Demethoxycurcumin induces apoptosis via inhibition of NF-κB pathway in FaDu human head and neck squamous cell carcinoma
Introduction
Human head and neck squamous cell carcinoma (HNSCC) develops from the mucosal epithelium in the oral cavity, pharynx, and larynx. The etiology of HNSCC, the sixth most common cancer type worldwide, is closely correlated with exposure to tobacco-derived carcinogens, excessive alcohol consumption, and human papillomavirus infection. Despite the incorporation of several therapeutic modalities such as surgery, radiation therapy, and chemotherapy, the survival rate of HNSCC is still estimated to be less than 60%. Furthermore, these clinical strategies may fail due to several pathological characteristics of HNSCC, such as rapid tumor growth and tumor progression, and also lead patients to permanent disability caused by several lesions, including the alteration of mechanical oral functions, dysfunction in swallowing, and malnutrition. Hence, clinical interventions with fewer side effects and minimal toxicity are urgently required for treating patients with HNSCC.
Natural and herbal products that have shown efficacy for cancer treatment in traditional folk medicine have been considered as potential chemotherapeutic agents due to their low adverse reactions and low toxicity. Among the 121 drugs prescribed for cancer treatment, such as vinca alkaloids, taxanes, podophyllotoxin, and antra cyclins, which were approved by the FDA from 1984 to 1994, 90 drugs are derived from natural sources used in herbal medicine (1,2). Furthermore, some anti-cancer drugs isolated from herbs have been shown to induce apoptotic cell death, a process that is blocked in cancer cells (3).
Curcumin, a phenolic compound derived from Curcuma longa, is a well-known herbal plant used in traditional medicine that has shown anti-cancer effects through inhibition of proliferation, angiogenesis, and metastasis in most cancers, including colorectal (4), breast (5), gastric (6), prostate (7), and oral cancer (8). Furthermore, demethoxycurcumin (DMC, CAS# 22608-11-3, C20H18O5) is a derivative of curcumin in which one of the methoxy groups is replaced by hydrogen (Figure 1). Similar to curcumin, it has shown anti-cancer effects in various cancers such as leukemia, breast, renal, lung, and oral squamous cell carcinoma. However, the anti-cancer effects of DMC have not yet been reported in FaDu cells. Therefore, the aim of this study was to verify the anti-cancer effects of DMC and its cellular signaling pathways associated with cancer cell death in FaDu cells. We present the following article in accordance with the MDAR reporting checklist (available at https://tcr.amegroups.com/article/view/10.21037/tcr-21-2410/rc).
Methods
Cell culture
FaDu cells, a human pharyngeal squamous cell line, were obtained from the American Type Culture Collection (ATCC). Based on the ATCC instructions, FaDu cells were maintained in minimum essential medium (Life Technologies, Grand Island, NY, USA) containing 10% fetal bovine serum (FBS, Life Technologies) in a humidified incubator at 37 ℃ with 5% CO2.
Cell viability assay
To determine the cytotoxicity of DMC (Sigma-Aldrich, St. Louis, MO, USA), FaDu cells (1×105 cells/mL) were grown in 96-well culture plates and were stimulated with 0, 1, 10, 20, 50, and 100 µM DMC for 24 h at 37 ℃. Afterwards, FaDu cells were cultured for an additional 4 h in 20 µL of 5 mg/mL 3-(4,5-dimethylthiazol-2-yl)-2,5-diphenyltetrazolium bromide (MTT) (Life Technologies). To dissolve the MTT crystal, 200 µL of dimethyl sulfoxide (DMSO) was added, after the remove of supernatant. Thereafter, DMSO containing dissolved MTT crystal was measured at 570 nm by a spectrophotometer (Epoch microplate spectrophotometer, BioTek Instruments, Winooski, VT, USA). The experiments were performed independently three replicates. The mean OD ± standard deviation for each group of replicates was calculated.
Live/Dead cell assay
To visualize both live and dead cells, we used the Live/Dead cell viability assay kit (Thermo Fisher Scientific, Waltham, MA, USA), which is composed of green calcein-AM to stain as a green fluorescence the cytosol of live cells and ethidium homodimer-1 to stain as a red fluorescence the nucleus of dead cells. FaDu cells (1×105 cells/mL) were grown in an 8-well chamber slide (Nunc® Lab-Tek® Chamber SlideTM system, Sigma-Aldrich) and allowed to attach on the bottom of well for 24 h at 37 ℃. Thereafter, cultured FaDu cells were stimulated with 0, 10, or 20 µM DMC for 24 h at 37 ℃ and stained by the Live/Dead cell viability assay kit, according to the instructions supplied from manufacturer. Stained cells were imaged by a fluorescence microscope (Eclipse TE2000; Nikon Instruments, Melville, NY, USA).
Hematoxylin and eosin (H&E) staining
FaDu cells (1×105 cells/mL) were grown in an 8-well chamber slide and allowed to adhere to the well for overnight. Cultured FaDu cells were then stimulated with 0, 10, or 20 µM DMC for 24 h at 37 ℃. Thereafter, the FaDu cells were washed three times by phosphate-buffered saline (PBS) at 4 ℃ and fixed with 4% paraformaldehyde for 30 min at 4 ℃. H&E staining was then performed to investigate the morphological alteration of FaDu cells induced by DMC. The cells were observed and imaged by a microscope (Leica DM750, Leica Microsystems, Heerbrugg, Switzerland).
Nuclear staining
DAPI (4,6-diamidino-2-phenylindole dihydrochloride) staining was performed to investigate the chromatin condensation of FaDu cells stimulated with DMC. FaDu cells (1×105 cells/mL) were grown in an 8-well chamber slide and allowed to adhere to the well overnight. Cultured FaDu cells were stimulated with 0, 10, or 20 µM DMC for 24 h at 37 ℃. Thereafter, the FaDu cells were rinsed three times by PBS at 4 ℃. The cells were stained with 1 mg/mL DAPI (Sigma-Aldrich) for 20 min. Nuclear condensation was observed and imaged by a fluorescence microscope (Eclipse TE200; Nikon Instruments).
Flow cytometry analysis (FACS)
FACS was performed by annexin V-FITC and propidium iodide (PI) (Cell Signaling Technology, Danvers, MA, USA) to investigate the alteration of apoptotic populations in the FaDu cells stimulated with DMC. FaDu cells (5 × 105 cells/mL) were grown on a 6-well culture plate for 24 h and then stimulated with 0, 10, or 20 µM DMC for 24 h. The cells were collected, washed with ice-cold PBS, and resuspended in 1× binding buffer (BD Biosciences, San Diego, CA, USA). Thereafter, annexin V-FITC and PI were added to the cells, followed by a 15-min incubation at 37 °C. The apoptotic populations were analyzed by BD Cell Quest® version 3.3 (Becton Dickinson, San José, CA, USA).
Western blot
FaDu cells (5×105 cells/mL) were grown in a 6-well culture plate and stimulated with DMC. Thereafter, cell lysates were extracted by cell lysis buffer (Cell Signaling Technology), according to the manufacturer’s instructions. The concentration of total proteins extracted from each FaDu cells was determined by a bicinchoninic acid protein assay (Thermo Fisher Scientific). An equal concentration of each total proteins was electrophoresed using sodium dodecyl sulfate polyacrylamide gel electrophoresis (SDS-PAGE), and then western blot was performed. The following antibodies were used and purchased from Santa Cruz Biotechnology Inc. (Dallas, TX, USA): antibodies against Fas Ligand (FasL, Cat No. sc-1968), caspase-3 (Cat No. sc-65497), caspas-8 (Cat No. sc-81656), caspase-9 (Cat No. sc-56076), B-cell lymphoma-2 (Bcl-2, Cat No. sc-7382), B-cell lymphoma extra-large (Bcl-xL, Cat No. sc-8392), Bcl-2-associated X protein (Bax, Cat No. sc-23959), Bcl-2 associated agonist of cell death (Bad, Cat No. sc-8044), Poly(ADP-ribose) polymerase (PARP, Cat No. sc-74470), β-actin (Cat No. sc-47778), phospho-nuclear factor kappa B (NF-κB, Cat No. Cat No. sc-135769), total NF-κB (Cat No. sc-71675) and Lamin B1 (Cat No. sc-374015). The immunoreactive bands were visualized by the ECL System (Amersham Biosciences, Piscataway, NJ, USA) and exposed by MicorChemi 4.2 (Dong-iL SHIMADZE Crop., Seoul, Republic of Korea).
Caspase-3/-7 activity assay
FaDu cells (1×105 cells/mL) were grown in an 8-well chamber slide and allowed to adhere to the wells for overnight. Cultured FaDu cells were stimulated with 0, 10, or 20 µM DMC for 24 h at 37 ℃. The activity of caspase-3/-7 was assessed by the cell-permeable fluorogenic substrate PhiPhiLux-G1D2 (OncoImmunin Inc.; Gaithersburg, MD, USA), according to the instructions supplied from manufacturer. Thereafter, the activity of caspase-3/-7 was imaged by fluorescence microscopy (Eclipse TE200; Nikon Instruments).
Nucleus translocation
FaDu cells (1×105 cells/mL) were grown in an 8-well chamber slide and allowed to adhere to the wells for overnight. Cultured cells were stimulated with 0, 10, or 20 µM DMC for 24 h at 37 ℃. Thereafter, FaDu cells were fixed with 1% paraformaldehyde, permeabilized in 0.2% Triton X-100, and extensively rinsed three times by PBS. To blockage non-specific signals, FaDu cells were stimulated with normal goat serum for 30 min at room temperature. After multiple washes, FaDu cells were incubated with rabbit anti-NF-κB antibody, followed by incubation with FITC-conjugated goat anti-rabbit IgG (Thermo Fisher Scientific) for overnight at 4 ℃. The stained FaDu cells were imaged by a laser confocal scanning microscope system (Leica Microsystems) at the Gwangju branch of the Korea Basic Science Institute (Gwangju, Republic of Korea).
Statistical analysis
The experimental data are expressed as the mean ± standard deviation from at least three independent experiments and were compared using analysis of variance (ANOVA), followed by Student’s t-test. A P value of less than 0.05 was considered statistically significant.
Results
DMC decreases the viability and survival of FaDu cells
FaDu cells were stimulated with 0, 1, 5, 20, 50, and 100 µM DMC for 24 h. Thereafter, MTT assay were performed to verify the viability and survival of FaDu cells. The results of the MTT assay showed that relative cell viabilities were measured by 101.14%±0.6%, 85.7%±0.6%, 68.2%±1.6%, 30.1%±2.3%, and 21.5%±3.2% in the in the HNSCC stimulated with 0, 1, 10, 20, 50, and 100 µM DMC, respectively, compared with un stimulated control (100%±0.2%), as shown in Figure 2A. Furthermore, the IC50 value of DMC in FaDu cells was estimated to be 37.78±2 µM. As shown in Figure 2B, the results of Live/Dead cell staining showed that DMC decreased the number of FaDu cells in a dose-dependent manner. Moreover, the number of dead cells stained with red fluorescence was increased in FaDu cells stimulated with DMC compared with that in the control, as shown in Figure 2B. These data demonstrated that DMC induced FaDu cell death through an increase in cytotoxicity.
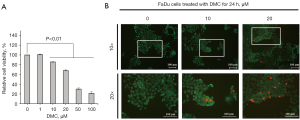
DMC-induced FaDu cell death has apoptotic characteristics
FaDu cells were grown in an 8 well-chamber slide and allowed to attach on the bottom for 24 h. Thereafter, FaDu cells were stimulated with 10 and 20 µM DMC to investigate morphological alterations and condensed chromatin by H&E staining and DAPI staining, respectively. As shown in Figure 3A, DMC not only decreased the number of FaDu cells, but also altered their morphology in a dose-dependent manner. Furthermore, DMC increased the number of FaDu cells with condensed chromatin, a representative characteristic of apoptotic cell death (Figure 3B). Sequentially, the caspase-3/-7 activity assay showed that DMC increased the activity of caspase-3 in FaDu cells, as shown in Figure 3C. Hence, these data consistently indicated that DMC-induced FaDu cell death was mediated by apoptosis.
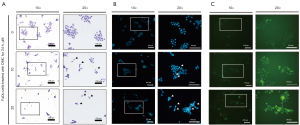
DMC induces apoptosis of FaDu cells
FaDu cells were stimulated with 10 and 20 µM DMC for 24 h. To determine whether DMC induced FaDu cell apoptosis, FACS analysis was performed by PI and Annexin-V. As depicted in Figure 4, the results of FACS analysis showed that the apoptotic population was estimated to be 12.6% and 24.69% in FaDu cells stimulated with 10 and 20 µM DMC, respectively, compared with the control (1.98%). Hence, these data consistently showed that DMC induced apoptosis of FaDu cells.
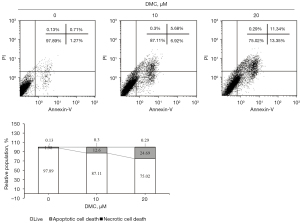
DMC-induced FaDu cell death was mediated by a cascade of caspase activation through death receptor-mediated extrinsic and mitochondria-dependent intrinsic apoptosis
Western blotting using specific antibodies associated with apoptosis was performed to determine the DMC-induced apoptotic pathway in FaDu cells, as shown in Figure 5. FasL expression was increased in the FaDu cells stimulated with DMC (Figure 5A). Furthermore, DMC upregulated the expression of pro- and cleaved caspase-8, a downstream target molecule of FasL, in FaDu cells, Sequentially, DMC-induced cleaved caspase-8 induced the upregulation of cleaved caspase-3 through the cleavage of pro-caspase-3. Furthermore, cleaved caspase-3 increased the expression of cleaved PARP to induce FaDu cell death, as shown in Figure 5C. In addition, the expression of Bcl-2 and Bcl-xL, anti-apoptotic factors associated with the mitochondria-dependent intrinsic apoptosis pathway, was decreased in FaDu cells stimulated with DMC. In contrast, the expression of pro-apoptotic factors such as Bax, Bad, and cleaved caspase-9 was upregulated in FaDu cells stimulated with DMC, as shown in Figure 5B. Sequentially, cleaved caspase-9 increased the expression of cleaved caspase-3 and cleaved PARP to induce FaDu cell death by DMC in a dose-dependent manner (Figure 5C). These data consistently indicated that DMC-induced FaDu cell death is not only mediated by the death receptor-mediated extrinsic apoptosis pathway, but also by the mitochondria-dependent intrinsic apoptosis pathway.
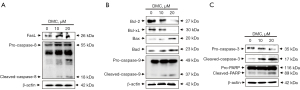
DMC induces apoptosis of FaDu cells through modulation of the NF-κB cellular signaling pathway
To investigate the cellular signaling pathways involved in DMC-induced apoptosis of FaDu cells, FaDu cells were stimulated with 10 and 20 µM DMC for 24 h. As shown in Figure 6A, DMC strongly suppressed NF-κB phosphorylation in FaDu cells. These data indicated that DMC inhibited the transition of NF-κB from the cytosol to the nucleus in FaDu cells. Hence, to verify the inhibition of NF-κB transition from cytosol to nucleus in FaDu cells, cytosolic and nuclear proteins were extracted from FaDu cells stimulated with 10 and 20 µM DMC for 24 h. As shown in Figure 6B, cytosolic NF-κB was increased in FaDu cells stimulated with DMC compared to the control cells. In contrast, NF-κB expression in the nucleus of FaDu cells stimulated with DMC was decreased by DMC in a dose-dependent manner. Furthermore, laser confocal scanning microscopic images showed that DMC inhibited the transition of NF-κB from the cytosol to the nucleus in FaDu cells (Figure 6C). Taken together, these data consistently indicated that DMC-induced apoptosis was mediated by the modulation of the NF-κB cellular signaling pathway in FaDu cells.
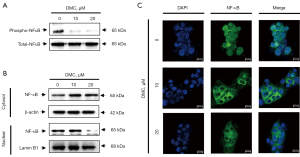
Discussion
Curcumin, a representative phenolic compound derived from Curcuma species, not only inhibits carcinogenic events such as proliferation, angiogenesis, and metastasis in various types of cancers, but also acts as a chemosensitizer by increasing the activity of other anti-tumor factors in cancers with multi-drug and chemotherapy resistance (2,9). Furthermore, curcumin not only induces apoptotic cell death through the modulation of the caspase-dependent death receptor pathway and mitochondrial pathway (10,11), but also decreases the survival of cancer cells through the modulation of tumor suppressor pathways and other cellular signaling pathways such as p53, mitogen-activated protein kinase, PI3K/Akt, and NF-κB (12-15). In addition, it has been shown that a dose of 8,000 mg/day curcumin is not toxic to humans in phase II clinical trial studies (16). Hence, curcumin is a representative chemotherapeutic compound derived from herbal plants. However, it has low oral bioavailability due to its poor solubility in water at acidic or neutral pH and instability in alkaline solutions (17). The low oral bioavailability of curcumin leads to cellular efflux by p-glycoprotein and its rapid excretion from the body after oral administration (18,19). Hence, there is a need for curcumin analogs with similar pharmacological properties and better oral biocompatibility for effective cancer treatment.
DMC is a curcumin analog with a similar chemical structure that lacks the methoxy group bound to the benzene ring (Figure 1). It has similar therapeutic effects, low toxicity, and relative safety compared to those of curcumin (17,20). However, this minor chemical difference in DMC makes it not only more stable than curcumin, but also improves its chemical activity under physiological conditions, that is, pH >7.30 (17). In addition, recent studies have reported DMC-induced anti-cancer activities such as cell apoptosis, inhibition of proliferation, migration, and invasion in various types of cancers, including lung (21), skin (22), prostate (23), breast (24) and cervical cancers (25). Similar to these studies, our results showed that DMC induced anti-cancer effects in FaDu cells.
In this study, DMC effectively decreased the survival of FaDu cells in a dose-dependent manner. Moreover, 37.78±2 µM DMC in FaDu cells stimulated for 24 h was estimated to be IC50 value, as shown in Figure 2. However, the IC50 values of DMC were estimated to be different depending on the type of cancer. For example, IC50 values of DMC were estimated to be 12, 54.5, and 111.6 µM in human cervical cancer (HeLa cell) (26), human osteosarcoma cells (MG-63), and prostate cancer (PC3 cells) (23), respectively.
For over three decades, cancer therapy strategies have focused on cancer-specific targeted apoptosis, which is a programmed cell death process mediated by several cellular signaling pathways, to promote the effective elimination of cancer cells (27). Generally, hallmarks of apoptosis are DNA fragmentation, chromatin condensation, and cellular blebbing (28,29). In this study, DMC not only decreased the number of cells, but also increased the number of cells with cellular blebbing and condensed chromatin (Figure 3). Hence, FACS analysis to verify whether DMC-induced FaDu cell death is mediated by apoptosis showed an increase in apoptotic populations in a dose-dependent manner (Figure 4). Taken together, these data consistently indicated that DMC-induced FaDu cell death is mediated by apoptosis.
These apoptotic hallmarks are mainly two mechanisms that are extrinsic pathways triggered by the Fas death receptor and the intrinsic pathway, resulting in the release of cytochrome c from mitochondria (29,30). Furthermore, both pathways are dependent on the activation of caspases that cleave regulatory and structural molecules during cell death (31). Recent studies have reported that DMC induces apoptosis by activating caspase signaling in various types of cancers, including skin cancer cells (22), cisplatin-resistant non-small lung cancer cells (32), oral squamous cell carcinoma cells (33), and human glioma U87 MG cells (34). In the present study, apoptosis of FaDu cells stimulated with DMC was triggered by the upregulation of FasL, which activates caspase-8, an extrinsic apoptosis pathway (Figure 5A). Furthermore, the activation of caspase-8 in FaDu cells stimulated with DMC induced the cleavage of Bid to truncated Bid, an initiator of the mitochondria-dependent intrinsic apoptosis pathway, to upregulate the expression of pro-apoptotic factors, including Bax and Bad, which form micropores in the mitochondrial outer membrane (Figure 5B). In contrast, anti-apoptotic factors such as Bcl-2 and Bcl-xL, which have a strong affinity for Bad, were decreased in FaDu cells stimulated with DMC. These events synergistically promoted the increase in mitochondrial outer membrane permeabilization (MOMP). Sequentially, cytochrome c, which activates caspase-9, was released into the cytosol from the mitochondrial outer membrane. Both activated caspase-8 and -9 induced the cleavage of caspase-3, a crucial executor caspase that is essential for chromatin condensation and DNA fragmentation in apoptotic cell death. Thereafter, activated caspase-3 induced the cleavage of PARP, which is involved in lysis and cell death through ATP depletion, in an attempt to repair the damaged DNA (Figure 3C and Figure 5C). Taken together, these findings indicated that DMC-induced FaDu cell death was mediated by both the FasL-induced extrinsic and the mitochondria-dependent intrinsic apoptosis pathways.
Generally, NF-κB is closely associated with oncogenesis through the regulation of gene expression associated with the development and progression of cancer such as proliferation, migration and apoptosis (35). Hence, aberrant or constitutive activation of NF-κB has been detected in many human malignancies (36-38). Especially, NF-κB is closely associated with transcription of genes involved in the suppression of cell death by both death receptor mediated extrinsic and mitochondria dependent intrinsic apoptosis pathways. The phosphorylation of NF-κB may upregulate the expression of interfering proteins such as FLICE-like inhibitory protein (FLIP) that prevent caspase-8 recruitment to death-inducing signaling complex (35,39,40). Hence, the inhibition of NF-κB phosphorylation induces the death receptor mediated extrinsic apoptosis through the suppression of FLIP expression. Furthermore, NF-κB induces the expression of anti-apoptotic factors such as the inhibitors of apoptosis (IAPs) and Bcl-2 family. IAPs such as c-IAP1, c-IAP2, and XIAP suppress apoptotic cell death through directing inhibition of caspases such as caspase-3 and caspase-9 (41-43). Bcl-2 family increase the mitochondrial outer membrane permeabilization (MOMP) through antagonize the function of pro-apoptotic factor such as Bad (44,45). These are indicating that the suppression of NF-κB phosphorylation is closely associated with the induction of apoptotic cell death that is mediated by the mitochondria dependent apoptosis pathway.
Hence, the suppression of NF-κB phosphorylation has been considered as a strategy for cancer therapy. Several studies have reported that curcumin-induced apoptosis is mediated by the inhibition of the NF-κB cellular signaling pathway in various types of cancers (46-48). Similar to curcumin, recent studies have shown that DMC-induced apoptosis is mediated by the inhibition of NF-κB phosphorylation in various types of cancers (34,49,50). In the present study, DMC significantly inhibited the translocation of cytosolic NF-κB to the nucleus through the inhibition of NF-κB phosphorylation in FaDu cells (Figure 6). Hence, these data indicated that DMC-induced FaDu cell apoptosis was mediated by the modulation of the NF-κB cellular signaling pathway.
In conclusion, as shown in Figure 7, DMC-induced cell death was mediated through the caspase-dependent apoptosis pathway involved in the inhibition of NF-κB activation in FaDu cells. Therefore, DMC may have potential as a novel anticancer agent for the treatment of HNSCC.
Acknowledgments
Funding: This study was supported by research fund from Chosun University, 2020.
Footnote
Reporting Checklist: The authors have completed the MDAR reporting checklist. Available at https://tcr.amegroups.com/article/view/10.21037/tcr-21-2410/rc
Peer Review File: Available at https://tcr.amegroups.com/article/view/10.21037/tcr-21-2410/prf
Conflicts of Interest: All authors have completed the ICMJE uniform disclosure form (available at https://tcr.amegroups.com/article/view/10.21037/tcr-21-2410/coif). The authors have no conflicts of interest to declare.
Ethical Statement: The authors are accountable for all aspects of the work in ensuring that questions related to the accuracy or integrity of any part of the work are appropriately investigated and resolved.
Open Access Statement: This is an Open Access article distributed in accordance with the Creative Commons Attribution-NonCommercial-NoDerivs 4.0 International License (CC BY-NC-ND 4.0), which permits the non-commercial replication and distribution of the article with the strict proviso that no changes or edits are made and the original work is properly cited (including links to both the formal publication through the relevant DOI and the license). See: https://creativecommons.org/licenses/by-nc-nd/4.0/.
References
- Naveen Kumar DR, Cijo George V, Suresh PK, et al. Cytotoxicity, apoptosis induction and anti-metastatic potential of Oroxylum indicum in human breast cancer cells. Asian Pac J Cancer Prev 2012;13:2729-34. [Crossref] [PubMed]
- Safarzadeh E, Sandoghchian Shotorbani S, Baradaran B. Herbal medicine as inducers of apoptosis in cancer treatment. Adv Pharm Bull 2014;4:421-7. [PubMed]
- de Araújo Júnior RF, de Souza TP, Pires JG, et al. A dry extract of Phyllanthus niruri protects normal cells and induces apoptosis in human liver carcinoma cells. Exp Biol Med (Maywood) 2012;237:1281-8. [Crossref] [PubMed]
- Pricci M, Girardi B, Giorgio F, et al. Curcumin and Colorectal Cancer: From Basic to Clinical Evidences. Int J Mol Sci 2020;21:2364. [Crossref] [PubMed]
- Wang Y, Yu J, Cui R, et al. Curcumin in Treating Breast Cancer: A Review. J Lab Autom 2016;21:723-31. [Crossref] [PubMed]
- Bahrami A. A Ferns G. Effect of Curcumin and Its Derivates on Gastric Cancer: Molecular Mechanisms. Nutr Cancer 2021;73:1553-69. [Crossref] [PubMed]
- Termini D, Den Hartogh DJ, Jaglanian A, et al. Curcumin against Prostate Cancer: Current Evidence. Biomolecules 2020;10:1536. [Crossref] [PubMed]
- Kim JY, Cho TJ, Woo BH, et al. Curcumin-induced autophagy contributes to the decreased survival of oral cancer cells. Arch Oral Biol 2012;57:1018-25. [Crossref] [PubMed]
- Qi F, Li A, Inagaki Y, et al. Chinese herbal medicines as adjuvant treatment during chemo- or radio-therapy for cancer. Biosci Trends 2010;4:297-307. [PubMed]
- Anto RJ, Mukhopadhyay A, Denning K, et al. Curcumin (diferuloylmethane) induces apoptosis through activation of caspase-8, BID cleavage and cytochrome c release: its suppression by ectopic expression of Bcl-2 and Bcl-xl. Carcinogenesis 2002;23:143-50. [Crossref] [PubMed]
- Wu SH, Hang LW, Yang JS, et al. Curcumin induces apoptosis in human non-small cell lung cancer NCI-H460 cells through ER stress and caspase cascade- and mitochondria-dependent pathways. Anticancer Res 2010;30:2125-33. [PubMed]
- Wu B, Yao H, Wang S, et al. DAPK1 modulates a curcumin-induced G2/M arrest and apoptosis by regulating STAT3, NF-κB, and caspase-3 activation. Biochem Biophys Res Commun 2013;434:75-80. [Crossref] [PubMed]
- Larasati YA, Yoneda-Kato N, Nakamae I, et al. Curcumin targets multiple enzymes involved in the ROS metabolic pathway to suppress tumor cell growth. Sci Rep 2018;8:2039. [Crossref] [PubMed]
- Fu H, Wang C, Yang D, et al. Curcumin regulates proliferation, autophagy, and apoptosis in gastric cancer cells by affecting PI3K and P53 signaling. J Cell Physiol 2018;233:4634-42. [Crossref] [PubMed]
- Lim W, Jeong M, Bazer FW, et al. Curcumin Suppresses Proliferation and Migration and Induces Apoptosis on Human Placental Choriocarcinoma Cells via ERK1/2 and SAPK/JNK MAPK Signaling Pathways. Biol Reprod 2016;95:83. [Crossref] [PubMed]
- Ramachandran C, Rodriguez S, Ramachandran R, et al. Expression profiles of apoptotic genes induced by curcumin in human breast cancer and mammary epithelial cell lines. Anticancer Res 2005;25:3293-302. [PubMed]
- Hatamipour M, Ramezani M, Tabassi SAS, et al. Demethoxycurcumin: A naturally occurring curcumin analogue for treating non-cancerous diseases. J Cell Physiol 2019;234:19320-30. [Crossref] [PubMed]
- Chuah AM, Jacob B, Jie Z, et al. Enhanced bioavailability and bioefficacy of an amorphous solid dispersion of curcumin. Food Chem 2014;156:227-33. [Crossref] [PubMed]
- Zhang X, Li LC, Mao S. Nanosuspensions of poorly water soluble drugs prepared by top-down technologies. Curr Pharm Des 2014;20:388-407. [Crossref] [PubMed]
- Shi L, Sun G, Zhang Y. Demethoxycurcumin analogue DMC-BH exhibits potent anticancer effects on orthotopic glioblastomas. Aging (Albany NY) 2020;12:23795-807. [Crossref] [PubMed]
- Lin CY, Hung CC, Wang CCN, et al. Demethoxycurcumin sensitizes the response of non-small cell lung cancer to cisplatin through downregulation of TP and ERCC1-related pathways. Phytomedicine 2019;53:28-36. [Crossref] [PubMed]
- Wu Y, Zhang P, Yang H, et al. Effects of demethoxycurcumin on the viability and apoptosis of skin cancer cells. Mol Med Rep 2017;16:539-46. [Crossref] [PubMed]
- Ni X, Zhang A, Zhao Z, et al. Demethoxycurcumin inhibits cell proliferation, migration and invasion in prostate cancer cells. Oncol Rep 2012;28:85-90. [PubMed]
- Yodkeeree S, Ampasavate C, Sung B, et al. Demethoxycurcumin suppresses migration and invasion of MDA-MB-231 human breast cancer cell line. Eur J Pharmacol 2010;627:8-15. [Crossref] [PubMed]
- Chueh FS, Lien JC, Chou YC, et al. Demethoxycurcumin Inhibits In Vivo Growth of Xenograft Tumors of Human Cervical Cancer Cells. In Vivo 2020;34:2469-74. [Crossref] [PubMed]
- Lin CC, Kuo CL, Huang YP, et al. Demethoxycurcumin Suppresses Migration and Invasion of Human Cervical Cancer HeLa Cells via Inhibition of NF-κB Pathways. Anticancer Res 2018;38:2761-9. [PubMed]
- Carneiro BA, El-Deiry WS. Targeting apoptosis in cancer therapy. Nat Rev Clin Oncol 2020;17:395-417. [Crossref] [PubMed]
- Yanumula A, Cusick JK. Biochemistry, Extrinsic Pathway of Apoptosis. StatPearls. Treasure Island (FL) 2021.
- Hockenbery D, Nuñez G, Milliman C, et al. Bcl-2 is an inner mitochondrial membrane protein that blocks programmed cell death. Nature 1990;348:334-6. [Crossref] [PubMed]
- Zapata JM, Pawlowski K, Haas E, et al. A diverse family of proteins containing tumor necrosis factor receptor-associated factor domains. J Biol Chem 2001;276:24242-52. [Crossref] [PubMed]
- Ghobrial IM, Witzig TE, Adjei AA. Targeting apoptosis pathways in cancer therapy. CA Cancer J Clin 2005;55:178-94. [Crossref] [PubMed]
- Chen Y, Hong C, Chen X, et al. Demethoxycurcumin increases the sensitivity of cisplatin-resistant non-small lung cancer cells to cisplatin and induces apoptosis by activating the caspase signaling pathway. Oncol Lett 2020;20:209. [Crossref] [PubMed]
- Chien MH, Yang WE, Yang YC, et al. Dual Targeting of the p38 MAPK-HO-1 Axis and cIAP1/XIAP by Demethoxycurcumin Triggers Caspase-Mediated Apoptotic Cell Death in Oral Squamous Cell Carcinoma Cells. Cancers (Basel) 2020;12:703. [Crossref] [PubMed]
- Kumar R, Lal N, Nemaysh V, et al. Demethoxycurcumin mediated targeting of MnSOD leading to activation of apoptotic pathway and inhibition of Akt/NF-κB survival signalling in human glioma U87 MG cells. Toxicol Appl Pharmacol 2018;345:75-93. [Crossref] [PubMed]
- Dolcet X, Llobet D, Pallares J, et al. NF-kB in development and progression of human cancer. Virchows Arch 2005;446:475-82. [Crossref] [PubMed]
- Beauparlant P, Kwan I, Bitar R, et al. Disruption of I kappa B alpha regulation by antisense RNA expression leads to malignant transformation. Oncogene 1994;9:3189-97. [PubMed]
- Berenson JR, Ma HM, Vescio R. The role of nuclear factor-kappaB in the biology and treatment of multiple myeloma. Semin Oncol 2001;28:626-33. [Crossref] [PubMed]
- Ferreira CG, Epping M, Kruyt FA, et al. Apoptosis: target of cancer therapy. Clin Cancer Res 2002;8:2024-34. [PubMed]
- Kreuz S, Siegmund D, Scheurich P, et al. NF-kappaB inducers upregulate cFLIP, a cycloheximide-sensitive inhibitor of death receptor signaling. Mol Cell Biol 2001;21:3964-73. [Crossref] [PubMed]
- Micheau O, Lens S, Gaide O, et al. NF-kappaB signals induce the expression of c-FLIP. Mol Cell Biol 2001;21:5299-305. [Crossref] [PubMed]
- Deveraux QL, Roy N, Stennicke HR, et al. IAPs block apoptotic events induced by caspase-8 and cytochrome c by direct inhibition of distinct caspases. EMBO J 1998;17:2215-23. [Crossref] [PubMed]
- Takahashi R, Deveraux Q, Tamm I, et al. A single BIR domain of XIAP sufficient for inhibiting caspases. J Biol Chem 1998;273:7787-90. [Crossref] [PubMed]
- Wang CY, Mayo MW, Korneluk RG, et al. NF-kappaB antiapoptosis: induction of TRAF1 and TRAF2 and c-IAP1 and c-IAP2 to suppress caspase-8 activation. Science 1998;281:1680-3. [Crossref] [PubMed]
- Chen QM, Tu VC. Apoptosis and heart failure: mechanisms and therapeutic implications. Am J Cardiovasc Drugs 2002;2:43-57. [Crossref] [PubMed]
- Lee JU, Hosotani R, Wada M, et al. Role of Bcl-2 family proteins (Bax, Bcl-2 and Bcl-X) on cellular susceptibility to radiation in pancreatic cancer cells. Eur J Cancer 1999;35:1374-80. [Crossref] [PubMed]
- Ghasemi F, Shafiee M, Banikazemi Z, et al. Curcumin inhibits NF-kB and Wnt/β-catenin pathways in cervical cancer cells. Pathol Res Pract 2019;215:152556. [Crossref] [PubMed]
- Divya CS, Pillai MR. Antitumor action of curcumin in human papillomavirus associated cells involves downregulation of viral oncogenes, prevention of NFkB and AP-1 translocation, and modulation of apoptosis. Mol Carcinog 2006;45:320-32. [Crossref] [PubMed]
- Singh S, Khar A. Biological effects of curcumin and its role in cancer chemoprevention and therapy. Anticancer Agents Med Chem 2006;6:259-70. [Crossref] [PubMed]
- Huang TY, Hsu CW, Chang WC, et al. Demethoxycurcumin Retards Cell Growth and Induces Apoptosis in Human Brain Malignant Glioma GBM 8401 Cells. Evid Based Complement Alternat Med 2012;2012:396573. [Crossref] [PubMed]
- Lal N, Nemaysh V, Luthra PM. Proteasome mediated degradation of CDC25C and Cyclin B1 in Demethoxycurcumin treated human glioma U87 MG cells to trigger G2/M cell cycle arrest. Toxicol Appl Pharmacol 2018;356:76-89. [Crossref] [PubMed]