsy reports also indicate that ~30% of pancreatic patients die with evidence of locally progressive disease, without distant metastases (4).
As such, SBRT can be an effective form of re-irradiation for limited locoregional relapses following standard EBRT for locally advanced tumors. Lominska et al. retrospectively studied 28 patients with unresectable locally recurrent or progressive disease who either received an SBRT boost within 2 months after completing conventional RT or salvage SBRT following documented local recurrence or progression (36). Treatments were predominantly localized to gross disease visualized on CT or PET/CT although inclusion of regions encompassing subclinical disease was allowed at the discretion of the treating physician. CyberKnife was used to deliver various dose fractionation schedules with the most common being 24 Gy in 3 fractions (n=10), 21 Gy in 3 fractions (n=9), and 22.5 Gy in 3 fractions (n=3). Their findings included a 1-year freedom from local progression of 70%, as well as a median OS of 5.9 months. These outcomes were achieved with limited toxicity consisting of only one case of acute grade 2 nausea/vomiting and two cases of late grade 3 small bowel obstruction and gastric perforation, both of which resolved with conservative management.
Similarly, Wild et al. analyzed a multi-institutional series of 18 patients who received SBRT re-irradiation for isolated local recurrences or isolated local progression after having received prior CRT either neoadjuvantly (n=4), adjuvantly (n=11) or definitively (n=3) (47). SBRT was delivered largely using 5 Gy × 5 (89%) to gross disease. Median survival from time of SBRT was 8.8 months. However, patients who experienced local recurrence/progression more than 9 months from initial CRT had longer median survival after salvage therapy than when progression occurred in under 9 months (11.3 vs. 3.4 months). After SBRT, freedom from local progression at 6 and 12 months was 78% and 62%, respectively. Treatments were delivered without any acute grade ≥3 events and with only one patient experiencing late small bowel obstruction.
Based on the above series, it is therefore feasible and tolerable to deliver SBRT treatments for localized disease following prior conventional RT. As highlighted by Wild et al., the utility of re-irradiation with SBRT may be best achieved in those patients with the longest time between prior conventional EBRT and recurrence/progression. The optimal dose fractionation and normal tissue constraints to be used in the re-irradiation setting require further evaluation.
Treatment planning
SBRT permits precise, conformal delivery of high dose radiation to target tissues using single or hypofractionated courses (Figure 1). These higher doses are believed to generate an ablative response clinically analogous to surgical resection (48). Unlike in EBRT, where areas beyond the gross tumor are targeted for eradication of subclinical disease, SBRT doses must be limited to the tumor to minimize normal tissue destruction. Therefore, a clinical treatment volume (CTV) is rarely employed. Most patients are treated to targets consisting of the GTV plus small margins accounting for tumor motion and setup error (PTV). PET-CT imaging is often utilized during the treatment planning process to accurately delineate the GTV and normal tissues such as the duodenum and pancreas. These structures are often difficult to distinguish by CT alone (49).
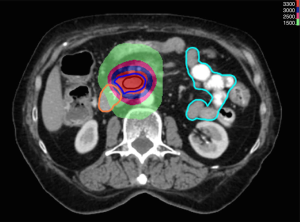
Given the small margins used for treatment, precise accounting and control of tumor motion is paramount for successful SBRT delivery. Ideally, tumor motion should be kept at an absolute minimum in order to prevent a geographic miss while limiting prohibitively large target margins. Unlike in intracranial radiosurgery, SBRT targets are subject to considerable movement from respiration or cardiac motion. Huguet et al. documented maximum tumor displacements of 0.35, 0.57, and 1.34 cm in the left-right (LR), anterior-posterior (AP) and superior-inferior (SI) directions, respectively, during the respiratory cycle (50). Cranio-caudal (CC) motion is the most severe due to diaphragmatic motion.
Various strategies have been developed to limit respiratory motion. These include abdominal compression, active breathing control, and respiratory gating to limit treatment time to certain phases of the respiratory cycle. In addition, real time tumor tracking can potentially increase precision of dose delivery to target volumes. The series by Huguet et al. showed that respiratory gating to the mid-cycle of the patient’s breathing pattern could reduce GTV motion uncertainty by 17% in the LR direction, 63% in the AP direction, 60% in the SI direction (50). Similarly, utilization of abdominal compression can achieve motion reductions by as much as 62% in the CC direction, and 38% in the AP direction (51). With respect to active breath holding techniques, Nakamura et al. showed that by using end expiration-breath holding, they were able to limit maximum displacement to 3.3 mm for intrafraction pancreatic motion, as well as limit interfraction motion to a maximum of 5 mm in the SI direction (52).
Although there are several effective strategies to limit pancreatic motion, no technique can eliminate it. Therefore, dynamic imaging studies are necessary to document target movement during SBRT. One strategy is to account for tumor motion during treatment planning. This is typically accomplished through 4D CT imaging, which displays tumor and normal tissue positioning throughout the respiratory cycle. 4D CT data permits gated treatments in pre-defined phases of the respiratory cycle or the creation of an internal target volume (ITV) accounting for GTV motion. A second option is to actively adjust dose delivery during treatment by monitoring target motion real time. Robotic systems, such as CyberKnife, utilize this approach by adjusting dose delivery to tumor motion inferred through fiducial marker displacement.
As in most institutions, our policy for SBRT pancreas planning omits elective nodal irradiation to prevent prohibitively large field sizes. For LAPC and BRPC patients, we delineate the primary tumor and FDG-avid or enlarged (>1 cm) lymphadenopathy as target structures. ITV margins are then determined by quantifying GTV motion with 4D imaging, or through motion monitoring of surrogate markers such intra-tumor fiducials or stents that may be identified during image-guided therapy. For SBRT cases in the setting of recurrent cancers, utilization of patterns of failure mapping such as those by Dholakia et al. can be effective in creating appropriate CTV volumes (53). PTV margins, usually between 2-5 mm, are subsequently placed in all cases to account for set up error.
SBRT treatments can be delivered through non-isocentric techniques such as CyberKnife, or isocentric methods such as volumetric modulated arc therapy (VMAT) and intensity modulated radiation therapy (IMRT). Multiple beam angles are utilized in static or rotational fashion (54) to distribute high doses away from organs at risk (OAR) and provide improved dose conformality to the tumor. Various case series have evaluated VMAT and IMRT dosimetry for pancreatic malignancies. Kumar et al. compared duodenal dosing using VMAT and static IMRT delivery for single fraction treatment of 25 Gy. While both VMAT and static IMRT improved duodenal sparing, static IMRT resulted in inferior target volume coverage (55). Additionally, VMAT permits expedited treatment time with less monitor units than IMRT (56). Our institution has similarly compared static IMRT, 1-arc VMAT, and 2-arc VMAT techniques for 5-fraction SBRT. These unpublished data indicate that 2-arc VMAT provides the most significant sparing of OARs, especially of small bowel and duodenum, and does so without compromising target volume coverage. Furthermore, the benefit of 2-arc VMAT over the other techniques increases as the distance between the PTV and the OAR decreases.
Normal tissue tolerance
Although higher radiation doses improve local control and histopathologic response, the dose than can safely be used to treat pancreas cancer is limited by the proximity of normal organs, especially the stomach, duodenum, and small bowel (18,57-60). Radiation dose received by these GI luminal structures can result in complications ranging from ulceration and bleeding to obstruction, stricture, and perforation. While the dose-volume effects for GI luminal structures are better understood for standard fractionation (61), the tolerance of these radiosensitive organs is becoming better understood when using a single fraction or hypofractionation approach (57,62).
A study from Stanford University was the first to demonstrate that single-fraction pancreatic SBRT results in duodenal toxicity in a dose-dependent manner (60). Murphy et al. evaluated 73 unresectable pancreatic cancer patients who were prescribed 25 Gy in 1 fraction. Grade 2 or higher duodenal toxicity was reported in 12 patients (16%); 6- and 12-month actuarial duodenal toxicity rates were 11% and 29%, respectively. Dosimetric evaluation showed that duodenal toxicity was correlated with V15, V20, and maximum dose thus emphasizing the need to minimize the volume of duodenum receiving higher doses. The importance of restricting higher doses to limited volumes is further supported by the Danish phase II trial, which as mentioned above, reported a 64% incidence of > grade 2 GI toxicity with 45 Gy in 3 fractions. This result remains discordant with other pancreatic SBRT experiences that have reported late GI toxicities rates <10-15% (18).
A study from South Korea evaluated GI toxicity after SBRT for abdominopelvic malignancies that were prescribed a median 45 Gy in 3 fractions. Using a 2-4 mm expansion from GTV to PTV, the authors reported severe gastroduodenal toxicity in 6 of 40 patients (15%) and severe intestinal toxicity in 6 of 55 patients (11%) in two separate analyses (63,64). Maximum stomach or duodenal doses of 35 and 38 Gy were associated with a 5% and 10% probability of severe gastroduodenal toxicity, respectively. V25 ≤20 mL was associated with a significantly lower incidence of severe intestinal toxicity (4% vs. 50%). Their data also suggested that intestinal toxicity was reduced if treatment was not given on 3 consecutive days, but rather over 4-8 total days.
The potential advantage of non-consecutive day SBRT treatment requires further evaluation, but severe GI toxicity rates from pancreas SBRT series treating on consecutive days are low. For example, 33 Gy in 5 consecutive fractions was well tolerated in a prospective evaluation of SBRT for LAPC with median follow up of 13.9 months (acute and late ≥ grade 2 GI toxicities were 2% and 11%, respectively) (32). The long-term outcomes of 5-fraction SBRT for BRPC and LAPC from the Moffitt Cancer Center also included low rates of toxicity with median follow up of 14 months (35). Based on these fairly low rates of toxicity using 5 fractions, it is certainly plausible that increasing the number of fractions decreases the potential benefit of treating in non-consecutive days. The use of 5 fractions may also decrease clinical toxicity compared to use of fewer fractions. As mentioned previously, Stanford’s comparison of single fraction versus 5-fraction pancreatic SBRT demonstrated that fractionated SBRT was associated with markedly lower rates of 12-month ≥ grade 3 GI toxicity (5.6% vs. 12.3%) (31).
Finally, several groups have performed systematic literature reviews of pancreatic SBRT and have reported collective toxicity outcomes culled from these publications (57,62). Using 20 studies that included a total of 721 patients, Brunner and colleagues found that ≥ grade 3 acute toxicity was exceedingly uncommon. The majority of patients had LAPC and did not have surgery after SBRT. The incidence of ≥ grade 3 late toxicity was also low, and in most of the included studies was <10%. Higher prescription dose was correlated to higher incidence of late GI toxicity: using biologically equivalent doses in 2 Gy fractions (EQD2), a 5% probability of late grade 2 and late grade 3 toxicities were associated with prescription doses of 65 and 80 Gy, respectively. Elhammali et al. also reviewed 16 publications including 1,160 patients and reported a median late grade 3 or higher GI toxicity rate of 7.4% that most frequently included non-hemorrhagic ulcers (62).
With the increasing use of SBRT for various tumor types, normal tissue constraints were published in 2008 even though most of these were not validated (65). As of today there remains a lack of consensus regarding normal tissue constraints or dose fractionation schedules to guide pancreatic SBRT treatment planning. This situation is in part the consequence of the diversity of constraints used at various institutions. Although, there is certainly a need to clarify these issues, our understanding of appropriate constraints, particularly using 5-fraction SBRT, has improved markedly with the evolution of SBRT literature (32,35).
Cost effectiveness
Utilization of SBRT for pancreatic carcinoma allows significantly shorter treatment times than those required for conventionally fractionated radiation. Five-fraction SBRT courses are also reimbursed at a lower rate than conventional 28-fraction EBRT as per the 2015 Medicare freestanding reimbursement schedule ($11,000 vs. $20,000). As long as clinical outcomes remain comparable, SBRT may be more cost effective than standard EBRT. Unfortunately, there are limited comprehensive cost effectiveness data with respect to SBRT utilization for pancreatic carcinoma. A single series by Murphy et al. compared 4 treatment regimens for LAPC: gem alone, gem-SBRT, gem-3DRT, and gem-IMRT (66). The authors incorporated the relevant costs of chemotherapy, RT, and outpatient care. They analyzed these factors with the probability of varying clinical outcomes as extrapolated from ECOG 4201 and Stanford SBRT series to create a Markov decision model. They concluded that gem-SBRT was more cost-effective than gem-3DRT or gem-IMRT based on comparisons of an incremental cost effectiveness ratio-ICER ($/Quality Adjusted Life Year). Gem-SBRT had an ICER of $69,500/QALY compared to gem alone, whereas gem-3DRT presented an ICER of ~$126,800 compared with gem alone. Despite these findings, additional comparative analyses are needed to validate these conclusions in support of SBRT.
Conclusions
SBRT has been shown to be safe and effective in LAPC patients. It offers several advantages over standard EBRT including increased patient convenience, reduced toxicities, and the ability to minimize delays in modern multi-agent chemotherapy regimens such as FOLFIRINOX. The ability of SBRT to complete treatment in a compact time span may also be more cost effective than standard EBRT. The favorable SBRT outcomes for LAPC patients have paved the way for exploration of SBRT for BPRC and resected pancreatic cancer patients, with promising early results.
Acknowledgments
Funding: None.
Footnote
Provenance and Peer Review: This article was commissioned by the Guest Editors (R. Charles Nichols Jr, Debashish Bose and George P. Kim) for the series “Pancreatic Cancer” published in Translational Cancer Research. The article has undergone external peer review.
Conflicts of Interest: All authors have completed the ICMJE uniform disclosure form (available at http://dx.doi.org/10.3978/j.issn.2218-676X.2015.11.01). The series “Pancreatic Cancer” was commissioned by the editorial office without any funding or sponsorship. The authors have no other conflicts of interest to declare.
Ethical Statement: The authors are accountable for all aspects of the work in ensuring that questions related to the accuracy or integrity of any part of the work are appropriately investigated and resolved.
Open Access Statement: This is an Open Access article distributed in accordance with the Creative Commons Attribution-NonCommercial-NoDerivs 4.0 International License (CC BY-NC-ND 4.0), which permits the non-commercial replication and distribution of the article with the strict proviso that no changes or edits are made and the original work is properly cited (including links to both the formal publication through the relevant DOI and the license). See: https://creativecommons.org/licenses/by-nc-nd/4.0/.
References
- Siegel RL, Miller KD, Jemal A. Cancer statistics, 2015. CA Cancer J Clin 2015;65:5-29. [PubMed]
- Moertel CG, Frytak S, Hahn RG, et al. Therapy of locally unresectable pancreatic carcinoma: a randomized comparison of high dose (6000 rads) radiation alone, moderate dose radiation (4000 rads + 5-fluorouracil), and high dose radiation + 5-fluorouracil: The Gastrointestinal Tumor Study Group. Cancer 1981;48:1705-10. [PubMed]
- Hammel P, Huguet F, Van Laethem JL, et al. Comparison of chemoradiotherapy (CRT) and chemotherapy (CT) in patients with a locally advanced pancreatic cancer (LAPC) controlled after 4 months of gemcitabine with or without erlotinib: Final results of the international phase III LAP 07 study. J Clin Oncol 2013;33:abstr LBA4003.
- Iacobuzio-Donahue CA, Fu B, Yachida S, et al. DPC4 gene status of the primary carcinoma correlates with patterns of failure in patients with pancreatic cancer. J Clin Oncol 2009;27:1806-13. [PubMed]
- Videtic GM, Chang JY, Chetty IJ, et al. ACR appropriateness Criteria® early-stage non-small-cell lung cancer. Am J Clin Oncol 2014;37:201-7. [PubMed]
- Kollar L, Rengan R. Stereotactic body radiotherapy. Semin Oncol 2014;41:776-89. [PubMed]
- Heestand GM, Murphy JD, Lowy AM. Approach to patients with pancreatic cancer without detectable metastases. J Clin Oncol 2015;33:1770-8. [PubMed]
- Burris HA 3rd, Moore MJ, Andersen J, et al. Improvements in survival and clinical benefit with gemcitabine as first-line therapy for patients with advanced pancreas cancer: a randomized trial. J Clin Oncol 1997;15:2403-13. [PubMed]
- André T, Balosso J, Louvet C, et al. Combined radiotherapy and chemotherapy (cisplatin and 5-fluorouracil) as palliative treatment for localized unresectable or adjuvant treatment for resected pancreatic adenocarcinoma: results of a feasibility study. Int J Radiat Oncol Biol Phys 2000;46:903-11. [PubMed]
- Huguet F, André T, Hammel P, et al. Impact of chemoradiotherapy after disease control with chemotherapy in locally advanced pancreatic adenocarcinoma in GERCOR phase II and III studies. J Clin Oncol 2007;25:326-31. [PubMed]
- Chauffert B, Mornex F, Bonnetain F, et al. Phase III trial comparing intensive induction chemoradiotherapy (60 Gy, infusional 5-FU and intermittent cisplatin) followed by maintenance gemcitabine with gemcitabine alone for locally advanced unresectable pancreatic cancer. Definitive results of the 2000-01 FFCD/SFRO study. Ann Oncol 2008;19:1592-9. [PubMed]
- Loehrer PJ Sr, Feng Y, Cardenes H, et al. Gemcitabine alone versus gemcitabine plus radiotherapy in patients with locally advanced pancreatic cancer: an Eastern Cooperative Oncology Group trial. J Clin Oncol 2011;29:4105-12. [PubMed]
- Mukherjee S, Hurt CN, Bridgewater J, et al. Gemcitabine-based or capecitabine-based chemoradiotherapy for locally advanced pancreatic cancer (SCALOP): a multicentre, randomised, phase 2 trial. Lancet Oncol 2013;14:317-26. [PubMed]
- Von Hoff DD, Ervin T, Arena FP, et al. Increased survival in pancreatic cancer with nab-paclitaxel plus gemcitabine. N Engl J Med 2013;369:1691-703. [PubMed]
- Conroy T, Desseigne F, Ychou M, et al. FOLFIRINOX versus gemcitabine for metastatic pancreatic cancer. N Engl J Med 2011;364:1817-25. [PubMed]
- Koong AC, Le QT, Ho A, et al. Phase I study of stereotactic radiosurgery in patients with locally advanced pancreatic cancer. Int J Radiat Oncol Biol Phys 2004;58:1017-21. [PubMed]
- Koong AC, Christofferson E, Le QT, et al. Phase II study to assess the efficacy of conventionally fractionated radiotherapy followed by a stereotactic radiosurgery boost in patients with locally advanced pancreatic cancer. Int J Radiat Oncol Biol Phys 2005;63:320-3. [PubMed]
- Hoyer M, Roed H, Sengelov L, et al. Phase-II study on stereotactic radiotherapy of locally advanced pancreatic carcinoma. Radiother Oncol 2005;76:48-53. [PubMed]
- Schellenberg D, Goodman KA, Lee F, et al. Gemcitabine chemotherapy and single-fraction stereotactic body radiotherapy for locally advanced pancreatic cancer. Int J Radiat Oncol Biol Phys 2008;72:678-86. [PubMed]
- Chang DT, Schellenberg D, Shen J, et al. Stereotactic radiotherapy for unresectable adenocarcinoma of the pancreas. Cancer 2009;115:665-72. [PubMed]
- Seo Y, Kim MS, Yoo S, et al. Stereotactic body radiation therapy boost in locally advanced pancreatic cancer. Int J Radiat Oncol Biol Phys 2009;75:1456-61. [PubMed]
- Polistina F, Costantin G, Casamassima F, et al. Unresectable locally advanced pancreatic cancer: a multimodal treatment using neoadjuvant chemoradiotherapy (gemcitabine plus stereotactic radiosurgery) and subsequent surgical exploration. Ann Surg Oncol 2010;17:2092-101. [PubMed]
- Didolkar MS, Coleman CW, Brenner MJ, et al. Image-guided stereotactic radiosurgery for locally advanced pancreatic adenocarcinoma results of first 85 patients. J Gastrointest Surg 2010;14:1547-59. [PubMed]
- Mahadevan A, Jain S, Goldstein M, et al. Stereotactic body radiotherapy and gemcitabine for locally advanced pancreatic cancer. Int J Radiat Oncol Biol Phys 2010;78:735-42. [PubMed]
- Mahadevan A, Miksad R, Goldstein M, et al. Induction gemcitabine and stereotactic body radiotherapy for locally advanced nonmetastatic pancreas cancer. Int J Radiat Oncol Biol Phys 2011;81:e615-22. [PubMed]
- Schellenberg D, Kim J, Christman-Skieller C, et al. Single-fraction stereotactic body radiation therapy and sequential gemcitabine for the treatment of locally advanced pancreatic cancer. Int J Radiat Oncol Biol Phys 2011;81:181-8. [PubMed]
- Rwigema JC, Parikh SD, Heron DE, et al. Stereotactic body radiotherapy in the treatment of advanced adenocarcinoma of the pancreas. Am J Clin Oncol 2011;34:63-9. [PubMed]
- Goyal K, Einstein D, Ibarra RA, et al. Stereotactic body radiation therapy for nonresectable tumors of the pancreas. J Surg Res 2012;174:319-25. [PubMed]
- Gurka MK, Collins SP, Slack R, et al. Stereotactic body radiation therapy with concurrent full-dose gemcitabine for locally advanced pancreatic cancer: a pilot trial demonstrating safety. Radiat Oncol 2013;8:44. [PubMed]
- Chuong MD, Springett GM, Freilich JM, et al. Stereotactic body radiation therapy for locally advanced and borderline resectable pancreatic cancer is effective and well tolerated. Int J Radiat Oncol Biol Phys 2013;86:516-22. [PubMed]
- Pollom EL, Alagappan M, von Eyben R, et al. Single- versus multifraction stereotactic body radiation therapy for pancreatic adenocarcinoma: outcomes and toxicity. Int J Radiat Oncol Biol Phys 2014;90:918-25. [PubMed]
- Herman JM, Chang DT, Goodman KA, et al. Phase 2 multi-institutional trial evaluating gemcitabine and stereotactic body radiotherapy for patients with locally advanced unresectable pancreatic adenocarcinoma. Cancer 2015;121:1128-37. [PubMed]
- Song Y, Yuan Z, Li F, et al. Analysis of clinical efficacy of CyberKnife(®) treatment for locally advanced pancreatic cancer. Onco Targets Ther 2015;8:1427-31. [PubMed]
- Moningi S, Marciscano AE, Rosati LM, et al. Stereotactic body radiation therapy in pancreatic cancer: the new frontier. Expert Rev Anticancer Ther 2014;14:1461-75. [PubMed]
- Mellon EA, Hoffe SE, Springett GM, et al. Long-term outcomes of induction chemotherapy and neoadjuvant stereotactic body radiotherapy for borderline resectable and locally advanced pancreatic adenocarcinoma. Acta Oncol 2015;54:979-85. [PubMed]
- Lominska CE, Unger K, Nasr NM, et al. Stereotactic body radiation therapy for reirradiation of localized adenocarcinoma of the pancreas. Radiat Oncol 2012;7:74. [PubMed]
- Park C, Papiez L, Zhang S, et al. Universal survival curve and single fraction equivalent dose: useful tools in understanding potency of ablative radiotherapy. Int J Radiat Oncol Biol Phys 2008;70:847-52. [PubMed]
- Chuong MD, Springett GM, Weber J, et al. Induction gemcitabine-based chemotherapy and neoadjuvant stereotactic body radiation therapy achieve high margin-negative resection rates for borderline resectable pancreatic cancer. J Radiat Oncol 2012;1:273-81.
- Moningi S, Dholakia AS, Raman SP, et al. The Role of Stereotactic Body Radiation Therapy for Pancreatic Cancer: A Single-Institution Experience. Ann Surg Oncol 2015;22:2352-8. [PubMed]
- Rajagopalan MS, Heron DE, Wegner RE, et al. Pathologic response with neoadjuvant chemotherapy and stereotactic body radiotherapy for borderline resectable and locally-advanced pancreatic cancer. Radiat Oncol 2013;8:254. [PubMed]
- Mellon EA, Hoffe SE, Springett GM, et al. Long-term outcomes of induction chemotherapy and neoadjuvant stereotactic body radiotherapy for borderline resectable and locally advanced pancreatic adenocarcinoma. Acta Oncol 2015;54:979-85. [PubMed]
- Chuong MD, Frakes JM, Figura N, et al. Histopathologic tumor response after induction chemotherapy and stereotactic body radiation therapy for borderline resectable pancreatic cancer. J Gastrointest Oncol 2015; [Epub ahead of print].
- He J, Moningi S, Blair AB, et al. Surgical outcomes of patients with pancreatic cancer treated with stereotactic body radiation therapy. J Clin Oncol 2015;33:abstr 341.
- Blazer M, Wu C, Goldberg RM, et al. Neoadjuvant modified (m) FOLFIRINOX for locally advanced unresectable (LAPC) and borderline resectable (BRPC) adenocarcinoma of the pancreas. Ann Surg Oncol 2015;22:1153-9. [PubMed]
- Rwigema JC, Heron DE, Parikh SD, et al. Adjuvant stereotactic body radiotherapy for resected pancreatic adenocarcinoma with close or positive margins. J Gastrointest Cancer 2012;43:70-6. [PubMed]
- Regine WF, Winter KA, Abrams RA, et al. Fluorouracil vs gemcitabine chemotherapy before and after fluorouracil-based chemoradiation following resection of pancreatic adenocarcinoma: a randomized controlled trial. JAMA 2008;299:1019-26. [PubMed]
- Wild AT, Hiniker SM, Chang DT, et al. Re-irradiation with stereotactic body radiation therapy as a novel treatment option for isolated local recurrence of pancreatic cancer after multimodality therapy: experience from two institutions. J Gastrointest Oncol 2013;4:343-51. [PubMed]
- Stauder MC, Miller RC. Stereotactic Body Radiation Therapy (SBRT) for Unresectable Pancreatic Carcinoma. Cancers (Basel) 2010;2:1565-75. [PubMed]
- Ford EC, Herman J, Yorke E, et al. 18F-FDG PET/CT for image-guided and intensity-modulated radiotherapy. J Nucl Med 2009;50:1655-65. [PubMed]
- Huguet F, Yorke ED, Davidson M, et al. Modeling pancreatic tumor motion using 4-dimensional computed tomography and surrogate markers. Int J Radiat Oncol Biol Phys 2015;91:579-87. [PubMed]
- Wunderink W, Méndez Romero A, de Kruijf W, et al. Reduction of respiratory liver tumor motion by abdominal compression in stereotactic body frame, analyzed by tracking fiducial markers implanted in liver. Int J Radiat Oncol Biol Phys 2008;71:907-15. [PubMed]
- Nakamura M, Shibuya K, Shiinoki T, et al. Positional reproducibility of pancreatic tumors under end-exhalation breath-hold conditions using a visual feedback technique. Int J Radiat Oncol Biol Phys 2011;79:1565-71. [PubMed]
- Dholakia AS, Kumar R, Raman SP, et al. Mapping patterns of local recurrence after pancreaticoduodenectomy for pancreatic adenocarcinoma: a new approach to adjuvant radiation field design. Int J Radiat Oncol Biol Phys 2013;87:1007-15. [PubMed]
- Potters L, Kavanagh B, Galvin JM, et al. American Society for Therapeutic Radiology and Oncology (ASTRO) and American College of Radiology (ACR) practice guideline for the performance of stereotactic body radiation therapy. Int J Radiat Oncol Biol Phys 2010;76:326-32. [PubMed]
- Kumar R, Wild AT, Ziegler MA, et al. Stereotactic body radiation therapy planning with duodenal sparing using volumetric-modulated arc therapy vs intensity-modulated radiation therapy in locally advanced pancreatic cancer: a dosimetric analysis. Med Dosim 2013;38:243-50. [PubMed]
- Sapkaroski D, Osborne C, Knight KA. A review of stereotactic body radiotherapy - is volumetric modulated arc therapy the answer? J Med Radiat Sci 2015;62:142-51. [PubMed]
- Brunner TB, Nestle U, Grosu AL, et al. SBRT in pancreatic cancer: what is the therapeutic window? Radiother Oncol 2015;114:109-16. [PubMed]
- Chang ST, Goodman KA, Yang GP, et al. Stereotactic body radiotherapy for unresectable pancreatic cancer. Front Radiat Ther Oncol 2007;40:386-94. [PubMed]
- Hirata T, Teshima T, Nishiyama K, et al. Histopathological effects of preoperative chemoradiotherapy for pancreatic cancer: an analysis for the impact of radiation and gemcitabine doses. Radiother Oncol 2015;114:122-7. [PubMed]
- Murphy JD, Christman-Skieller C, Kim J, et al. A dosimetric model of duodenal toxicity after stereotactic body radiotherapy for pancreatic cancer. Int J Radiat Oncol Biol Phys 2010;78:1420-6. [PubMed]
- Kelly P, Das P, Pinnix CC, et al. Duodenal toxicity after fractionated chemoradiation for unresectable pancreatic cancer. Int J Radiat Oncol Biol Phys 2013;85:e143-9. [PubMed]
- Elhammali A, Patel M, Weinberg B, et al. Late gastrointestinal tissue effects after hypofractionated radiation therapy of the pancreas. Radiat Oncol 2015;10:186. [PubMed]
- Bae SH, Kim MS, Cho CK, et al. Predictor of severe gastroduodenal toxicity after stereotactic body radiotherapy for abdominopelvic malignancies. Int J Radiat Oncol Biol Phys 2012;84:e469-74. [PubMed]
- Bae SH, Kim MS, Kim SY, et al. Severe intestinal toxicity after stereotactic ablative radiotherapy for abdominopelvic malignancies. Int J Colorectal Dis 2013;28:1707-13. [PubMed]
- Timmerman RD. An overview of hypofractionation and introduction to this issue of seminars in radiation oncology. Semin Radiat Oncol 2008;18:215-22. [PubMed]
- Murphy JD, Chang DT, Abelson J, et al. Cost-effectiveness of modern radiotherapy techniques in locally advanced pancreatic cancer. Cancer 2012;118:1119-29. [PubMed]