Mechanical property evaluation of the right ventricular myocardium in cancer patients with chemotherapy by echocardiography: a systematic review and meta-analysis
Introduction
With the development of modern cancer treatment, the mortality rate of cancer patients has significantly decreased (1,2). However, cancer therapy-related cardiotoxicity remains as the leading cause of morbidity and mortality in cancer survivors (3,4), and cardiovascular complications caused by cancer therapy have become the second largest threat to the long-term survival of patients (5). As a result, for patients with cancer, contemporary management should focus on the identification of potential cardiotoxicity during anticancer therapy for early detection and intervention.
Cardiovascular side effects caused by chemotherapy or radiotherapy mainly include myocardial dysfunction, heart failure, myocardial ischemia, arrhythmia, arterial hypertension, and pulmonary hypertension, among which myocardial dysfunction and heart failure are the most common cardiotoxicities (6). According to the type and the intensity of the anticancer regimen, the risk and the degree of cardiotoxicity are different. Common chemotherapeutic drugs that cause cardiotoxicity include anthracycline and fluorouracil, as well as, targeted agents such as human epidermal growth factor 2 (HER-2) inhibitors and angiogenesis inhibitors. Most cardiotoxicity caused by anthracyclines is type I, which involves myocyte death, as well as, irreversible and permanent myocardial injury in a dose-dependent manner (7), while trastuzumab, an HER-2 inhibitor, mostly leads to type II treatment-related cardiac damage, whose effects are reversible in a dose-independent manner and without the induction of significant ultrastructural changes (7).
Position papers and recommendations on the prevention and treatment of cancer therapy-related cardiotoxicity refer mainly to the left ventricle. The guidelines recommend measuring the left ventricular ejection fraction (LVEF) to monitor the occurrence of cardiotoxicity, defining cancer therapy-related cardiotoxicity by the left ventricular index, and using the left ventricular global longitudinal strain (LVGLS) to monitor myocardial function (8-10). The importance of right ventricular structure and function in a variety of cardiovascular diseases, such as heart failure, coronary artery disease, valvular disease, pulmonary hypertension, and hypertrophic cardiomyopathy, has been confirmed (11-15), and greater attention has been paid to the inclusion of right ventricular assessment in cancer patients who have underwent chemotherapy and radiotherapy.
An increasing number of studies have begun to evaluate changes of the right ventricle (RV). Some studies have reported that RV function decreases early, even before the derangement of LV function, while other studies have not identified significant statistical changes in RV contraction. Furthermore, several indexes can evaluate right ventricular contraction, and different studies have reported changes in different parameters at variable degrees. Thus, a meta-analysis of these studies was performed to objectively evaluate the mechanical properties of the right ventricular myocardium by echocardiography in cancer patients who have underwent chemotherapy. We present the following analysis in accordance with the Preferred Reporting Items for Systematic Reviews and Meta-Analyses (PRISMA) reporting checklist (available at https://tcr.amegroups.com/article/view/10.21037/tcr-21-2324/rc).
Methods
Searching strategies and selection criteria
This systematic review and meta-analysis was reported in accordance with the PRISMA Statement (16) and was registered at the International Prospective Register of Systematic Reviews (No. CRD42021284129). Selected studies were published before August 11, 2021 by application of Embase, PubMed, and CENTRAL (Cochrane Central of Controlled Trials) databases. A manual search was adopted as well. The combined texts and MeSH terms were used during the searches as follows: “right ventricle” “cardiotoxicity” “chemotherapy”, and “deformation”. The complete search terms used for PubMed were: (chemotherapy[MeSH Terms] OR chemotherap* OR trastuzumab OR pertuzumab OR trastuzumab emtansine OR lapatinib OR neratinib OR anthracycline OR doxorubicin OR adriamycin OR idarubicin OR epirubicin OR daunorubicin OR mitoxantrone OR 5-fluorouracil OR paclitaxel OR cyclophosphamide) AND (cardiotoxicity[MeSH Terms] OR heart failure[MeSH Terms] OR ventricular dysfunction[MeSH Terms] OR cardiotox* OR heart failure OR ventricular dysfunction) AND (right ventricle OR heart right ventricle wall) AND (deformation OR strain OR global longitudinal strain). All potentially eligible studies were selected for reviewing irrespective of primary outcomes or languages.
Study selection and evaluated outcomes
Studies were regarded as eligible for enrollment if they were performed in cancer patients who underwent chemotherapy and reported right ventricular global longitudinal strain (RVGLS), right ventricular free wall longitudinal strain (RVFWLS), tricuspid annular plane systolic excursion (TAPSE), systolic velocity of tricuspid annulus (S'), right ventricular fractional area change (RVFAC), and pulmonary artery systolic pressure (PASP) by echocardiography at baseline and follow-up. Studies were excluded if they did not adhere to the aforementioned criteria. Ethical approval was not required as this was a systematic review and meta-analysis.
We compared the mechanical properties of the right ventricular myocardium in cancer patients who underwent chemotherapy at baseline and follow-up, without restrictions on treatment history. The evaluated outcomes were as follows: RVGLS, RVFWLS, TAPSE, S', and PASP, which were measured by echocardiography at baseline, at the end of several cycles, or several months after the completion of the entire regimen. The RVFAC was calculated by measuring the end-diastolic and end-systolic area of the RV.
Data extraction and assessment
Titles and abstracts from different studies were reviewed by two independent investigators. Studies qualified for inclusion were retrieved, and the full text was reviewed. Trials selected for detailed analysis and data extraction were analyzed by two independent investigators, and disagreements were evaluated and resolved by a third investigator. The following data were extracted from the selected studies: basic characteristics of the study (author, published year, sample size, and ultrasound system), features of the included patients (average age, gender, weight, height, body mass index, body surface index, heart rate, blood pressure, malignancy history, comorbidities, cumulative dose of drugs, received radiotherapy or not), and the outcomes including RVGLS, RVFWLS, TAPSE, S', PASP and RVFAC at baseline and follow-up.
Two independent reviewers assessed risk of bias according to PRISMA recommendations. The quality of the included studies was assessed using the validated Newcastle-Ottawa Scale, which evaluates nonrandomized observational studies recommended by the nonrandomized research methodology group of Cochrane Collaboration. The Newcastle-Ottawa Scale evaluated the quality of the enrolled articles from three dimensions, including selection, comparability, and outcome, with a full score of nine stars.
Statistical analysis
The mechanical properties of the right ventricular myocardium in cancer patients who underwent chemotherapy were assessed based on six parameters as follows: RVGLS, RVFWLS, TAPSE, S', PASP, and RVFAC, which were measured by echocardiography. These indexes were regarded as continuous variables and reported absolute differences between arithmetic means before and after chemotherapy. Pooled estimates of the standardized mean differences of these indexes were calculated using a random-effects model. Meanwhile, pre-planned sensitivity analysis was performed before formal meta-analysis of TAPSE, S', RVFWLS and RVGLS. The possibility of publication biases of these parameters was also assessed utilizing the Egger test and the Begg test, and P values <0.05 were taken as significant publication bias. The Cochran Q test was performed to assess heterogeneity between studies, and P values <0.05 were taken as significant heterogeneity. The I2 test was also adopted to assess the magnitude of the heterogeneity, and values greater than 50% were taken as moderate-to-high heterogeneity. In cases of obvious heterogeneity, Stata software was used for sensitivity analysis of the impact of a single study on the overall study. Stata version 16.0 software (StataCorp LP, College Station, TX, USA) was used for all statistical analysis.
Results
Characteristics of included trials
Two hundred and fifty-nine studies were identified from databases and manual search after excluding duplicated studies. Finally, twenty-one trials published between 2013 to 2021, including 1,355 participants, were adopted in our analysis (17-37). The detailed process of literature searching and the explanation for exclusion are described in Figure 1.
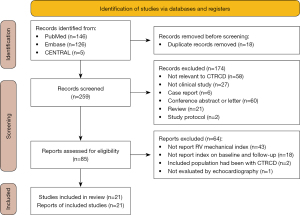
The characteristics of these studies included study information, and the demographic characteristics are summarized in Tables 1,2. Among the included studies, all patients received chemotherapy, and in five studies, patients received radiotherapy. Most studies provided percentages or numbers pertaining to the mean age (ranged from 11.85 to 60 years), male ratio (ranged from 0% to 65.6%), mean cumulative dose (Anthracycline ranged from 175 to 483.0 mg/m2), mean body mass index (ranged from 25.1 to 27.9 kg/m2), and ratio of smokers (ranged from 10% to 68.7%). Some patients presented comorbidities, such as hypertension (the ratio ranged from 11.8% to 77.14%), diabetes mellitus (the ratio range from 1.6 % to 54.2%), and hyperlipidemia (the ratio ranged from 6.7% to 51.4%). The quality of the enrolled articles evaluated by the Newcastle-Ottawa Scale was medium to high, with a total score of 6–9 (Table 3). The main confounding factors included medications and heart disease history before chemotherapy, which may have affected the outcomes. These indexes were used to evaluate the mechanical properties of the right ventricular myocardium reported in most studies during the different stages of chemotherapy. Notably, strain of the right ventricular myocardium was reported in seventeen studies.
Table 1
Study | Region | Sample | Mean age, y | Male, n [%] | Cumulative dose (mg/m2) | Malignancy history, n [%] | Radiotherapy, n [%] | Ultrasonic system |
---|---|---|---|---|---|---|---|---|
Zhao 2020 | Shanghai, China | 74 | 48.9±11.8 | 41 [55] | Anthracycline 358.20±69.04 | DLBCL | Without | iE33, Philips Medical Systems (Andover Massachusetts) |
Wang 2018 | Nanjing, China | 36 | 34±7 | 0 [0] | Anthracycline – | Breast cancer | Without | Toshiba Artida SSH-880CV (Japan) |
Wang 2021 | Cangzhou, China | 61 | 50.8±12.1 | 29 [47.5] | Anthracycline 311.2±99.8 | DLBCL | Without | iE33, Philips Medical Systems (Bothell, WA, USA) |
Song 2017 | Shanghai, China | 89 | 49.3±12.5 | 60 [59] | Anthracycline 358.20±69.03 | DLBCL | Without | iE33, Philips Medical Systems (Andover WA, USA) |
Planek 2020 | USA | 35 | 50.6±13.2 | 21 [60] | Anthracycline 239±104 | Lymphoma | Without | Vivid 7, GE Vingmed Ultrasound AS (Horten, Norway) |
Paraskevaidis 2017 | Greece | 80 | 45±11 | 44 [55] | – | NHL 40 [50]; AML 32 [40]; CML 8 [10] | Without | Vivid 7, GE Vingmed Ultrasound (Horten, Norway) |
Moustafa 2016 | USA | 50 | 60±13 | 0 [0] | Anthracycline ≤240 | Breast cancer | 31 [62] | VVI system, Siemens Medical Solutions USA (Malvern, PA, USA) |
Keramida 2019 | USA | 101 | 54.3±11.4 | 0 [0] | Anthracycline 483.0±145.1 | Breast cancer | 55 [54.4] | Vivid E9, GE Vingmed Ultrasound; iE33, Philips Medical Systems |
Boczar 2016 | Canada | 49 | 53.4±3.3 | 1 [2] | Doxorubicin 232±30.99; Epirubicin 294.12±23.90 | Breast cancer | Without | TomTec Imaging Systems (Unterschleissheim, Germany) |
Cherata 2019 | Romania | 68 | 55±14 | 35 [51] | – | HL 9 [13.2]; NHL 20 [29.4]; MM 17 [25]; AML 10 [14.7]; ALL 7 [10.3]; others 5 [7.4] | Without | Vivid E9 GE Healthcare (Horten, Norway); iE33, Philips Ultrasound |
Chen 2019 | Shanghai, China | 128 | 60±19 | 84 [65.6] | – | NSCLC: squamous 34 [26.6]; non-squamous 4 [73.4] | 128 [100] | Vivid 7 or E9, GE Vingmed Ultrasound (Horten, Norway) |
Chang 2016 | Taiwan, China | 35 | 45.33±8.48 | 0 [0] | Anthracycline 354.19±336.08 | Breast cancer | 2 [5.71] | Vivid E9, GE Vingmed Ultrasound AS (Horten, Norway) |
Arciniegas Calle 2018 | USA | 66 | 52±9 | 0 [0] | Anthracycline 252±45; trastuzumab 5.4±2.7 g | Breast cancer | 50 [76] | GE Vivid 7 system (General Electric Company) |
Anqi 2019 | China | 36 | 47.27±9.90 | 0 [0] | Anthracycline 363.90±107.77 | Breast cancer | Without | EPIQ7C, Philips Medical Systems |
Mornoş 2014 | Romania | 59 | 51±10 | 24 [40.7] | Anthracycline 175±62 | NHL 12 [20.3]; HL 10 [16.9]; ALL 8 [13.6]; AML 2 [3.4]; breast cancer 26 [44.1]; osteosarcoma 1 [1.7] | Without | Vivid 9 General Electric (Milwaukee, WI); version 6.0, GE Healthcare (UK) |
Lange 2016 | Germany | 27 | 56 [38–75] | 0 | – | Breast cancer | Without | – |
Xu 2019 | Shanghai, China | 60 | 52±14 | 35 [58.3] | Anthracycline 238±21.8 | DLBCL | Without | iE33,Philips Medical Systems (Andover, WA, USA) |
Wang 2020 | Nanjing, China | 32 | 33.2±8.3 | 0 | – | Breast cancer | Without | Artida SSH-880CV (Toshiba, Japan) |
Mornos 2013 | Romania | 74 | 51±11 | 31 [41.9] | Anthracycline 259±52 | NHL 15 [20.3]; HL 13 [17.6]; ALL 9 [12.1]; AML 2 [2.7]; breast cancer 33 [44.6]; osteosarcoma 2 [2.7] | Without | Vivid 7 GE, Milwaukee (Wisconsin, USA); version 6.0, GE Healthcare (UK) |
Khairat 2019 | Egypt | 100 | 11.85±2.09 | 50 [50.0] | Anthracycline 450 | Osteosarcoma | Without | Vivid 7 or E9 GE Vingmed Ultrasound (Horten, Norway) |
Xu 2021 | Nanjing, China | 95 | 53.2±8.7 | 0 | Anthracycline 360 | Breast cancer | Without | GE Vivid E9 ultrasound machine (Horten, Norway) |
DLBCL, diffuse large B-cell lymphoma; NHL, non-Hodgkin’s lymphoma; HL, Hodgkin’s lymphoma; AML, acute myeloid leukemia; CML, chronic myeloid leukemia; ALL, acute lymphoblastic leukemia; MM, multiple myeloma; NSCLC, non-small cell lung cancer.
Table 2
Study | Height (cm) | Weight (kg) | BMI (kg/m2) | BSA (m2) | HR (bpm) | SBP (mmHg) | DBP (mmHg) | Smoker, n [%] | Hyper-tension, n [%] | Diabetes mellitus, n [%] | Hyper-lipidemia, n [%] |
---|---|---|---|---|---|---|---|---|---|---|---|
Zhao 2020 | 166.9±6.1 | 65.6±11.6 | – | – | 78.6±11.9 | 117.4±9.6 | 75.2±7.4 | 20 [27] | – | 5 [7] | – |
Wang 2018 | – | – | – | – | – | – | – | – | – | – | – |
Wang 2021 | – | – | 26.6±5.5 | 1.73±0.17 | 65.9±10.1 | 125.1±15.7 | 77.2±8.6 | 18 [29.5] | 20 [32.8] | 9 [14.8] | – |
Song 2017 | 166.9±6.1 | 65.6±11.6 | – | 1.7±0.1 | 78.6±11.9 | 117.4±9.6 | 75.2±7.4 | – | – | – | – |
Planek 2020 | – | – | 27.9±5.6 | – | 89.1±14.2 | – | – | 13 [37.1] | 27 [77.14] | 19 [54.2] | 18 [51.4] |
Paraskevaidis 2017 | – | – | – | – | 77±12 | 125±6 | 76±6 | 16 [20] | 12 [15] | – | 8 [10] |
Moustafa 2016 | – | – | 26.5±5.2 | – | 71±11 | – | – | 5 [10] | 14 [28] | 8 [16] | 10 [20] |
Keramida 2019 | – | – | – | 1.8±0.2 | – | – | – | – | 15 [14.8] | – | – |
Boczar 2016 | – | – | 25.9±1.3 | – | 83.0±31.3 | – | – | 21 [42.9] | 12 [24.5] | 7 [14.3] | 7 [14.3] |
Cherata 2019 | – | – | – | 1.84±2.14 | 79±13 | 127±17 | 75±12 | 9 [13] | 11 [16] | 3 [4.4] | – |
Chen 2019 | – | – | – | – | – | – | – | 88 [68.7] | – | – | – |
Chang 2016 | 157.57±5.74 | 56.88±6.86 | – | 1.63±0.21 | 73.5±8.24 | 129.66±14.01 | 82.66±7.37 | – | – | – | |
Arciniegas Calle 2018 | – | – | – | – | 71±12 | – | – | 13 [20] | 13 [20] | 3 [5] | 12 [18] |
Anqi 2019 | 1.60±0.43 | 65.41±9.15 | 25.45±3.44 | 1.66±0.13 | 83.92±9.86 | 131.42±16.75 | 76.65±9.85 | – | 10 [27.78] | – | – |
Mornoş 2014 | – | – | 25.1±3.8 | – | 73±10 | 121±17 | 76±9 | 10 [16.9] | 7 [11.8] | 1 [1.6] | 4 [6.7] |
Lange 2016 | – | – | 26.26±3.74 | – | – | – | – | – | – | – | – |
Xu 2019 | – | 63±14 | – | – | 80.6±11.6 | – | – | – | – | – | – |
Wang 2020 | – | – | – | – | – | – | – | – | – | – | – |
Mornos 2013 | – | – | 25.3±3.7 | – | 72±10 | 120±16 | 75±9 | 15 [20.2] | 11 [14.8] | 3 [4.0] | 8 [10.8] |
Khairat 2019 | – | – | – | 1.39±0.07 | – | – | – | – | – | – | – |
Xu 2021 | – | – | 25.5±3.4 | 1.6±0.1 | 70.8±8.0 | 113.2±9.9 | 71.4±6.2 | – | – | – | – |
BMI, body mass index; BSA, body surface index; HR, heart rate; bpm, beat per minute; SBP, systolic blood pressure; DBP, diastolic blood pressure.
Table 3
Study | Selection | Comparability | Outcome | Total score | |||||||
---|---|---|---|---|---|---|---|---|---|---|---|
Representativeness of the exposed cohort | Selection of the nonexposed cohort | Ascertainment of exposure | Demonstration that outcome of interest was not present at start of study | Comparability of cohorts on the basis of the design or analysis | Assessment of outcome | Was follow-up long enough for outcomes to occur | Adequacy of follow up of cohorts | ||||
Zhao 2020 | * | * | * | * | ** | * | * | 8 | |||
Wang 2018 | * | * | * | * | * | * | 6 | ||||
Wang 2021 | * | * | * | * | ** | * | * | * | 9 | ||
Song 2017 | * | * | * | * | * | * | * | 7 | |||
Planek 2020 | * | * | * | * | ** | * | * | * | 9 | ||
Paraskevaidis 2017 | * | * | * | * | ** | * | * | * | 9 | ||
Moustafa 2016 | * | * | * | * | * | * | * | * | 8 | ||
Keramida 2019 | * | * | * | * | * | * | * | * | 8 | ||
Boczar 2016 | * | * | * | * | ** | * | * | * | 9 | ||
Cherata 2019 | * | * | * | * | * | * | * | * | 8 | ||
Chen 2019 | * | * | * | * | * | * | * | 7 | |||
Chang 2016 | * | * | * | * | * | * | * | * | 8 | ||
Arciniegas Calle 2018 | * | * | * | * | * | * | * | * | 8 | ||
Anqi 2019 | * | * | * | * | ** | * | * | 8 | |||
Mornoş 2014 | * | * | * | * | ** | * | * | * | 9 | ||
Lange 2016 | * | * | * | * | * | * | * | 7 | |||
Xu 2019 | * | * | * | * | * | * | 6 | ||||
Wang 2020 | * | * | * | * | * | * | 6 | ||||
Mornos 2013 | * | * | * | * | ** | * | * | 8 | |||
Khairat 2019 | * | * | * | * | * | * | * | 7 | |||
Xu 2021 | * | * | * | * | * | * | * | * | 8 |
*, give asterisks according to the description of the Newcastle-Ottawa Scale, a higher total score indicates that the quality of the study is better. (Note: A study can be awarded a maximum of one star for each numbered item within the Selection and Outcome categories. A maximum of two stars can be given for Comparability).
Changes of mechanical properties in patients without radiotherapy
Sixteen articles reported changes in the mechanical properties of the RV during the different stages of chemotherapy. This cohort comprised of 975 cancer patients who underwent chemotherapy but not radiotherapy.
PASP increased [standardized mean difference (SMD) =0.161, 95% CI: 0.007 to 0.316, P=0.040, Figure 2] compared to the condition at baseline. An analysis of this outcome showed a prominent heterogeneity between the studies (P value of Q test =0.005, I2=58.8%). The Egger or Begg test demonstrated that there was no evident publication bias (P value of Egger test =0.295, P value of Begg test =0.193).
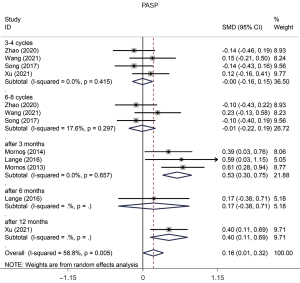
The results of TAPSE assessed in a pooled analysis indicated there was a reduction in patients who underwent chemotherapy (SMD =−0.543, 95% CI: −0.698 to −0.389, P=0.000, Figure 3) compared to the condition before chemotherapy, and there was significant heterogeneity between each study (P value of Q test =0.000, I2=81.4%). The Egger or Begg test demonstrated evident publication bias in this study (P value of Egger test =0.000, P value of Begg test =0.004). Moreover, all subgroups showed a significant reduction with no publication bias, except the subgroup of “1–2 Cycles”, and TAPSE showed a reduction at the stage of “6–8 Cycles” without heterogeneity between studies (Table 4).
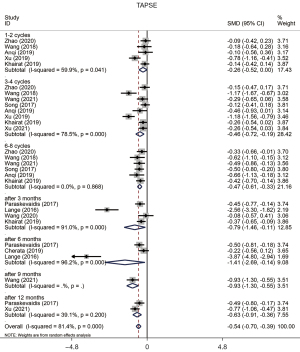
Table 4
Variables | SMD | 95% CI | I2 (%) | Cochran Q (P) | Z | P | Model | Begg test | Egger test |
---|---|---|---|---|---|---|---|---|---|
PASP | |||||||||
Overall | 0.161 | (0.007, 0.316) | 58.8 | 0.005 | 2.05 | 0.040 | Random | 0.193 | 0.295 |
3–4 Cycles | −0.004 | (−0.160, 0.151) | 0.0 | 0.415 | 0.06 | 0.955 | Random | 1.000 | 0.835 |
6–8 Cycles | −0.011 | (−0.215, 0.194) | 17.6 | 0.297 | 0.10 | 0.920 | Random | 0.296 | 0.322 |
After 3 months | 0.526 | (0.302, 0.750) | 0.0 | 0.657 | 4.61 | 0.000 | Random | 1.000 | 0.954 |
TAPSE | |||||||||
Overall | −0.543 | (−0.698, −0.389) | 81.4 | 0.000 | 6.90 | 0.000 | Random | 0.004 | 0.000 |
1–2 Cycles | −0.258 | (−0.519, 0.002) | 59.9 | 0.041 | 1.94 | 0.052 | Random | 0.462 | 0.798 |
3–4 Cycles | −0.455 | (−0.720, −0.191) | 78.5 | 0.000 | 3.37 | 0.001 | Random | 0.063 | 0.045 |
6–8 Cycles | −0.472 | (−0.613, −0.331) | 0.0 | 0.868 | 6.54 | 0.000 | Random | 0.133 | 0.084 |
After 3 months | −0.787 | (−1.463, −0.111) | 91.0 | 0.000 | 2.28 | 0.022 | Random | 0.734 | 0.305 |
After 6 months | −1.414 | (−2.692, −0.136) | 96.2 | 0.000 | 2.17 | 0.030 | Random | 1.000 | 0.153 |
S' | |||||||||
Overall | −0.507 | (−0.748, −0.266) | 89.5 | 0.000 | 4.13 | 0.000 | Random | 0.369 | 0.035 |
1–2 Cycles | 0.050 | (−0.180, 0.279) | 0.0 | 0.789 | 0.43 | 0.670 | Random | 1.000 | 0.846 |
3–4 Cycles | −0.219 | (−0.399, −0.039) | 36.2 | 0.166 | 2.38 | 0.017 | Random | 0.452 | 0.291 |
6–8 Cycles | −0.362 | (−0.669, −0.056) | 70.1 | 0.010 | 2.32 | 0.020 | Random | 0.806 | 0.737 |
After 3 months | −1.514 | (−2.865, −0.162) | 97.1 | 0.000 | 2.19 | 0.028 | Random | 0.308 | 0.360 |
RVGLS | |||||||||
Overall | 1.017 | (0.751, 1.283) | 91.3 | 0.000 | 7.49 | 0.000 | Random | 0.055 | 0.002 |
1–2 Cycles | 0.677 | (0.266, 1.088) | 67.0 | 0.048 | 3.23 | 0.001 | Random | 1.000 | 0.850 |
3–4 Cycles | 1.124 | (0.438, 1.809) | 95.2 | 0.000 | 3.21 | 0.001 | Random | 0.707 | 0.074 |
6–8 Cycles | 1.625 | (0.696, 2.554) | 96.2 | 0.000 | 3.43 | 0.001 | Random | 0.806 | 0.186 |
After 3 months | 0.961 | (0.498, 1.424) | 78.5 | 0.009 | 4.07 | 0.000 | Random | 0.296 | 0.078 |
SMD, standardized mean difference; PASP, pulmonary artery systolic pressure; TAPSE, tricuspid annular plane systolic excursion; S', systolic velocity of tricuspid annulus; RVGLS, right ventricular global longitudinal strain.
Combined data from these studies implied that the mechanical property of the RV evaluated by S' exhibited a reduction after chemotherapy (SMD =−0.507, 95% CI: −0.748 to −0.266, P=0.000, Figure 4), and a statistically significant heterogeneity was observed (P value of Q test =0.000, I2=89.5%). We found that the data from Lange’s study had an impact on the overall result, namely, in the sensitivity analysis of TAPSE and S'. We speculate that the small sample size of this trial may have affected the accuracy of the results. The Begg test illustrated that no evident publication bias was found in this study (P value of Begg test =0.369). Furthermore, this significant reduction of S' was observed in a late stage of treatment without publication bias (Table 4).
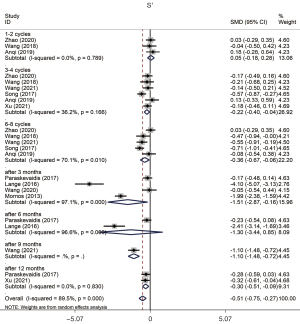
We used free wall longitudinal strain (FWLS) and global longitudinal strain (GLS), whose values were negative, to evaluate the change of strain of the RV. Our study demonstrated a significant decrease of RVFWLS (SMD =0.833, 95% CI: 0.549 to 1.118, P=0.000, Figure 5) and RVGLS (SMD =1.017, 95% CI: 0.751 to 1.283, P=0.000, Figure 6) with heterogeneity between studies (P value of Q test =0.000, I2=91.3%). The sensitivity analysis of right ventricular strain showed that the results reported by Wang had an effect on the overall study. The subjects of this study were mainly young patients, and the observation period was relatively short, which might have contributed to the limitations of the test results. The Begg test showed that there was no evident publication bias in this analysis (P value of Begg test =0.055). All subgroups showed an evident reduction of strain without publication bias (Table 4).
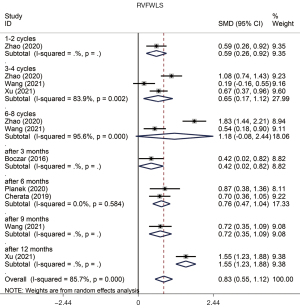
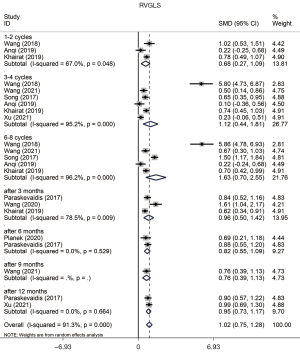
There was no significant difference in RVFAC (SMD =−0.097, 95% CI: −0.213 to 0.018, P=0.099) with significant heterogeneity between these studies (P value of Q test =0.000, I2=68.8%).
Changes in the mechanical properties in all patients who underwent chemotherapy
We further explored the changes in the mechanical properties of the RV in cancer patients who underwent chemotherapy and radiotherapy. This analysis included PASP, TAPSE, S', RVFWLS, and RVGLS (Table 5). The results of PASP assessed in this analysis indicated there was an increase in cancer patients who underwent chemotherapy or radiotherapy (SMD =0.175, 95% CI: 0.027 to 0.324, P=0.020). The pooled analysis of TAPSE showed a significant reduction in the mechanical properties of the RV after chemotherapy (SMD =−0.475, 95% CI: −0.606 to −0.345, P=0.000) compared with the baseline, and this phenomenon was observed in every stage of treatment. Our study also demonstrated a reduction of S' (SMD =−0.401, 95% CI: −0.580 to −0.222, P=0.000) and RVFWLS (SMD =0.702, 95% CI: 0.482 to 0.923, P=0.000). Notably, almost in every subgroup, we found an evident reduction of right ventricular strain, especially GLS (SMD =0.853, 95% CI: 0.655 to 1.052, P=0.000).
Table 5
Variables | SMD | 95% CI | I2 (%) | Cochran Q (P) | Z | P | Model | Begg test | Egger test |
---|---|---|---|---|---|---|---|---|---|
PASP | |||||||||
Overall | 0.175 | (0.027, 0.324) | 56.9 | 0.006 | 2.32 | 0.020 | Random | 0.161 | 0.202 |
3–4 Cycles | 0.044 | (−0.131, 0.219) | 27.1 | 0.241 | 0.49 | 0.623 | Random | 0.462 | 0.216 |
6–8 Cycles | −0.011 | (−0.215, 0.194) | 17.6 | 0.297 | 0.10 | 0.920 | Random | 0.296 | 0.322 |
After 3 months | 0.526 | (0.302, 0.750) | 0.0 | 0.657 | 4.61 | 0.000 | Random | 1.000 | 0.954 |
TAPSE | |||||||||
Overall | −0.475 | (−0.606, −0.345) | 81.1 | 0.000 | 7.15 | 0.000 | Random | 0.000 | 0.000 |
1–2 Cycles | −0.317 | (−0.567, −0.066) | 60.9 | 0.026 | 2.48 | 0.013 | Random | 0.260 | 0.486 |
3–4 Cycles | −0.542 | (−0.824, −0.259) | 82.0 | 0.000 | 3.76 | 0.000 | Random | 0.029 | 0.011 |
6–8 Cycles | −0.472 | (−0.613, −0.331) | 0.0 | 0.868 | 6.54 | 0.000 | Random | 0.133 | 0.084 |
After 3 months | −0.602 | (−1.133, −0.070) | 90.5 | 0.000 | 2.22 | 0.026 | Random | 0.221 | 0.159 |
After 6 months | −0.642 | (−1.137, −0.148) | 91.9 | 0.000 | 2.55 | 0.011 | Random | 0.260 | 0.016 |
After 12 months | −0.378 | (−0.747, −0.010) | 81.7 | 0.001 | 2.01 | 0.044 | Random | 1.000 | 0.837 |
S' | |||||||||
Overall | −0.401 | (−0.580, −0.222) | 87.5 | 0.000 | 4.39 | 0.000 | Random | 0.019 | 0.002 |
1–2 Cycles | −0.120 | (−0.475, 0.234) | 63.7 | 0.041 | 0.67 | 0.506 | Random | 0.734 | 0.573 |
3–4 Cycles | −0.235 | (−0.395, −0.075) | 25.9 | 0.231 | 2.89 | 0.004 | Random | 1.000 | 0.448 |
6–8 Cycles | −0.362 | (−0.669, −0.056) | 70.1 | 0.010 | 2.32 | 0.020 | Random | 0.806 | 0.737 |
After 3 months | −1.170 | (−2.193, −0.147) | 96.9 | 0.000 | 2.24 | 0.025 | Random | 0.221 | 0.174 |
After 6 months | −0.496 | (−1.002, 0.011) | 91.0 | 0.000 | 1.92 | 0.055 | Random | 0.086 | 0.019 |
After 9 months | −0.506 | (−1.161, 0.149) | 90.6 | 0.000 | 1.51 | 0.130 | Random | 1.000 | 0.403 |
After 12 months | −0.161 | (−0.436, 0.114) | 62.7 | 0.068 | 1.15 | 0.251 | Random | 1.000 | 0.542 |
RVFWLS | |||||||||
Overall | 0.702 | (0.482, 0.923) | 87.1 | 0.000 | 6.24 | 0.000 | Random | 0.049 | 0.181 |
3–4 Cycles | 0.703 | (0.322, 1.085) | 77.4 | 0.004 | 3.62 | 0.000 | Random | 1.000 | 0.841 |
After 6 months | 0.729 | (0.382, 1.075) | 77.4 | 0.004 | 4.12 | 0.000 | Random | 1.000 | 0.952 |
RVGLS | |||||||||
Overall | 0.853 | (0.655, 1.052) | 90.0 | 0.000 | 8.42 | 0.000 | Random | 0.038 | 0.001 |
1–2 Cycles | 0.677 | (0.266, 1.088) | 67.0 | 0.048 | 3.23 | 0.001 | Random | 1.000 | 0.850 |
3–4 Cycles | 1.124 | (0.438, 1.809) | 95.2 | 0.000 | 3.21 | 0.001 | Random | 0.707 | 0.074 |
6–8 Cycles | 1.625 | (0.696, 2.554) | 96.2 | 0.000 | 3.43 | 0.001 | Random | 0.806 | 0.186 |
After 3 months | 0.771 | (0.376, 1.165) | 85.1 | 0.000 | 3.82 | 0.000 | Random | 0.086 | 0.065 |
After 6 months | 0.739 | (0.459, 1.018) | 75.6 | 0.001 | 5.18 | 0.000 | Random | 0.707 | 0.649 |
After 12 months | 0.665 | (0.311, 1.019) | 79.4 | 0.002 | 3.68 | 0.000 | Random | 1.000 | 0.871 |
SMD, standardized mean difference; PASP, pulmonary artery systolic pressure; TAPSE, tricuspid annular plane systolic excursion; S', systolic velocity of tricuspid annulus; RVGLS, right ventricular global longitudinal strain; RVFWLS, right ventricular free wall longitudinal strain.
Discussion
Cancer therapeutics related cardiac dysfunction (CTRCD)
With improvements in cancer diagnosis and treatment, the incidence of anticancer therapy-related cardiotoxicity and complications of cancer survivors has increased. Modern oncological treatment regimens often incorporate chemotherapy, radiotherapy, and immune checkpoint inhibitors, whose deleterious cardiac effects might be additive or synergistic. Anthracyclines are the most extensively studied chemotherapeutic agents associated with myocardial injury. The complex of anthracycline-Top (topoisomerase) IIβ-DNA is a leading mechanism of irreversible DNA damage and mitochondrial dysfunction in cardiomyocytes, which contributes to myocardial energetic depletion, oxidative stress, and apoptotic cell death (38). Trastuzumab was the first monoclonal antibody to inhibit the receptor tyrosine-protein kinase erbB-2 pathway that was studied in detail for the treatment of cancer. While erbB-2 is present in cardiac myocytes, it is also involved in some pathways which promote cardioprotection that regulate apoptosis, hypertrophic growth, mitotic growth, cell elongation, intercellular adhesion, angiogenesis, and sensitivity to adrenergic signaling. Therefore, an interruption of these cellular pathways may be potentially harmful to the heart (39). Radiation to the chest can damage the heart in a dose-dependent manner. Radiotherapy can cause ischemic damage, heart failure, pericarditis, valvular dysfunction, and arrhythmias, which are a result of radiation-induced activation of acute inflammatory pathways that cause a chronic pathogenic cascade (40). Although the mechanism of myocardial injury caused by radiotherapy and chemotherapy is different, radiotherapy combined with anthracycline can enhance the cardiotoxicity of the agent (6).
Assessment of right ventricular function
In the emerging field of cardio-oncology, experts usually focus on the evaluation of left ventricular function. The guidelines recommend measuring LVEF at baseline, during, and post-therapy to monitor the occurrence of cardiotoxicity, with long-term follow-up of different frequencies based on the clinical predictors of cardiotoxicity risk (8,9). The consensus document of cardiac oncology experts of the American Echocardiography Society (ASE) and the European Association of Cardiovascular Imaging (EACVI) define CTRCD using LVEF and recommend the routine use of LVGLS to monitor myocardial function during treatment (10). The prognostic role of the RV in cardiac diseases has recently emerged (41,42). Presently, right ventricle dysfunction (RVD) has been an area of intense research of cardio-oncology.
In this study, we analyzed the changes in the mechanical properties of the right ventricular myocardium in cancer patients with chemotherapy by echocardiography. Indeed, right ventricular ejection fraction (RVEF), as measured by echocardiography was found to be poorly reliable and not recommended for clinical use or standard reference in research. PASP indirectly reflects the function of the RV. This meta-analysis demonstrated that there was an increase in PASP compared with the condition before chemotherapy and radiotherapy or chemotherapy alone.
The current 2015 recommendations for cardiac chamber quantification of ASE and EACVI (43) recommend that RV systolic function evaluation be performed using conventional parameters, including TAPSE, S', and RVFAC. TAPSE and S', to quantify the displacement and the longitudinal velocity of excursion of the tricuspid annulus, although they may not accurately reflect global RV function because they reflect the function of the basal segment of the RV free wall and assume they are representative of RV global function. Furthermore, they are angle and load-dependent. However, they also have the important advantage of being easily measurable, even in the case of poor image quality and being highly reproducible (44). The pooled analysis also indicated a reduction in TAPSE and S' after chemotherapy, and these results were also observed in patients who underwent chemotherapy and radiotherapy. On the other hand, RVFAC reflects both the longitudinal and the radial components of RV contraction and is angle independent. At the same time, it depends on image quality, requiring complete visualization of the entire RV structure. Furthermore, it was found to have high inter-observer and intra-observer variability when the definition of endocardial was unsatisfactory (44). However, there was no significant difference in RVFAC in this study. TAPSE is limited to longitudinal function and is the first to be altered in RVD. Preliminary evidence suggests that in the first stage of RVD, the systolic pattern of the RV may change, with reduced longitudinal but enhanced transverse contraction (45). Hence, the change of fractional area change (FAC) and TAPSE may be conflicting, with TAPSE being reduced and FAC being enhanced.
Strain is a useful parameter for estimating RV global and regional systolic function. RVGLS is also mainly a reflection of longitudinal contraction, and the term of RVGLS is not limited to analysis of the right ventricular free wall but also the septal segment, which contributes to RV systolic function through biventricular interactions. Sometimes, the term refers to the RV free wall segments only. RV longitudinal strain is less affected by overall cardiac motion (46,47), although it depends on loading conditions, size, and shape of the RV. It is well known that left ventricle positively contributes to right ventricular systolic performance through the shared ventricular septum (48). Therefore, subtle LV contractile dysfunction can reduce the direct contribution of LV contraction to RV function (49,50). This is the reason why RVGLS might be a better indicator of RV function and have a stronger prognostic value than RVFWLS or TAPSE. Furthermore, RVGLS was also superior to commonly used RV function parameters such as RVEF, FAC, TAPSE, and S' for predicting both the cardiovascular mortality and the overall mortality of patients with heart failure (51,52). Strain, as determined by Doppler tissue imaging (DTI), is angle-dependent, while RV speckle-tracking echocardiographic (STE) strain has the disadvantage of being influenced by the image quality, frame rate dependency, and intervendor variability. This analysis demonstrated a reduction in TAPSE and S', such conventional indicators, as well as a significant depression of right ventricular strain, including RVFWLS and RVGLS. It is noteworthy that an evident reduction of strain, especially RVGLS, can be seen at every stage of treatment, while another indicator has not shown changes at the early stage.
All of the above indicators used to assess the mechanical properties of the RV pointed to a deterioration of RV contraction function after anticancer therapy. Chemotherapeutic regimens induced cardiotoxicity displays a regional pattern in which the subendocardial component of the ventricle is most susceptible to toxicity (53). The impaired contractility of the subendocardial layer could be compensated by the relatively normal epicardial layer at an early stage. However, if the toxic effects of chemotherapy persist, then cardiac decompensation and symptomatic heart failure will occur. This might be due to the thinner RV wall and less compensatory reserve, which might lead to the earlier decline in systolic function of RV. The structure of the right ventricular myocardium is composed of three layers, namely, the superficial layer, the middle layer, and the deep layer. In the longitudinal direction, the superficial and deep muscles spiral, so that cardiac contraction moves toward the direction of the ventricular outflow tract, causing the ventricle to move toward the bottom of the heart and the cardiac cavity to become shorter. In coordination with the ventricular septum, the blood is driven from the RV into the pulmonary artery. In the circular direction, the contraction of the middle muscle can narrow the cardiac cavity. Notably, the longitudinal myocardium (the superficial and deep layers) of the RV plays a crucial role in cardiac ejection function (54,55). Basic research has confirmed that the subendocardial longitudinal myocardial fibers are more vulnerable to many factors such as ischemia and poisoning. This may be because chemotherapeutic drugs first contact the subendocardial myocardium, which suffers a relatively large blood flow impact, and the drug concentration of microvessels on the endocardial side is high. The subendocardial myocardium is mainly composed of the longitudinal myocardium, so the longitudinal deformation appeared early and was further aggravated with the accumulation of dose (56).
Limitations
The present study has some limitations. Firstly, the technology of echocardiography associated with some disadvantages. Indeed, the echocardiographic imaging technique requires accurate acquisition of border tracing, while the complex geometry, thin walls, and localization behind the sternum of the RV may bring about considerable challenges in the positioning of the region. Additionally, the included studies are observational studies with comparatively small examples so that potential bias may still exist. The heterogeneity of the outcomes of the different studies can be attributed to several reasons, with differences in baseline cardiotoxicity risk, follow-up timepoints, and chemotherapeutic regimes with or without radiotherapy being the most important. Thirdly, the analysis of some outcomes showed a prominent heterogeneity between the studies, and a reasonable guess is the sample size of the included studies was insufficient. Therefore, the ability of RV functional indexes to predict the incidence of cardiotoxicity and the overall prognosis need to be confirmed in larger multicenter studies with different types of tumors. Meanwhile, a consensus on how to define RV systolic dysfunction ought to be made furtherly to adequately reflect the cancer therapeutics related cardiotoxicity to RV, when evaluating multiple echocardiography parameters together.
Conclusions
Our findings prove that right ventricular contraction function would deteriorate after anticancer therapy in patients, especially with the prolongation of chemotherapy duration and the accumulation of chemotherapeutic drugs. However, further larger multicenter studies are needed to establish the definition of right ventricular systolic dysfunction which can adequately reflect the cancer therapeutics related cardiotoxicity.
Acknowledgments
We would like to thank Editorbar Language Editing (www.editorbar.com) for their help in checking and polishing our paper.
Funding: None.
Footnote
Reporting Checklist: The authors have completed the PRISMA reporting checklist. Available at https://tcr.amegroups.com/article/view/10.21037/tcr-21-2324/rc
Peer Review File: Available at https://tcr.amegroups.com/article/view/10.21037/tcr-21-2324/prf
Conflicts of Interest: All authors have completed the ICMJE uniform disclosure form (available at https://tcr.amegroups.com/article/view/10.21037/tcr-21-2324/coif). The authors have no conflicts of interest to declare.
Ethical Statement: The authors are accountable for all aspects of the work in ensuring that questions related to the accuracy or integrity of any part of the work are appropriately investigated and resolved. This study was based on previously published studies, therefore, ethical approval and patient consent were not required.
Open Access Statement: This is an Open Access article distributed in accordance with the Creative Commons Attribution-NonCommercial-NoDerivs 4.0 International License (CC BY-NC-ND 4.0), which permits the non-commercial replication and distribution of the article with the strict proviso that no changes or edits are made and the original work is properly cited (including links to both the formal publication through the relevant DOI and the license). See: https://creativecommons.org/licenses/by-nc-nd/4.0/.
References
- Lei S, Zheng R, Zhang S, et al. Global patterns of breast cancer incidence and mortality: A population-based cancer registry data analysis from 2000 to 2020. Cancer Commun (Lond) 2021;41:1183-94. [Crossref] [PubMed]
- Chang HM, Moudgil R, Scarabelli T, et al. Cardiovascular Complications of Cancer Therapy: Best Practices in Diagnosis, Prevention, and Management: Part 1. J Am Coll Cardiol 2017;70:2536-51. [Crossref] [PubMed]
- Jacobse JN, Schaapveld M, Boekel NB, et al. Risk of heart failure after systemic treatment for early breast cancer: results of a cohort study. Breast Cancer Res Treat 2021;185:205-14. [Crossref] [PubMed]
- Patnaik JL, Byers T, DiGuiseppi C, et al. Cardiovascular disease competes with breast cancer as the leading cause of death for older females diagnosed with breast cancer: a retrospective cohort study. Breast Cancer Res 2011;13:R64. [Crossref] [PubMed]
- Curigliano G, Cardinale D, Dent S, et al. Cardiotoxicity of anticancer treatments: Epidemiology, detection, and management. CA Cancer J Clin 2016;66:309-25. [Crossref] [PubMed]
- Zamorano JL, Lancellotti P, Rodriguez Muñoz D, et al. 2016 ESC Position Paper on cancer treatments and cardiovascular toxicity developed under the auspices of the ESC Committee for Practice Guidelines: The Task Force for cancer treatments and cardiovascular toxicity of the European Society of Cardiology (ESC). Eur J Heart Fail 2017;19:9-42. [Crossref] [PubMed]
- Ewer MS, Ewer SM. Cardiotoxicity of anticancer treatments. Nat Rev Cardiol 2015;12:547-58. [Crossref] [PubMed]
- Armenian SH, Hudson MM, Mulder RL, et al. Recommendations for cardiomyopathy surveillance for survivors of childhood cancer: a report from the International Late Effects of Childhood Cancer Guideline Harmonization Group. Lancet Oncol 2015;16:e123-36. [Crossref] [PubMed]
- Bovelli D, Plataniotis G, Roila F, et al. Cardiotoxicity of chemotherapeutic agents and radiotherapy-related heart disease: ESMO Clinical Practice Guidelines. Ann Oncol 2010;21:v277-82. [Crossref] [PubMed]
- Plana JC, Galderisi M, Barac A, et al. Expert consensus for multimodality imaging evaluation of adult patients during and after cancer therapy: a report from the American Society of Echocardiography and the European Association of Cardiovascular Imaging. J Am Soc Echocardiogr 2014;27:911-39. [Crossref] [PubMed]
- Dahou A, Clavel MA, Capoulade R, et al. Right ventricular longitudinal strain for risk stratification in low-flow, low-gradient aortic stenosis with low ejection fraction. Heart 2016;102:548-54. [Crossref] [PubMed]
- Guazzi M, Bandera F, Pelissero G, et al. Tricuspid annular plane systolic excursion and pulmonary arterial systolic pressure relationship in heart failure: an index of right ventricular contractile function and prognosis. Am J Physiol Heart Circ Physiol 2013;305:H1373-81. [Crossref] [PubMed]
- van Kessel M, Seaton D, Chan J, et al. Prognostic value of right ventricular free wall strain in pulmonary hypertension patients with pseudo-normalized tricuspid annular plane systolic excursion values. Int J Cardiovasc Imaging 2016;32:905-12. [Crossref] [PubMed]
- Risum N, Valeur N, Søgaard P, et al. Right ventricular function assessed by 2D strain analysis predicts ventricular arrhythmias and sudden cardiac death in patients after acute myocardial infarction. Eur Heart J Cardiovasc Imaging 2018;19:800-7. [Crossref] [PubMed]
- Śpiewak M, Kłopotowski M, Mazurkiewicz Ł, et al. Predictors of right ventricular function and size in patients with hypertrophic cardiomyopathy. Sci Rep 2020;10:21054. [Crossref] [PubMed]
- Moher D, Shamseer L, Clarke M, et al. Preferred reporting items for systematic review and meta-analysis protocols (PRISMA-P) 2015 statement. Syst Rev 2015;4:1. [Crossref] [PubMed]
- Wang B, Yu Y, Zhang Y, et al. Right ventricular dysfunction in patients with diffuse large B-cell lymphoma undergoing anthracycline-based chemotherapy: a 2D strain and 3D echocardiography study. Int J Cardiovasc Imaging 2021;37:1311-9. [Crossref] [PubMed]
- Zhao R, Shu F, Zhang C, et al. Early Detection and Prediction of Anthracycline-Induced Right Ventricular Cardiotoxicity by 3-Dimensional Echocardiography. JACC CardioOncol 2020;2:13-22. [Crossref] [PubMed]
- Wang Y, Lu C, Li H, et al. 3D-STI evaluation of the effect of dexrazoxane on the mechanical properties of right ventricular myocardium in breast cancer patients treated with pirarubicin. Ann Palliat Med 2020;9:1187-97. [Crossref] [PubMed]
- Planek MIC, Manshad A, Hein K, et al. Prediction of doxorubicin cardiotoxicity by early detection of subclinical right ventricular dysfunction. Cardiooncology 2020;6:10. [Crossref] [PubMed]
- Xu Y, Shi J, Zhao R, et al. Anthracycline induced inconsistent left ventricular segmental systolic function variation in patients with lymphoma detected by three-dimensional speckle tracking imaging. Int J Cardiovasc Imaging 2019;35:771-9. [Crossref] [PubMed]
- Khairat I, Khalfallah M, Shaban A, et al. Right ventricular 2D speckle-tracking echocardiography in children with osteosarcoma under chemotherapy. Egypt Heart J 2019;71:23. [Crossref] [PubMed]
- Keramida K, Farmakis D, Bingcang J, et al. Longitudinal changes of right ventricular deformation mechanics during trastuzumab therapy in breast cancer patients. Eur J Heart Fail 2019;21:529-35. [Crossref] [PubMed]
- Cherata DA, Donoiu I, Diaconu R, et al. Longitudinal strain analysis allows the identification of subclinical deterioration of right ventricular function in patients with cancer therapy-related left ventricular dysfunction. Discoveries (Craiova) 2019;7:e94. [Crossref] [PubMed]
- Chen L, Huang J, Wu W, et al. The impact of right ventricular function on prognosis in patients with stage III non-small cell lung cancer after concurrent chemoradiotherapy. Int J Cardiovasc Imaging 2019;35:1009-17. [Crossref] [PubMed]
- Anqi Y, Yu Z, Mingjun X, et al. Use of echocardiography to monitor myocardial damage during anthracycline chemotherapy. Echocardiography 2019;36:495-502. [Crossref] [PubMed]
- Wang Y, Zhang P, Liu K, et al. Evaluation of changes in right ventricular myocardial mechanical properties in breast cancer patients receiving pirarubicin using three-dimensional speckle tracking imaging. Nan Fang Yi Ke Da Xue Xue Bao 2018;38:1032-8. [PubMed]
- Arciniegas Calle MC, Sandhu NP, Xia H, et al. Two-dimensional speckle tracking echocardiography predicts early subclinical cardiotoxicity associated with anthracycline-trastuzumab chemotherapy in patients with breast cancer. BMC Cancer 2018;18:1037. [Crossref] [PubMed]
- Song FY, Shi J, Guo Y, et al. Assessment of biventricular systolic strain derived from the two-dimensional and three-dimensional speckle tracking echocardiography in lymphoma patients after anthracycline therapy. Int J Cardiovasc Imaging 2017;33:857-68. [Crossref] [PubMed]
- Paraskevaidis IA, Makavos G, Tsirigotis P, et al. Deformation Analysis of Myocardial Layers Detects Early Cardiac Dysfunction after Chemotherapy in Bone Marrow Transplantation Patients: A Continuous and Additive Cardiotoxicity Process. J Am Soc Echocardiogr 2017;30:1091-102. [Crossref] [PubMed]
- Moustafa S, Murphy K, Nelluri BK, et al. Temporal Trends of Cardiac Chambers Function with Trastuzumab in Human Epidermal Growth Factor Receptor II-Positive Breast Cancer Patients. Echocardiography 2016;33:406-15. [Crossref] [PubMed]
- Lange SA, Jung J, Jaeck A, et al. Subclinical Myocardial Impairment Occurred in Septal and Anterior LV Wall Segments After Anthracycline-Embedded Chemotherapy and did not Worsen During Adjuvant Trastuzumab Treatment in Breast Cancer Patients. Cardiovasc Toxicol 2016;16:193-206. [Crossref] [PubMed]
- Chang WT, Shih JY, Feng YH, et al. The Early Predictive Value of Right Ventricular Strain in Epirubicin-Induced Cardiotoxicity in Patients with Breast Cancer. Acta Cardiol Sin 2016;32:550-9. [PubMed]
- Boczar KE, Aseyev O, Sulpher J, et al. Right heart function deteriorates in breast cancer patients undergoing anthracycline-based chemotherapy. Echo Res Pract 2016;3:79-84. [Crossref] [PubMed]
- Mornoş C, Manolis AJ, Cozma D, et al. The value of left ventricular global longitudinal strain assessed by three-dimensional strain imaging in the early detection of anthracyclinemediated cardiotoxicity. Hellenic J Cardiol 2014;55:235-44. [PubMed]
- Mornoş C, Petrescu L. Early detection of anthracycline-mediated cardiotoxicity: the value of considering both global longitudinal left ventricular strain and twist. Can J Physiol Pharmacol 2013;91:601-7. [Crossref] [PubMed]
- Xu H, Mao L, Liu H, et al. Assessment of Subclinical Deterioration of Right Ventricular Function by Three-Dimensional Speckle Tracking Echocardiography in Breast Cancer Patients Undergoing Anthracycline-Based Chemotherapy. Int J Gen Med 2021;14:885-93. [Crossref] [PubMed]
- Mordente A, Meucci E, Silvestrini A, et al. Anthracyclines and mitochondria. Adv Exp Med Biol 2012;942:385-419. [Crossref] [PubMed]
- De Keulenaer GW, Doggen K, Lemmens K. The vulnerability of the heart as a pluricellular paracrine organ: lessons from unexpected triggers of heart failure in targeted ErbB2 anticancer therapy. Circ Res 2010;106:35-46. [Crossref] [PubMed]
- Banfill K, Giuliani M, Aznar M, et al. Cardiac Toxicity of Thoracic Radiotherapy: Existing Evidence and Future Directions. J Thorac Oncol 2021;16:216-27. [Crossref] [PubMed]
- Meyer P, Filippatos GS, Ahmed MI, et al. Effects of right ventricular ejection fraction on outcomes in chronic systolic heart failure. Circulation 2010;121:252-8. [Crossref] [PubMed]
- Gulati A, Ismail TF, Jabbour A, et al. The prevalence and prognostic significance of right ventricular systolic dysfunction in nonischemic dilated cardiomyopathy. Circulation 2013;128:1623-33. [Crossref] [PubMed]
- Lang RM, Badano LP, Mor-Avi V, et al. Recommendations for cardiac chamber quantification by echocardiography in adults: an update from the American Society of Echocardiography and the European Association of Cardiovascular Imaging. J Am Soc Echocardiogr 2015;28:1-39.e14. [Crossref] [PubMed]
- Longobardo L, Suma V, Jain R, et al. Role of Two-Dimensional Speckle-Tracking Echocardiography Strain in the Assessment of Right Ventricular Systolic Function and Comparison with Conventional Parameters. J Am Soc Echocardiogr 2017;30:937-946.e6. [Crossref] [PubMed]
- Kind T, Mauritz GJ, Marcus JT, et al. Right ventricular ejection fraction is better reflected by transverse rather than longitudinal wall motion in pulmonary hypertension. J Cardiovasc Magn Reson 2010;12:35. [Crossref] [PubMed]
- Maffessanti F, Gripari P, Tamborini G, et al. Evaluation of right ventricular systolic function after mitral valve repair: a two-dimensional Doppler, speckle-tracking, and three-dimensional echocardiographic study. J Am Soc Echocardiogr 2012;25:701-8. [Crossref] [PubMed]
- Giusca S, Dambrauskaite V, Scheurwegs C, et al. Deformation imaging describes right ventricular function better than longitudinal displacement of the tricuspid ring. Heart 2010;96:281-8. [Crossref] [PubMed]
- Schwarz K, Singh S, Dawson D, et al. Right ventricular function in left ventricular disease: pathophysiology and implications. Heart Lung Circ 2013;22:507-11. [Crossref] [PubMed]
- Melenovsky V, Hwang SJ, Lin G, et al. Right heart dysfunction in heart failure with preserved ejection fraction. Eur Heart J 2014;35:3452-62. [Crossref] [PubMed]
- Aschauer S, Kammerlander AA, Zotter-Tufaro C, et al. The right heart in heart failure with preserved ejection fraction: insights from cardiac magnetic resonance imaging and invasive haemodynamics. Eur J Heart Fail 2016;18:71-80. [Crossref] [PubMed]
- Lejeune S, Roy C, Ciocea V, et al. Right Ventricular Global Longitudinal Strain and Outcomes in Heart Failure with Preserved Ejection Fraction. J Am Soc Echocardiogr 2020;33:973-984.e2. [Crossref] [PubMed]
- Houard L, Benaets MB, de Meester de Ravenstein C, et al. Additional Prognostic Value of 2D Right Ventricular Speckle-Tracking Strain for Prediction of Survival in Heart Failure and Reduced Ejection Fraction: A Comparative Study With Cardiac Magnetic Resonance. JACC Cardiovasc Imaging 2019;12:2373-85. [Crossref] [PubMed]
- Ho E, Brown A, Barrett P, et al. Subclinical anthracycline- and trastuzumab-induced cardiotoxicity in the long-term follow-up of asymptomatic breast cancer survivors: a speckle tracking echocardiographic study. Heart 2010;96:701-7. [Crossref] [PubMed]
- Franco V. Right ventricular remodeling in pulmonary hypertension. Heart Fail Clin 2012;8:403-12. [Crossref] [PubMed]
- D'Andrea A, D'Alto M, Di Maio M, et al. Right atrial morphology and function in patients with systemic sclerosis compared to healthy controls: a two-dimensional strain study. Clin Rheumatol 2016;35:1733-42. [Crossref] [PubMed]
- Bi X, Deng Y, Zeng F, et al. Evaluation of epirubicin-induced cardiotoxicity by two-dimensional strain echocardiography in breast cancer patients. J Huazhong Univ Sci Technolog Med Sci 2009;29:391-4. [Crossref] [PubMed]