High cystic fibrosis transmembrane conductance regulator expression in childhood B-cell acute lymphoblastic leukemia acts as a potential therapeutic target
Introduction
In previous years, we reported that cystic fibrosis transmembrane conductance regulator (CFTR), a well-known cAMP-activated anion channel belongs to ABC (ATP-binding cassette) transporter superfamily (1,2), played an unrecognized role in primitive and definitive hematopoiesis by regulating Wnt signaling (3). Moreover, we demonstrated that highly expressed CFTR was associated with acute lymphoblastic leukemia (4). CFTRinh-172, a classic CFTR inhibitor was also shown to exert a significant apoptosis-inducing, anti-proliferative, and cell cycle-arresting effect on leukemia cells with high CFTR expression (4,5).
B-cell acute lymphoblastic leukemia (B-ALL), the most common acute lymphoblastic leukemia in children (>85%) (6,7), is a neoplastic proliferation of B-cell lymphoblasts that primarily affects the bone marrow, blood, and lymph nodes. Outcomes for most children with B-ALL have improved drastically over the past 30 years through multiagent chemotherapy with some variation in regimens based on factors such as cytogenetics, age, and molecular genetics (8). However, up to 10% of patients will have disease that is refractory to initial treatment and about 40% to 70% will relapse (9). In particular, cases that harbor Philadelphia chromosome (Ph) encoding the BCR-ABL fusion protein continue to have a poorer prognosis (10,11).
To further explore the function of CFTR and its inhibitor CFTR-inh172 in acute lymphoblastic leukemia, we hypothesized d that CFTR may be associated with human childhood B-ALL, and CFTR inhibitor CFTR-inh172 may also be potent at treating B-ALL since the average protein level of CFTR in adult B-ALL primary leukemia cells is higher than normal control significantly (4). In the present study, we demonstrate that high expression of CFTR protein and mRNAs are associated with childhood B-ALL. The CFTR-Dvl2-β-catenin pathway we found in a previous study is conserved in B-ALL primary cells and that treatment with CFTR inhibitor CFTRinh-172 in B-ALL cell lines SUP-B15 cell-transplanted non-obese diabetic/severe combined immunodeficiency (NOD/SCID) mice prolong survival and slows leukemia progression.
We present the following article in accordance with the ARRIVE reporting checklist (available at https://tcr.amegroups.com/article/view/10.21037/tcr-21-2296/rc).
Methods
Leukemia patients’ information and lymphocytes isolation
Peripheral blood samples of childhood B-ALL patients were obtained from Chengdu Women’s & Children’s Central Hospital, West China Hospital and West China Second University Hospital. Bone marrow samples of childhood B-ALL patients were obtained from the Department of Pediatrics of the Chinese University of Hong Kong. The patients were diagnosed as having primary B-ALL according to the 2016 World Health Organization (WHO) classification of tumors of hematopoietic and the French-American-British (FAB) classification criteria and their information are presented in Tables S1,S2. Human lymphocytes were separated by kit with following the manufacturer’s procedures (LTS1077, TBDsciences, Tianjin, China). The control samples (n=10, Table S3) were from the Chengdu Women’s & Children’s Central Hospital, Chengdu Second People’s hospital and West China Second University Hospital. The control bone marrow samples (n=12, Table S4) were also obtained from the Department of Pediatrics of the Chinese University of Hong Kong.
The study was conducted in accordance with the 1964 Helsinki declaration and its later amendments. The study was approved by the ethics committee of West China Second University Hospital, Sichuan University (Approval ID: 2018, No. 32). All patients provided informed consent.
Western blotting
Whole-cell extracts were prepared by lysing cells in non-denaturing lysis buffer (137 mM NaCl, 20 mM Tris HCl pH 8, 10% glycerol, 1% NP-40, 2 mM EDTA). All the lysis buffers were supplemented with protease inhibitor cocktail (Cat. No. 04 693 132 001, Roche) and the conventional Western blotting assay was performed. Signal strength grayscale of western blotting assay was measured by software ImageJ. All detected sample expression was relative to internal reference protein.
Quantitative real-time polymerase chain reaction (qPCR) assay and statistics
TRIzol (Invitrogen, 15596-018) was used for exacting total RNA of each sample and 1µg of RNA was used for cDNA synthesis by PrimeScript RT reagent Kit (Takara, DRR037A). We performed qPCR using SYBR Green detection method and 7500 real-time PCR system (Applied Biosystems) with three independent biological replicates and three technical replicates. The detected primers were from the PrimerBank website (https://pga.mgh.harvard.edu/primerbank/). All detected gene expression was relative to β-actin. Quantitative data are presented as mean ± standard deviation (SD). Data was analyzed by comparative CT (cycle threshold) method (ΔΔCT method). Statistical significance is defined as *P<0.05, **P<0.01, and P<0.001(***).
Cell culture, transfection, siRNA, cell proliferation assay and luciferase reporter assays
SUP-B15 cells were cultured in IMDM (Gibco) supplemented with 20% fetal bovine serum (FBS) and 0.05 mM 2-mercaptoethanol; 697 cells were grown in RPMI-1640 plus 10% FBS. Cell lines were grown in a 5% CO2 atmosphere at 37 ℃. INTERFERin (Polyplus) was used for siRNA transfection (the final concentration was 100 nM). Human CFTR siRNA pair (5'-CGCGAUUUAUCUAGGCAUAdTdT-3' and 3'-dTdTGCGCUAAAUAGAUCCGUAU-5') and common negative control siRNA were designed and synthesized by Ribobio (Guangzhou, China). Another Human CFTR siRNA pair (5'-CGCGAUUUAUCUAGGCAUAdTdT-3' and 3'-dTdTGCGCUAAAUAGAUCCGUAU-5') was used for testing the effect and specificity in cells (data not shown). Cell viability rate was measured using the 3-[4,5-dimethylthiazol-2-yl]-2,5-diphenyl tetrazolium bromide (MTT) colorimetric reduction method described by Guo et al. (12). Lipofectamine 3000 (ThermoFisher) was used for TopFlash-luciferase plasmid and siRNA transfection, luciferase reporter assays were performed 2 days after transfection as previously described (3).
In vivo experiments
Male NOD/SCID mice were purchased from the Beijing Vital River Laboratory Animal Technology Co., Ltd. (China) and were maintained in the animal center of West China Second University Hospital (Sichuan University) under specific pathogen-free conditions. The leukemia murine model was established as described below. Briefly, before leukemia cells xenograft, mice were injected with 100 mg/kg cyclophosphamide (CTX) daily for 2 days. Then, five million SUP-B15 cells were injected into 5- to 7-week-old NOD/SCID mice via the intraperitoneal route. For the in vivo assessment of drug effects, 5 days after transplantation of the SUP-B15 cells, the mice were treated daily with 2.5 mg/kg CFTRinh-172 (or DMSO for the control group) via intraperitoneal injection for 7 days. Survival was monitored daily. Several mice were sacrificed 25 days after transplantation, and their spleens were removed.
All animal experiments in this study were in accordance with the Guide for the Care and Use of Laboratory Animals (Eighth Edition, 2011. ILARCLS, National Research Council, Washington, DC, USA) and were approved by the Animal Care and Use Committee of West China Second University Hospital, Sichuan University (Approval ID: HXDEYY20131021).
Reagents
CFTRinh-172 was purchased from Selleck (USA). CFTRinh-172 was dissolved in 100% dimethyl sulfoxide (DMSO) (Sigma, USA) to a stock concentration of 1 mg/mL and stored at −20 ℃.
Statistical analysis
Data are showed as the mean ± SD. One-way analysis of variance (ANOVA) with Dunnett as a post-test was used for statistical analysis. GraphPad prism software was used for two-tailed unpaired t-test. P values <0.05 were considered statistically significant.
Results
CFTR was highly expressed in childhood B-ALL patients
Since B-ALL is much more common (>85%) (6) in acute lymphoblastic leukemia patients, we continued to collect the peripheral blood of human childhood patients diagnosed with primary B-ALL as well as non-leukemia controls (Tables S1,S3 for patient and control information) and isolated lymphocytes for further analysis, after the investigation of the association of CFTR with adult acute lymphoblastic leukemia (4). We investigated the expression of CFTR protein in childhood primary B-ALL peripheral blood samples by western blotting assay. The results showed that CFTR expression in non-leukemia lymphocytes was very low. However, among the 10 cases of childhood B-ALL primary leukemia cells included in this work, CFTR expressed higher in all detected B-ALL cases (Figure 1A).
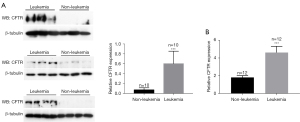
We also observed significantly increased CFTR mRNA levels measured by quantitative real-time RT-PCR (qPCR) in the bone marrows of B-ALL patients compared to that of normal controls (Figure 1B, Tables S2,S4 for patient and control information). These results indicated that CFTR protein and mRNA are expressed highly in primary B-ALL patients.
The CFTR-Wnt pathway is evolutionally conserved in primary childhood B-ALL
Aberrantly enhanced Wnt signaling has been associated with human leukemia pathogenesis (13,14). Our previous work demonstrated that CFTR was involved in Wnt-dependent hematopoiesis in zebrafish model (3), which prompted us to test whether the expression levels of CFTR, DVL2 and β-catenin are altered in childhood B-cell leukemia patients.
Immunofluorescence assay detected strong CFTR and DVL2 signals in almost all B-ALL cells in contrast to the less intense and scattered signals in the controls (Figure 2A). Of note, β-catenin showed cytoplastic pattern in control samples whereas nuclear localization of β-catenin was found in B-ALL lymphocytes (Figure 2B) indicating an activation of Wnt signaling in B-ALL. We also detected an interaction of CFTR with DVL2 in human B-ALL cell line SUP-B15 (Figure 2C) suggesting that the interaction between CFTR and DVL observed in zebrafish is conserved in humans. Importantly, knockdown of CFTR by siRNA in SUP-B15 cells and another B-ALL cell line 697 reduced the protein levels of DVL2 and β-catenin (Figure 2D and Figure S1A as well as mRNA levels Wnt/β-catenin downstream targets C-MYC and LEF1 72h post transfection (Figure 2E). Subsequently, we tested the effect of CFTR siRNA on the TopFlash luciferase activity in SUP-B15 and 697 cells and observed a significant reduction (Figure 2F and Figure S1B), confirming the function of CFTR in regulating β-catenin-dependent Wnt signaling. Furthermore, CFTR knockdown significantly reduced the viability of SUP-B15 cells in MTT test (Figure 2G). Taken together, these results suggest an evolutionally conserved role of CFTR in regulating hematopoiesis, a defect which may contribute to the development human leukemia, particularly B-ALL.
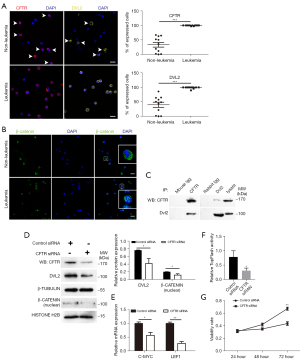
CFTR inhibitor CFTR-inh172 is highly active against SUP-B15-dependent B-ALL in vivo
To expand on the findings from previous B-ALL patients and cell lines, we established B-ALL xenograft model in NOD/SCID mice using SUP-B15 cells (https://cdn.amegroups.cn/static/public/TCR-21-2296-Appendix1.pdf). Mice were transplanted with B-ALL cell line SUP-B15 and treated with CFTR inhibitor CFTRinh-172 2.5 mg/kg/day by intraperitoneal injection 5 days after transplantation. After 7 days of treatment, mice receiving CFTRinh-172 had significant reductions in splenomegaly (Figure 3A) as well as decreased B-ALL in the spleen and bone marrow (Figure 3B,3C). We treated additional cohorts of mice transplanted with SUP-B15 for investigating body weight and survival. Indeed, CFTRinh-172 increased overall body weight and survival compared to vehicle DMSO treatment (Figure 4). CFTRinh-172 prolonged overall survival compared with vehicle DMSO with the median survival being 27.8 days in vehicle DMSO-treated mice compared to 45.8 days in mice that received CFTRinh-172 for 7 days.
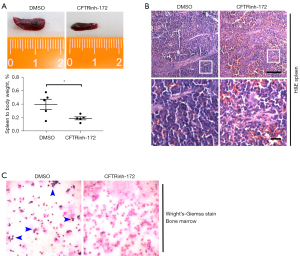
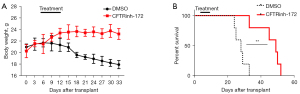
Discussion
To further investigate the role of CFTR in leukemia, we detected CFTR expression in human childhood B-ALL patients and the results showed that high CFTR expression was associated with childhood B-ALL. Subsequently, conserved molecular mechanism of CFTR regulating Wnt signaling was also be identified in B-ALL. Furthermore, CFTR inhibitor CFTRinh-172 was able to prolong survival and slow leukemia progression of the transplanted B-ALL model mice.
Patient cases that harbor Philadelphia chromosome (Ph) encoding the BCR-ABL fusion protein whose genetic lesion is a t(9;22) translocation continue to experience poorer prognosis (4,10). In a previous study, we showed that CFTR inhibitor CFTRinh-172 exerted a significant apoptosis-inducing, anti-proliferative and cell cycle-arresting effect on B-ALL Ph+ leukemia SUP-B15 cells (4). We found that high CFTR expression in Ph + acute leukemia cells protected and maintained the continuous activation of BCR-ABL and the canonical Wnt/β-catenin signaling pathway by decreasing PP2A phosphatase activity (4). Moreover, highly expressed CFTR functions in Ph+ leukemia pathogenesis by the HDAC2-mediated PTEN pathway (5). In this study, we further investigated the role of CFTRinh-172 in vivo. The results showed that CFTRinh-172 blocked the leukemia progress in SUP-B15 transplanted mice. In brief, our results suggested that targeting CFTR may have potential in the treatment of leukemia, especially Ph+ with BCR-ABL B-ALL leukemia.
Taken together, our previous works about CFTR on leukemia and significant inhibition of cell proliferation in acute lymphoblastic leukemia in vitro and in vivo by CFTR interference or CFTR inhibitor CFTR-inh172 (4,5) suggest that targeting CFTR as a new gene therapy may have potential in the future.
Acknowledgments
Funding: This research was supported by grants from
Footnote
Reporting Checklist: The authors have completed the ARRIVE reporting checklist. Available at https://tcr.amegroups.com/article/view/10.21037/tcr-21-2296/rc
Data Sharing Statement: Available at https://tcr.amegroups.com/article/view/10.21037/tcr-21-2296/dss
Peer Review File: Available at https://tcr.amegroups.com/article/view/10.21037/tcr-21-2296/prf
Conflicts of Interest: All authors have completed the ICMJE uniform disclosure form (available at https://tcr.amegroups.com/article/view/10.21037/tcr-21-2296/coif). The authors have no conflicts of interest to declare.
Ethical Statement:
Open Access Statement: This is an Open Access article distributed in accordance with the Creative Commons Attribution-NonCommercial-NoDerivs 4.0 International License (CC BY-NC-ND 4.0), which permits the non-commercial replication and distribution of the article with the strict proviso that no changes or edits are made and the original work is properly cited (including links to both the formal publication through the relevant DOI and the license). See: https://creativecommons.org/licenses/by-nc-nd/4.0/.
References
- Gadsby DC, Vergani P, Csanády L. The ABC protein turned chloride channel whose failure causes cystic fibrosis. Nature 2006;440:477-83. [Crossref] [PubMed]
- Moran O. The gating of the CFTR channel. Cell Mol Life Sci 2017;74:85-92. [Crossref] [PubMed]
- Sun H, Wang Y, Zhang J, et al. CFTR mutation enhances Dishevelled degradation and results in impairment of Wnt-dependent hematopoiesis. Cell Death Dis 2018;9:275. [Crossref] [PubMed]
- Yang X, Yan T, Gong Y, et al. High CFTR expression in Philadelphia chromosome-positive acute leukemia protects and maintains continuous activation of BCR-ABL and related signaling pathways in combination with PP2A. Oncotarget 2017;8:24437-48. [Crossref] [PubMed]
- Yan T, Leng Y, Yang X, et al. High-expressing cystic fibrosis transmembrane conductance regulator interacts with histone deacetylase 2 to promote the development of Ph+ leukemia through the HDAC2-mediated PTEN pathway. Leuk Res 2017;57:9-19. [Crossref] [PubMed]
- Wartenberg D, Groves FD, Adelman AS. Acute Lymphoblastic Leukemia: Epidemiology and Etiology. Acute Leukemias. Berlin Heidelberg: Springer 2008:77-93.
- Yu H, Du Y, Xu J, et al. Prognostic relevance of genetic variations in T-cell acute lymphoblastic leukemia/lymphoblastic lymphoma. Transl Cancer Res 2019;8:2485-95. [Crossref] [PubMed]
- Inaba H, Mullighan CG. Pediatric acute lymphoblastic leukemia. Haematologica 2020;105:2524-39. [Crossref] [PubMed]
- Wynne J, Wright D, Stock W. Inotuzumab: from preclinical development to success in B-cell acute lymphoblastic leukemia. Blood Adv 2019;3:96-104. [Crossref] [PubMed]
- El Fakih R, Jabbour E, Ravandi F, et al. Current paradigms in the management of Philadelphia chromosome positive acute lymphoblastic leukemia in adults. Am J Hematol 2018;93:286-95. [Crossref] [PubMed]
- Loghavi S, Kutok JL, Jorgensen JL. B-acute lymphoblastic leukemia/lymphoblastic lymphoma. Am J Clin Pathol 2015;144:393-410. [Crossref] [PubMed]
- Guo Y, Shan Q, Gong Y, et al. Oridonin in combination with imatinib exerts synergetic anti-leukemia effect in Ph+ acute lymphoblastic leukemia cells in vitro by inhibiting activation of LYN/mTOR signaling pathway. Cancer Biol Ther 2012;13:1244-54. [Crossref] [PubMed]
- Khan NI, Bradstock KF, Bendall LJ. Activation of Wnt/beta-catenin pathway mediates growth and survival in B-cell progenitor acute lymphoblastic leukaemia. Br J Haematol 2007;138:338-48. [Crossref] [PubMed]
- Luis TC, Ichii M, Brugman MH, et al. Wnt signaling strength regulates normal hematopoiesis and its deregulation is involved in leukemia development. Leukemia 2012;26:414-21. [Crossref] [PubMed]