CircPTN promotes angiogenesis via the MiR-595/LYRM5 signaling pathway in non-small cell lung cancer
Introduction
Lung carcinoma is a highly malignant tumor with a poor prognosis worldwide (1). It is divided into the following 2 subtypes: non-small cell lung cancer (NSCLC) and small-cell lung cancer (SCLC). NSCLC represents about 80% of all lung carcinoma cases (2,3). Angiogenesis plays a key role in most malignant tumors, including NSCLC (4). Abundant angiogenesis plays an important role in tumor malignant proliferation and metastasis (5-7). Thus, anti-angiogenic treatments efficiently prevent tumor growth and metastasis. However, the exact molecular mechanism associated with NSCLC angiogenesis is poorly defined.
Circular ribonucleic acids (circRNAs) are derived from the “back-splicing” of precursor messenger RNA (pre-mRNA) transcripts to form a covalently closed circular structure without a 3'polyA tail or 5' to 3' polarity (8-10). CircRNAs are more stable than linear RNAs, and provide resistance to degradation by ribonuclease R (11-13). There is emerging evidence that circRNAs are aberrantly expressed in a variety of human tumors, including gastric cancer (14), glioma (15), hepatocellular carcinoma (16), esophageal cancer (17), lung cancer (18), and breast cancer (19). CircRNAs play a vital role in many physiological and pathological processes (20). In tumors, circRNAs are involved in various aspects of malignant phenotypes, including apoptosis, the cell cycle, angiogenesis, invasion, and metastasis (21).
Circular pleiotrophin (circPTN) is derived from the pleiotrophin (PTN) gene. Recently, circPTN has been reported to sponge microRNA-330-5p/microRNA -145-5p to promote stemness and proliferation in glioma (22). CircPTN is highly expressed in hepatocellular cancer tissues, and promotes the growth of hepatocellular cancer by sponging miR-326 and inducing ErbB/PI3K expression (23). Additionally, circPTN inhibits glioma cell glycolysis, proliferation, and invasion by regulating the miR-432-5p/RAB10 axis (24). However, the roles of circPTN in the angiogenesis of NSCLC are largely unknown. Previous studies just reported circPTN have the promoting role in Glioma and hepatocellular carcinoma (22-24). Our team firstly confirmed circPTN promote NSCLC metastasis and via up-regulating LYRM5 protein expression, which is our study biggest innovation in the NSCLC
In the present study, we measured circPTN expression in human NSCLC tissues via quantitative real-time polymerase chain reaction (qRT-PCR). Additionally, the function and potential mechanisms of circPTN in NSCLC angiogenesis were explored. We found that a higher circPTN level was closely related to angiogenesis, and significantly shorter overall survival in patients with NSCLC. Further, we found preliminary evidence that circPTN upregulates the expression of LYRM5 by sponging miR-595, which facilitates NSCLC angiogenesis.
We present the following article in accordance with the MDAR reporting checklist (available at https://tcr.amegroups.com/article/view/10.21037/tcr-22-195/rc).
Methods
NSCLC tissues collection
In total, 80 pairs of NSCLC and matched normal tissues were obtained from the Department of Cardiothoracic Surgery, Zhuji People’s Hospital from June 2019 to December 2019. The experimental protocol was reviewed and approved by the Research Ethics Committee of Zhuji People’s Hospital (No. 2019056). A formal informed consent form was signed by each participant before sample collection. The study was conducted in accordance with the Declaration of Helsinki (as revised in 2013).
Cell culture
The NSCLC cell lines [A549 (ATCC® CRM-CCL-185), H1299 (ATCC® CRL-5803), and H358 (ATCC® CRL-5807)] and normal human bronchial epithelial cell line (16HBE) were purchased from ATCC® (Maryland, USA). The NSCLC line PC-9 was gifted by the Shanghai Jiao Tong University.
The H358, H1299, and PC-9 cells were cultured in Roswell Park Memorial Institute (RPMI) 1640 medium containing 10% fetal bovine serum (FBS). 16HBE and A549 were maintained in Dulbecco’s modified Eagle’s medium supplemented with 10% FBS. These cell lines were cultured in an incubator at 37 ℃ with an atmosphere of 5% carbon dioxide.
Immunohistochemistry
The experiment was performed using methods described previously (25). Paraffin-embedded tissue samples were incubated overnight using a primary antibody against cluster of differentiation 31 (CD31) (ab24590). The antibody was purchased from Abcam (Cambridge, UK). Images were taken via microscopy.
qRT-PCR
Total RNA was extracted and reverse transcribed into complementary deoxyribonucleic acid (cDNA). SYBR-Green (TaKaRa, China) was used to perform the qRT-PCR. β-actin and U6 were used as the internal controls. The relative quantification 2−ΔΔCT was applied to assess the level of circPTN, miR-595, and LYRM5.
Tube formation assay
The 96-well plates were incubated with 50 µL of dissolved Matrigel matrix (BD Biosciences, USA) at 37 ℃ for 30 minutes. 2×104/well human umbilical vein endothelial cells (HUVECs) were resuspended in 100 µL of serum-free medium, and seeded in the wells with dissolved Matrigel. Tube formation was observed under the microscope (Leica, Germany) after incubation at 37 ℃ for 6 to 8 hours. The total tubular length per well was assessed via ImageJ software.
Western blot
Western blot was performed as previously described (26) to test the level of LYRM5 protein. Briefly, 60 µg of protein was electrophoresed with 12.0% sodium dodecyl sulfate polyacrylamide gel electrophoresis and transferred onto polyvinylidene fluoride (PVDF) membranes (Millipore, USA). At 4 ℃, the primary antibody of LYRM5 (ab126379) (Abcam, UK) was incubated overnight. Band intensities were quantitated by enhanced chemiluminescence (ECL) (Beyotime, Shanghai, China).
Dual-luciferase reporter assay
The possible binding regions for miR-595 in circPTN and LYRM5 were predicted through a bioinformatics analysis. H1299 and A549 cells were transfected with miR-595-mimic and reporter plasmids. Reporter plasmids was purchased from Thermo Fisher Scientific. A dual-luciferase assay system (Promega, USA) was used to conduct the luciferase reporter assay.
Statistical analysis
The statistical analysis was performed by GraphPad Prism 8. The experiments were performed in triplicate. The data in this study are presented as the mean ± standard deviation. The correlation among the groups was explored via a Pearson correlation analysis. A Student’s t-test or 1-way analysis of variance was used to compare quantitative variables. A P value <0.05 was considered statistically significant.
Results
CircPTN is upregulated and closely associated with angiogenesis in NSCLC
To investigate circPTN expression in NSCLC, we assessed its expression in 80 pairs of NSCLC tissues and matched normal tissues through qRT-PCR. We found that the circPTN level was higher in the NSCLC tissues than the corresponding normal tissues (see Figure 1A). Additionally, the Kaplan-Meier analysis showed that the overall survival of patients with higher circPTN expression was decreased (see Figure 1B). To further explore the effect of circPTN on angiogenesis in NSCLC, we detected the expression of CD31 in NSCLC tissues via immunohistochemistry. Increased CD31 expression was observed in high circPTN expression tumor tissues (see Figure 1C,1D). Additionally, the circPTN expression level was closely associated with microvascular density in NSCLC (see Figure 1E). These results suggest that circPTN is upregulated and closely associated with angiogenesis in NSCLC.
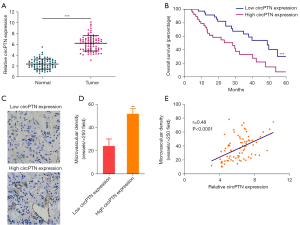
CircPTN promotes angiogenesis in NSCLC
To identify the effect of circPTN on the angiogenesis of NSCLC, circPTN expression was detected in NSCLC cell lines (H1299, H358, PC-9, and A549), and in normal bronchial epithelial cells (16HBE) by qRT-PCR. As Figure 2A shows, there was higher circPTN expression in the A549 and H1299 cell lines than the other 2 NSCLC cell lines. Next, A549 and H1299 cells were transfected with si-circPTN or si-NC, and si-circPTN transection efficiency was assessed through qRT-PCR (see Figure 2B). Conditioned medium from the A549 or H1299 cells transfected with si-circPTN or si-NC was collected, and HUVECs were stimulated with it. Compared to the si-NC group, decreased tube formation was detected in the si-circPTN group (see Figure 2C,2D). The results showed that circPTN promotes angiogenesis in NSCLC.
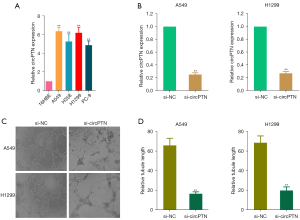
CircPTN facilitates angiogenesis by regulating LYRM5 in NSCLC
LYRM5 (also referred to as Ghiso) is a mitochondrial protein, and a member of the Complex1_LYR-like Superfamily (27,28). LYRM proteins play key roles in acetate metabolism, and in essential Fe-S cluster biogenesis (28). Mitochondria have been shown to be involved in the occurrence and development of carcinoma (29,30). To explore the role of circPTN in the expression of LYRM5, the LYRM5 protein and mRNA expression in the H1299 or A549 cells transfected with si-circPTN or si-NC were measured. We found that silenced circPTN suppressed LYRM5 mRNA and protein expression (see Figure 3A,3B). Next, tube formation assays were carried out using the HUVECs stimulated with conditioned medium from the A549 or H1299 cells transfected with si-LYRM5 or si-Control. The silencing of LYRM5 resulted in the significant inhibition of angiogenesis (see Figure 3C,3D). Our observations indicated that circPTN facilitates angiogenesis by regulating LYRM5 in NSCLC.
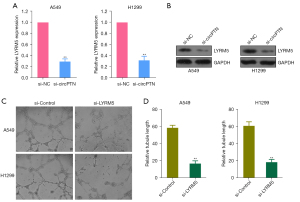
MiR-595 is an inhibitory target of circPTN
There is accumulating evidence that circRNAs are involved in the development and progression of cancer by serving as competing endogenous RNAs (ceRNAs), and competitively reduce miRNA activity (31-33). To further explore the molecular mechanisms associated with circPTN-induced LYRM5 expression, bioinformatics databases (RNAhybrid, TargetScan, and miRanda) were used to predict the potential target miRNAs. There was a potential binding site between circPTN and MiR-595 (see Figure 4A). The interaction between them was confirmed by dual-luciferase reporter assays (see Figure 4B). Additionally, the silencing of circPTN resulted in an obvious increase in miR-595 expression in A549 and H1299 cells (see Figure 4C). In NSCLC tissues, an inverse correlation between miR-595 and circPTN was verified via a Pearson correlation analysis (see Figure 4D). These data showed that miR-595 can be sponged by circPTN in NSCLC.
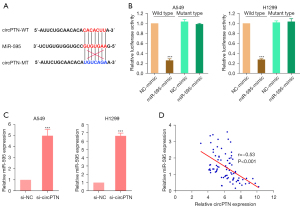
LYRM5 is a direct target of miR-595
To identify the role of miR-595 in LYRM5 expression induced by circPTN, the possible targets of miR-595 were predicted via a bioinformatic analysis using miRDB and TargetScan. A binding site for miR-595 in LYRM5 was identified (see Figure 5A). Subsequently, the interactions of miR-595 and LYRM5 was demonstrated through luciferase reporter assays (see Figure 5B). Additionally, our results showed that the protein and mRNA levels of LYRM5 were dramatically more reduced in the miR-595-mimic group than the negative control (NC)-mimic group (see Figure 5C,5D). A negative relationship between LYRM5 and miR-595 in NSCLC tissues was confirmed via a Pearson correlation analysis (see Figure 5E). More importantly, we proved that the inhibitory effect of silenced circPTN on the angiogenesis of HUVECs cells was attenuated by miR-595 (see Figure 5F). Above all, our findings revealed that LYRM5 is a direct target of miR-595 in NSCLC.
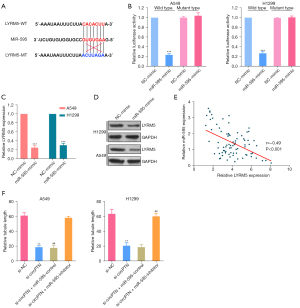
Discussion
It is estimated that deaths due to malignant lung cancer represent roughly 1/5 of all deaths worldwide (34,35). NSCLC represents about 80% of all lung carcinoma cases among the common subtypes of lung carcinoma (36). Until the last decade, the 5-year overall survival rate of patients with metastatic NSCLC was <5% (36). An improved understanding of the biology of NSCLC has resulted in the development of new biomarker-targeted therapies, and led to improvements in overall survival for patients with metastatic or advanced NSCLC. For many tumors, including NSCLC, angiogenesis has been taken as an essential therapeutic target. Angiogenesis is involved in the process of primary tumor growth, proliferation, and metastasis (4,6,7,37). In the present study, we verified that circPTN upregulated LYRM5 expression by sponging miR-595, which promoted NSCLC angiogenesis.
CircRNAs are derived from the “back-splicing” of pre-mRNA transcripts to form a covalently closed circular structure without a 3' polyA tail or 5' to 3' polarity (8-10). It is well established that circRNAs are aberrantly expressed in a variety of human tumors, and involved in various aspects of malignant phenotypes, including apoptosis, cell cycle, angiogenesis, and invasion, and metastasis, as an RNA sponge (8,10,21,38,39). CircPTN is derived from the PTN gene. Recently, circPTN has been reported to sponge miR-145-5p/miR-330-5p to promote proliferation and stemness in glioma (22). CircPTN is highly expressed in hepatocellular cancer tissues, and promotes the growth of hepatocellular cancer by sponging miR-326 and inducing ErbB/PI3K expression (23). Additionally, circPTN inhibits glioma cell glycolysis, proliferation, and invasion by regulating the miR-432-5p/RAB10 signaling pathway (24).
Numerous studies have confirmed that circRNAs are involved in tumor angiogenesis (40-42). For example, circ-CCAC1 levels are increased in cancerous bile-resident extracellular vesicles and cholangiocarcinoma tissues, and circ-CCAC1 from CCA-derived extracellular vesicles is transferred to endothelial monolayer cells, disrupting endothelial barrier integrity and inducing angiogenesis (40). SRSF10 facilitates circ-ATXN1 biogenesis and subsequently increases its binding with miR-526b-3p to induce metallopeptidase and vascular endothelia growth factor A (VEGFA), thus regulating glioma angiogenesis (41). Circ-001971 promotes the proliferation, invasion and angiogenesis of colorectal cancer via the miR-29c-3p/VEGFA axis (42). Similar to previous results, we found that circPTN was highly expressed in NSCLC tissues. A higher circPTN level was closely associated with angiogenesis and short overall survival in patients with NSCLC. With the development of small molecule drugs, the well-designed circPTN interference peptide is a novel useful therapeutic target to treat NSCLC patients with the highly circPTN expression. Moreover, the circPTN levels could be employed to predict the prognosis of NSCLC patients. Moreover, circPTN facilitated angiogenesis by regulating LYRM5 in NSCLC. Currently, just the miRNA-based drugs have been used in clinical trials (NCT03713320, NCT03837457). In comparison with miRNA, circRNA has the more complex circular conformation and sequence overlap with linear mRNA counterparts (43). In addition, circRNA is limited by drug chemistry, drug formulations and different routes of administration. All in all, circRNA is unable to be applied in the medical field. With the development of technology, the circRNA will be used in the future clinical trial.
The mechanisms associated with the functional roles of circRNAs in regulating tumorigenesis are still unclear. However, there is accumulating evidence that circRNAs regulate the expression of oncogenic or tumor-suppressive genes by acting as miRNA sponges and forming the circRNA-miRNA-mRNA signaling pathway (13). Chen J teams have verified that circPTN sponges miR-145-5p/miR-330-5p to promote proliferation and stemness in glioma (22). In hepatocellular carcinoma, circPTN promotes the growth of hepatocellular carcinoma by sponging miR-326 and inducing ErbB/PI3K expression (23). Additionally, circPTN suppresses glioma cell glycolysis, proliferation, and invasion by the miR-432-5p/RAB10 axis (24). In the present study, our results showed that LYRM5 could be a direct target of miR-595. Further, we found that circPTN upregulated LYRM5 expression by sponging miR-595, which facilitated NSCLC angiogenesis.
Our study proved for the first time that circPTN was highly expressed in NSCLC tissues. A higher circPTN level was closely associated with angiogenesis and significantly shorter overall survival in patients with NSCLC. Additionally, circPTN facilitated angiogenesis by regulating LYRM5 in NSCLC. Further, we found that circPTN upregulated LYRM5 expression by sponging miR-595, which promoted angiogenesis in NSCLC. Overall, circPTN serves as a ceRNA to promote NSCLC angiogenesis via the miR-595/LYRM5 signaling pathway. Our findings provide potential targets for the treatment of patients with NSCLC.
Acknowledgments
Funding: None.
Footnote
Reporting Checklist: The authors have completed the MDAR reporting checklist. Available at https://tcr.amegroups.com/article/view/10.21037/tcr-22-195/rc
Data Sharing Statement: Available at https://tcr.amegroups.com/article/view/10.21037/tcr-22-195/dss
Conflicts of Interest: All authors have completed the ICMJE uniform disclosure form (available at https://tcr.amegroups.com/article/view/10.21037/tcr-22-195/coif). The authors have no conflicts of interest to declare.
Ethical Statement: The authors are accountable for all aspects of the work in ensuring that questions related to the accuracy or integrity of any part of the work are appropriately investigated and resolved. The experimental protocol was reviewed and approved by the Research Ethics Committee of Zhuji People’s Hospital (No. 2019056). A formal informed consent form was signed by each participant before sample collection. The study was conducted in accordance with the Declaration of Helsinki (as revised in 2013).
Open Access Statement: This is an Open Access article distributed in accordance with the Creative Commons Attribution-NonCommercial-NoDerivs 4.0 International License (CC BY-NC-ND 4.0), which permits the non-commercial replication and distribution of the article with the strict proviso that no changes or edits are made and the original work is properly cited (including links to both the formal publication through the relevant DOI and the license). See: https://creativecommons.org/licenses/by-nc-nd/4.0/.
References
- Bray F, Ferlay J, Soerjomataram I, et al. Global cancer statistics 2018: GLOBOCAN estimates of incidence and mortality worldwide for 36 cancers in 185 countries. CA Cancer J Clin 2018;68:394-424. [Crossref] [PubMed]
- Wang S, Zhang X, Yang C, et al. MicroRNA-198-5p inhibits the migration and invasion of non-small lung cancer cells by targeting fucosyltransferase 8. Clin Exp Pharmacol Physiol 2019;46:955-67. [Crossref] [PubMed]
- Stumpf C, Kaemmerer D, Neubauer E, et al. Somatostatin and CXCR4 expression patterns in adenocarcinoma and squamous cell carcinoma of the lung relative to small cell lung cancer. J Cancer Res Clin Oncol 2018;144:1921-32. [Crossref] [PubMed]
- Sunamura M, Duda DG, Ghattas MH, et al. Heme oxygenase-1 accelerates tumor angiogenesis of human pancreatic cancer. Angiogenesis 2003;6:15-24. [Crossref] [PubMed]
- Chen LT, Xu SD, Xu H, et al. MicroRNA-378 is associated with non-small cell lung cancer brain metastasis by promoting cell migration, invasion and tumor angiogenesis. Med Oncol 2012;29:1673-80. [Crossref] [PubMed]
- Hall RD, Le TM, Haggstrom DE, et al. Angiogenesis inhibition as a therapeutic strategy in non-small cell lung cancer (NSCLC). Transl Lung Cancer Res 2015;4:515-23. [PubMed]
- Hanahan D, Weinberg RA. Hallmarks of cancer: the next generation. Cell 2011;144:646-74. [Crossref] [PubMed]
- Li J, Sun D, Pu W, et al. Circular RNAs in Cancer: Biogenesis, Function, and Clinical Significance. Trends Cancer 2020;6:319-36. [Crossref] [PubMed]
- Xiao MS, Ai Y, Wilusz JE. Biogenesis and Functions of Circular RNAs Come into Focus. Trends Cell Biol 2020;30:226-40. [Crossref] [PubMed]
- Kristensen LS, Andersen MS, Stagsted LVW, et al. The biogenesis, biology and characterization of circular RNAs. Nat Rev Genet 2019;20:675-91. [Crossref] [PubMed]
- Chen LL, Yang L. Regulation of circRNA biogenesis. RNA Biol 2015;12:381-8. [Crossref] [PubMed]
- Jeck WR, Sharpless NE. Detecting and characterizing circular RNAs. Nat Biotechnol 2014;32:453-61. [Crossref] [PubMed]
- Memczak S, Jens M, Elefsinioti A, et al. Circular RNAs are a large class of animal RNAs with regulatory potency. Nature 2013;495:333-8. [Crossref] [PubMed]
- Ma C, Wang X, Yang F, et al. Circular RNA hsa_circ_0004872 inhibits gastric cancer progression via the miR-224/Smad4/ADAR1 successive regulatory circuit. Mol Cancer 2020;19:157. [Crossref] [PubMed]
- Song X, Zhang N, Han P, et al. Circular RNA profile in gliomas revealed by identification tool UROBORUS. Nucleic Acids Res 2016;44:e87. [Crossref] [PubMed]
- Li Y, Zheng Q, Bao C, et al. Circular RNA is enriched and stable in exosomes: a promising biomarker for cancer diagnosis. Cell Res 2015;25:981-4. [Crossref] [PubMed]
- Su H, Lin F, Deng X, et al. Profiling and bioinformatics analyses reveal differential circular RNA expression in radioresistant esophageal cancer cells. J Transl Med 2016;14:225. [Crossref] [PubMed]
- Xu T, Yu Y, Guo S, et al. CircPSMC3 Suppresses Migration and Invasion of Non-Small Cell Lung Cancer Cells via miR-182-5p/NME2 Axis. Med Sci Monit 2020;26:e924134. [Crossref] [PubMed]
- Ren S, Liu J, Feng Y, et al. Knockdown of circDENND4C inhibits glycolysis, migration and invasion by up-regulating miR-200b/c in breast cancer under hypoxia. J Exp Clin Cancer Res 2019;38:388. [Crossref] [PubMed]
- Wang Y, Mo Y, Gong Z, et al. Circular RNAs in human cancer. Mol Cancer 2017;16:25. [Crossref] [PubMed]
- Zhong Y, Du Y, Yang X, et al. Circular RNAs function as ceRNAs to regulate and control human cancer progression. Mol Cancer 2018;17:79. [Crossref] [PubMed]
- Chen J, Chen T, Zhu Y, et al. circPTN sponges miR-145-5p/miR-330-5p to promote proliferation and stemness in glioma. J Exp Clin Cancer Res 2019;38:398. [Crossref] [PubMed]
- Jia B, Yin X, Wang Y, et al. CircRNA-PTN Sponges miR-326 to Promote Proliferation in Hepatocellular Carcinoma. Onco Targets Ther 2020;13:4893-903. [Crossref] [PubMed]
- Zhang X, Wang S, Lin G, et al. Down-regulation of circ-PTN suppresses cell proliferation, invasion and glycolysis in glioma by regulating miR-432-5p/RAB10 axis. Neurosci Lett 2020;735:135153. [Crossref] [PubMed]
- Chen J, Chen S, Zhuo L, et al. Regulation of cancer stem cell properties, angiogenesis, and vasculogenic mimicry by miR-450a-5p/SOX2 axis in colorectal cancer. Cell Death Dis 2020;11:173. [Crossref] [PubMed]
- Chen C, Xu ZQ, Zong YP, et al. CXCL5 induces tumor angiogenesis via enhancing the expression of FOXD1 mediated by the AKT/NF-κB pathway in colorectal cancer. Cell Death Dis 2019;10:178. [Crossref] [PubMed]
- Pagliarini DJ, Calvo SE, Chang B, et al. A mitochondrial protein compendium elucidates complex I disease biology. Cell 2008;134:112-23. [Crossref] [PubMed]
- Angerer H. The superfamily of mitochondrial Complex1_LYR motif-containing (LYRM) proteins. Biochem Soc Trans 2013;41:1335-41. [Crossref] [PubMed]
- Park D, Dilda PJ. Mitochondria as targets in angiogenesis inhibition. Mol Aspects Med 2010;31:113-31. [Crossref] [PubMed]
- Galluzzi L, Morselli E, Kepp O, et al. Mitochondrial gateways to cancer. Mol Aspects Med 2010;31:1-20. [Crossref] [PubMed]
- Zhou WY, Cai ZR, Liu J, et al. Circular RNA: metabolism, functions and interactions with proteins. Mol Cancer 2020;19:172. [Crossref] [PubMed]
- Xu T, Wang M, Jiang L, et al. CircRNAs in anticancer drug resistance: recent advances and future potential. Mol Cancer 2020;19:127. [Crossref] [PubMed]
- Wu P, Mo Y, Peng M, et al. Emerging role of tumor-related functional peptides encoded by lncRNA and circRNA. Mol Cancer 2020;19:22. [Crossref] [PubMed]
- Adjei AA. Lung Cancer Worldwide. J Thorac Oncol 2019;14:956. [Crossref] [PubMed]
- Arbour KC, Riely GJ. Systemic Therapy for Locally Advanced and Metastatic Non-Small Cell Lung Cancer: A Review. JAMA 2019;322:764-74. [Crossref] [PubMed]
- Govindan R, Page N, Morgensztern D, et al. Changing epidemiology of small-cell lung cancer in the United States over the last 30 years: analysis of the surveillance, epidemiologic, and end results database. J Clin Oncol 2006;24:4539-44. [Crossref] [PubMed]
- Aggarwal C, Somaiah N, Simon G. Antiangiogenic agents in the management of non-small cell lung cancer: where do we stand now and where are we headed? Cancer Biol Ther 2012;13:247-63. [Crossref] [PubMed]
- Yu T, Wang Y, Fan Y, et al. CircRNAs in cancer metabolism: a review. J Hematol Oncol 2019;12:90. [Crossref] [PubMed]
- Su M, Xiao Y, Ma J, et al. Circular RNAs in Cancer: emerging functions in hallmarks, stemness, resistance and roles as potential biomarkers. Mol Cancer 2019;18:90. [Crossref] [PubMed]
- Xu Y, Leng K, Yao Y, et al. A novel circular RNA, circ-CCAC1, contributes to CCA progression, induces angiogenesis, and disrupts vascular endothelial barriers. Hepatology 2021;73:1419-35. [Crossref] [PubMed]
- Liu X, Shen S, Zhu L, et al. SRSF10 inhibits biogenesis of circ-ATXN1 to regulate glioma angiogenesis via miR-526b-3p/MMP2 pathway. J Exp Clin Cancer Res 2020;39:121. [Crossref] [PubMed]
- Chen C, Huang Z, Mo X, et al. The circular RNA 001971/miR-29c-3p axis modulates colorectal cancer growth, metastasis, and angiogenesis through VEGFA. J Exp Clin Cancer Res 2020;39:91. [Crossref] [PubMed]
- Li X, Yang L, Chen LL. The Biogenesis, Functions, and Challenges of Circular RNAs. Mol Cell 2018;71:428-42. [Crossref] [PubMed]