Optimal long peptide for flagellin-adjuvanted HPV E7 cancer vaccine to enhance tumor suppression in combination with anti-PD-1
Introduction
Cancer immunotherapy, a treatment that exploits the host immune system to fight cancer, has become the first-line therapeutic option for a variety of cancers (1). Therapeutic cancer vaccines that elicit a new immune response or amplify an existing tumor antigen (Ag)-specific immune responses are continuously being developed (2-8). And cancer vaccine-mediated tumor cell killing results in epitope spreading and subsequent expansion of tumor-specific immune responses (6). Moynihan et al. reported that multiple combinations of immunotherapies elicit endogenous immunity to eradicate large established tumors, and the cancer vaccine is a critical component of successful therapy (9). The development of immune checkpoint inhibitor (ICI) therapy ushered in a fundamental shift in cancer treatment. Most types of cancer have been treated with ICIs using a variety of drugs from different manufacturers (1,6). Given that personalized neoantigen-based vaccines developed with efficient sequencing and bioinformatics tools have demonstrated potent tumor-specific immunogenicity (6,10), therapeutic cancer vaccines are expected to be an essential component of cancer immunotherapy. Current ICIs can be combined with other new ICIs, immunotherapies, and delivery strategies (11). Therefore, a successful cancer vaccine platform was crucially awaiting to establish a new combination strategy with ICI.
Cancer vaccines are administered to humans in cell-, viral vector-, nucleic acid- or peptide-based forms (3,12-16). Because of their ease of use, several forms of short (8–10 amino acids) or long (longer than 30 amino acids) peptide-based cancer vaccines have been tested in preclinical or clinical settings (2,4,9,15,17,18). However, the efficacy of therapeutic cancer vaccines remains disappointing. Short peptides (SPs) bind directly to surface MHC I molecules without processing in antigen-presenting cells (APCs), and the vast majority of administered short peptides are pharmacokinetically eliminated in vivo. In addition, short peptides administered in this way act on nonprofessional APCs and may mediate the induction of a tolerogenic response (3,14,19). In contrast to short peptides, long peptides (LPs) undergo internalization, processing, and MHC-restricted presentation by professional APCs (20). In addition, long peptides generally include CD4 and CD8 epitopes to induce efficient T cell activation (12,19,21). It has been demonstrated that human papillomavirus (HPV) 16 E6/E7 long peptide vaccines induce efficacy superior to that of the short peptide vaccines (22-24). The ideal therapeutic cancer vaccine should induce long-term antitumor immune responses and overcome the tolerogenic tumor microenvironment (TME).
We previously reported that bacterial flagellin, Vibrio vulnificus FlaB, exhibits excellent adjuvant activity for various antigens (25,26) and that coadministration of FlaB with HPV 16 E6/E7 short peptide induces tumor-specific immune responses through TLR5 stimulation in therapeutic cancer vaccine models (27,28). Furthermore, we suggested that engineered Salmonella bacteria secreting heterogeneous bacterial flagellin have a strong suppressive effect on the growth of both primary and metastatic tumors mediated through phenotypic and functional maturation of intratumoral macrophages via a shift toward an M1 phenotype and a reduction in M2-like suppressive activity (29). The strategy of administering cancer vaccines with pharmacokinetic advantages is thought to increase the in vivo availability of the vaccine.
In this study, we investigated the basic requirements of peptide-based antigens for the development of a flagellin-adjuvanted cancer vaccine in an HPV16 E7 vaccine model using TC-1 implantation experiments. We showed that compared to the E7-SP, E7-LP20, and E7 proteins, the E7-LP35 (amino acids 43-77) peptide is an optimal antigen for a flagellin-adjuvanted cancer vaccine (FlaB-Vax) in a mouse TC-1 tumor model. Flagellin was the essential component of this E7 peptide-based therapeutic cancer vaccine. In addition, PD-1 blockade enhanced the therapeutic efficacy of the flagellin-adjuvanted E7-LP35 vaccine (FlaB-LP35Vax) but not FlaB-SPVax. In summary, we evaluated an ideal peptide antigen for flagellin-adjuvanted HPV E7 cancer vaccines to potentiate anti-PD-1-mediated tumor suppression. We present the following article in accordance with the ARRIVE reporting checklist (available at https://tcr.amegroups.com/article/view/10.21037/tcr-21-2798/rc).
Methods
Peptides and recombinant proteins
The E7-SP (amino acids 49-57: RAHYNIVTF), E7-LP20 (amino acids 43-62: GQAEPDRAHYNIVTFCCKCD), and E7-LP35 (amino acids 43-77: GQAEPDRAHYNIVTFCCKCDSTLRLCVQSTHV) peptides from HPV16 (Figure 1A) were synthesized by AnyGen (Gwangju, Korea) with a purity of >95%.
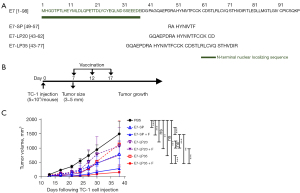
Tumor implantation and treatments
Seven- to eight-week-old female SPF C57BL/6 mice were purchased from the ORIENT (Seongnam-si, Gyeonggi-do, Korea). TC-1 cells were cultured in RPMI 1640 medium supplemented with 10% heat-inactivated fetal bovine serum (FBS), 100 units/mL penicillin, and 100 µg/mL streptomycin (Gibco, NY, USA) at 37 ℃ and 5% CO2. Tumors were established in mice by subcutaneous injection of 5×104 TC-1 cells in 100 µL of phosphate-buffered saline (PBS) into the right mid flank of each mouse (n=5). When the tumor diameter reached approximately 3–5 mm, the tumor-bearing mice were randomly assigned to different treatment groups (PBS, E7-SP, E7-SP+F, E7-LP20, E7-LP20+F, E7-LP35, and E7-LP35+F) (Figure 1B). The tumor-bearing mice were then peritumorally vaccinated with 200 µL of PBS (PBS group; n=5), PBS containing 100 µg of E7-SP (E7-SP group; n=10), 100 µg of E7-SP plus 4 µg of FlaB (E7-SP+F group; n=10), 100 µg of E7-LP20 (E7-LP20 group; n=5), 100 µg of E7-LP20 plus 4 µg of FlaB (E7-LP20+F group; n=5), 150 µg of E7-LP35 (E7-LP35 group; n=10), or 150 µg E7-LP35 plus 4 µg of FlaB (E7-LP+F group; n=10) under anesthesia intraperitoneal (i.p.) infection of diluted Zoletil®; tiletamine, zolazepam and Rompun®) at five-day intervals (Figure 1A). Tumor growth was monitored every 3 days. The tumor volume was calculated with the formula V = (tumor length) × (tumor width) × (tumor height) /2 as previously described (11,27,29).
For experiments exploring combinations with anti-PD1 therapy, when the tumor diameter reached approximately 2–3 mm, the vaccinated tumor-bearing mice were administered an anti-PD1 mAb following the experimental schedule (n=5/group). The anti-PD1 antibody was administered by i.p. injection in 200 µL of PBS containing 100 µg of anti-PD1 antibody (clone RMP1-14, BioXCell, Lebanon, USA) one day after each vaccination.
Statistical analyses
Results are expressed as mean ± standard error of mean (SEM) values, unless otherwise specified. Statistical significance between two groups were determined by the Mann-Whitney test. Log-rank (Mantel-Cox) tests and two-way ANOVA were used to compare survival and tumor volume, respectively. Statistical data analysis was carried out using the Prism 8.00 software for Windows (GraphPad Software, San Diego, CA, USA). P values of <0.05 were considered statistically significant.
Ethical statement
All animal experimental procedures were performed with approval from the Chonnam National University Institutional Animal Care and Use Committee under protocol CNU IACUC-H-2020-31. Animal research facility maintenance and experimental procedures were carried out in strict accordance with the guidelines of the Animal Welfare Act legislated by the Korean Ministry of Agriculture, Food and Rural Affairs.
Results
The E7-LP35 peptide is an optimal antigen for a flagellin-adjuvanted cancer vaccine (FlaB-Vax) in a mouse TC-1 tumor model
To evaluate the best peptide antigen for a flagellin-adjuvanted therapeutic cancer vaccine for clinical use, we synthesized the HPV16 E7 short peptide (E7-SP; E749-57) (27,28), the HPV16 E7 long peptide with a length of 20 amino acids (E7-LP20; E743-62) (30), and the HPV16 E7 35 mer long peptide (E7-LP35; E743-77) (31) (Figure 1A). Based on the predictions of MHC I and MHC II binding to HPV16 E7 generated by the immune epitope database (IEDB) analysis resource (http://tools.iedb.org/mhci/ and https://tools.iedb.org/mhcii/), E7-SP49-57 exhibits the strongest binding to MHC I molecules (data not shown). E7-LP20 and E7-LP35 share MHC I (E749-57, E750-58, and E744-52) and MHC II (E743-57, E744-58, and E745-59) epitopes. Compared to E7-LP20, E7-LP35 has additional but weaker MHC II binder epitopes (E761-75, E762-76, and E763-77), with a lower binding prediction rank (data not shown). The E7 oncoprotein has been reported to contain multiple sites for interaction with known transcriptional regulators, and the N-terminal conserved region-1 (CR-1) and CR-2 of E7 (E71-37) are involved in the nuclear localization of the E7 protein (32,33). Thus, to avoid unexpected cellular responses induced by the N-terminal sequence, we did not select peptide antigen in E71-37. To establish the mouse tumor model, groups of C57BL/6 mice were implanted with TC-1 cells in the right mid flank as previously described (27). When the tumor size reached approximately 3–5 mm in diameter, the tumor-bearing mice were subcutaneously vaccinated with 200 µL of PBS only (n=5), 100 µg of E7-SP (n=10), 100 µg of E7-SP plus 4 µg of FlaB (E7-SP+F; n=10), 100 µg of E7-LP20 (n=5), 100 µg of E7-LP20 plus 4 µg of FlaB (E7-LP20+F; n=5), 150 µg of E7-LP35 (n=10), and 150 µg of E7-LP35 plus 4 µg of FlaB (E7-LP35+F; n=10) in the peritumoral region three times at five-day intervals (Figure 1B), and the tumor volume was measured.
As shown in Figure 1C, the E7-SP-vaccinated groups showed a significant level of tumor suppression (***, P<0.001 for PBS vs. SP). However, the groups vaccinated with E7-LP alone (E7-LP20 and E7-LP35) did not show significant tumor suppression, with larger error values across the samples compared to the errors in the E7-SP-vaccinated group (P>0.05 for PBS vs. E7-LP20; P>0.05 for PBS vs. E7-LP35). Coadministration of flagellin potentiated E7-SP- and E7-LP35-mediated tumor suppression (***, P<0.001 for E7-SP vs. E7-SP+F; ***, P<0.001 for E7-LP35 vs. E7-LP35+F). However, the adjuvant effect of flagellin was not observed in the group vaccinated with E7-LP20 (P>0.05 for E7-LP20 vs. E7-LP20 + FlaB). Notably, the E7-LP35+F group (n=10) showed more prominent antitumor activity than the E7-SP+F (**, P<0.01 for E7-LP35+F vs. E7-SP+F) group. These results indicate that flagellin is an essential component in the cancer vaccine formulation and that E7-LP35 is an optimal antigen for the flagellin-adjuvanted cancer vaccine.
PD-1 blockade enhances the therapeutic efficacy of E7-LP35 + FlaB (FlaB-LP35Vax) but not FlaB-SPVax
T cell activation has been reported to be related to PD-1 expression and that IFNγ production is a potent signal for the induction of PD-L1 in tumor cells (34). Given that FlaB-Vax modulates immune cell activation and cytokine production (27,28), we further evaluated whether PD-1 blockade can enhance the therapeutic effect of FlaB-LP35Vax. Groups of C57BL/6 mice were implanted with TC-1 cells by subcutaneous injection into the right mid flank. When the tumor size reached approximately 2–3 mm in diameter, the tumor-bearing mice were then vaccinated with PBS, FlaB-SPVax (E7-SP+F), or FlaB-LP35Vax (E7-LP35+F) and treated with the anti-PD-1 antibody following the experimental schedule (Figure 2A). The survival of FlaB-LP35Vax-immunized mice was significantly improved by the anti-PD-1 antibody treatment (**, P<0.01; FlaB-LP35Vax+isotype vs. FlaB-LP35Vax+anti-PD-1). Notably, PD-1 blockade did not enhance the therapeutic efficacy of FlaB-SPVax (P>0.05; FlaB-SPVax + isotype vs. FlaB-SPVax +anti-PD-1) (Figure 2B). Consistent with the survival data, FlaB-LP35Vax+anti-PD-1 antibody-treated mice showed 60% tumor regression 55 days after tumor cell implantation (Figure 2B). This result suggests that FlaB-LP35Vax is the optimal vaccine formulation for combination with ICI therapy in clinical application.
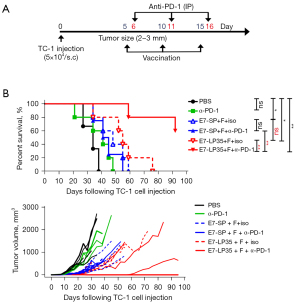
Conclusions
For successful cancer immunotherapy, selective and efficient engagement of T cells with cancer cells is required. Therapeutic cancer vaccines directed against tumor-specific antigens (TSAs) or tumor-associated antigens (TAAs) are active immunotherapy approaches to induce antitumor immune responses. The development of improved approaches focused on previous unsuccessful experiences should be continued. And more understanding about what is critical for the successful cancer vaccine is needed. In the present study, we clearly showed that E7-LP35 is an optimal peptide antigen for HPV E7. MHC-I binding short peptides (9-10 amino acids) have intrinsic limitations for use in clinical settings (19). Administered short peptides directly bind to surface MHC I molecules without antigen processing by APCs. Some short peptides that interact with nonprofessional APCs, including T cells and B cells, may induce tolerogenic responses. Therefore, improved peptide-based cancer vaccines should be formulated with optimally immunogenic antigens to induce long-term anticancer activity. It has been reported that MHC class II-restricted neoantigens have a key function to orchestrate antitumor responses in a therapeutic cancer vaccine (7). The MHC I and MHC II binding predictions generated by the IEDB analysis resource showed that E7-SP (E749-57) binds strongly to MHC I and that E7-LP35 (E743-77) contains several CD8+ and CD4+ epitopes. E7-LP35 and E7-LP20 may undergo normal internalization, processing, and MHC-restricted presentation by professional APCs. In contrast to E7-LP35, the E7-LP20 antigen did not show significant tumor-suppressive effects, suggesting that the E7-LP20 antigen is not properly presented by APCs, unlike E7-LP35.
Adjuvant and or delivery strategies of neoantigen-based cancer vaccines are critical for successful anti-cancer immune responses (6,35). In our previous studies, we showed that the TLR5 agonist flagellin is an excellent adjuvant that induces effective cell-mediated immunity (CMI) against coadministered tumor antigens (27,28) and that flagellin-expressing bacteria can modify the TME from an immunosuppressive to an immunostimulatory state (29). As shown in Figure 1, flagellin is essential to induce therapeutic efficacy. Combinations take center stage in ICIs clinical trials in cancer treatments (36). HPV infection is related to most anogenital and oropharyngeal cancers (37). HPV 16 is the primary oncogenic virus, and HPV E7, which plays a central role in the HPV life cycle, is a representative TSA. The HPV E7 protein, a transcriptional regulator modulating the retinoblastoma protein (pRb)/E2F system, is constitutively expressed in most HPV-induced neoplasias (32,38). The combination of ICIs with a tumor-specific vaccine showed promising clinical outcomes in patients with HPV-16-related cancers (39). Efficacious combinations of immunotherapies should potentiate immune responses in the TME (40). The selection of optimal peptide-based tumor antigens in a variety of cancer vaccine platforms may enhance antigen-specific antitumor immune responses.
Acknowledgments
The authors are grateful to Ms. Myeung Suk Kim for her excellent assistance in animal experiments.
Funding: JHR was supported by the National Research Foundation of Korea (NRF) grant funded by the Korea government (MSIT) (No. 2020R1A5A2031185). SEL was supported by the National Research Foundation of Korea (NRF) grant funded by the Korea government (MSIT) (No. 2019R1A2B5B01069981).
Footnote
Reporting Checklist: The authors have completed the ARRIVE reporting checklist. Available at https://tcr.amegroups.com/article/view/10.21037/tcr-21-2798/rc
Data Sharing Statement: Available at https://tcr.amegroups.com/article/view/10.21037/tcr-21-2798/dss
Conflicts of Interest: All authors have completed the ICMJE uniform disclosure form (available at https://tcr.amegroups.com/article/view/10.21037/tcr-21-2798/coif). JHR was supported by the National Research Foundation of Korea (NRF) grant funded by the Korea government (MSIT) (No. 2020R1A5A2031185). SEL was supported by the National Research Foundation of Korea (NRF) grant funded by the Korea government (MSIT) (No. 2019R1A2B5B01069981). The other authors have no conflicts of interest to declare.
Ethical Statement: The authors are accountable for all aspects of the work in ensuring that questions related to the accuracy or integrity of any part of the work are appropriately investigated and resolved. All animal experimental procedures were performed with approval from the Chonnam National University Institutional Animal Care and Use Committee under protocol CNU IACUC-H-2020-31. Animal research facility maintenance and experimental procedures were carried out in strict accordance with the guidelines of the Animal Welfare Act legislated by the Korean Ministry of Agriculture, Food and Rural Affairs.
Open Access Statement: This is an Open Access article distributed in accordance with the Creative Commons Attribution-NonCommercial-NoDerivs 4.0 International License (CC BY-NC-ND 4.0), which permits the non-commercial replication and distribution of the article with the strict proviso that no changes or edits are made and the original work is properly cited (including links to both the formal publication through the relevant DOI and the license). See: https://creativecommons.org/licenses/by-nc-nd/4.0/.
References
- Upadhaya S, Hubbard-Lucey VM, Yu JX. Immuno-oncology drug development forges on despite COVID-19. Nat Rev Drug Discov 2020;19:751-2. [Crossref] [PubMed]
- Shemesh CS, Hsu JC, Hosseini I, et al. Personalized Cancer Vaccines: Clinical Landscape, Challenges, and Opportunities. Mol Ther 2021;29:555-70. [Crossref] [PubMed]
- Jou J, Harrington KJ, Zocca MB, et al. The Changing Landscape of Therapeutic Cancer Vaccines-Novel Platforms and Neoantigen Identification. Clin Cancer Res 2021;27:689-703. [Crossref] [PubMed]
- Buonaguro L, Tagliamonte M. Selecting Target Antigens for Cancer Vaccine Development. Vaccines (Basel) 2020;8:615. [Crossref] [PubMed]
- Li W, Jing Z, Wang S, et al. P22 virus-like particles as an effective antigen delivery nanoplatform for cancer immunotherapy. Biomaterials 2021;271:120726. [Crossref] [PubMed]
- Blass E, Ott PA. Advances in the development of personalized neoantigen-based therapeutic cancer vaccines. Nat Rev Clin Oncol 2021;18:215-29. [Crossref] [PubMed]
- Alspach E, Lussier DM, Miceli AP, et al. MHC-II neoantigens shape tumour immunity and response to immunotherapy. Nature 2019;574:696-701. [Crossref] [PubMed]
- Morse MA, Gwin WR 3rd, Mitchell DA. Vaccine Therapies for Cancer: Then and Now. Target Oncol 2021;16:121-52. [Crossref] [PubMed]
- Moynihan KD, Opel CF, Szeto GL, et al. Eradication of large established tumors in mice by combination immunotherapy that engages innate and adaptive immune responses. Nat Med 2016;22:1402-10. [Crossref] [PubMed]
- De Mattos-Arruda L, Vazquez M, Finotello F, et al. Neoantigen prediction and computational perspectives towards clinical benefit: recommendations from the ESMO Precision Medicine Working Group. Ann Oncol 2020;31:978-90. [Crossref] [PubMed]
- Hwang HS, Cherukula K, Bang YJ, et al. Combination of Photodynamic Therapy and a Flagellin-Adjuvanted Cancer Vaccine Potentiated the Anti-PD-1-Mediated Melanoma Suppression. Cells 2020;9:2432. [Crossref] [PubMed]
- Hu Z, Ott PA, Wu CJ. Towards personalized, tumour-specific, therapeutic vaccines for cancer. Nat Rev Immunol 2018;18:168-82. [Crossref] [PubMed]
- Kreiter S, Vormehr M, van de Roemer N, et al. Mutant MHC class II epitopes drive therapeutic immune responses to cancer. Nature 2015;520:692-6. [Crossref] [PubMed]
- Slingluff CL Jr. The present and future of peptide vaccines for cancer: single or multiple, long or short, alone or in combination? Cancer J 2011;17:343-50. [Crossref] [PubMed]
- Bahmani B, Amini-Bayat Z, Ranjbar MM, et al. HPV16-E7 Protein T Cell Epitope Prediction and Global Therapeutic Peptide Vaccine Design Based on Human Leukocyte Antigen Frequency: An In-Silico Study. Int J Pept Res Ther 2021;27:365-78. [Crossref] [PubMed]
- Peng S, Ferrall L, Gaillard S, et al. Development of DNA Vaccine Targeting E6 and E7 Proteins of Human Papillomavirus 16 (HPV16) and HPV18 for Immunotherapy in Combination with Recombinant Vaccinia Boost and PD-1 Antibody. mBio 2021;12:03224-20. [Crossref] [PubMed]
- Kumai T, Lee S, Cho HI, et al. Optimization of Peptide Vaccines to Induce Robust Antitumor CD4 T-cell Responses. Cancer Immunol Res 2017;5:72-83. [Crossref] [PubMed]
- van Poelgeest MI, Welters MJ, Vermeij R, et al. Vaccination against Oncoproteins of HPV16 for Noninvasive Vulvar/Vaginal Lesions: Lesion Clearance Is Related to the Strength of the T-Cell Response. Clin Cancer Res 2016;22:2342-50. [Crossref] [PubMed]
- Melief CJ, van Hall T, Arens R, et al. Therapeutic cancer vaccines. J Clin Invest 2015;125:3401-12. [Crossref] [PubMed]
- Lee MY, Jeon JW, Sievers C, et al. Antigen processing and presentation in cancer immunotherapy. J Immunother Cancer 2020;8:e001111. [Crossref] [PubMed]
- Grau M, Walker PR, Derouazi M. Mechanistic insights into the efficacy of cell penetrating peptide-based cancer vaccines. Cell Mol Life Sci 2018;75:2887-96. [Crossref] [PubMed]
- Kenter GG, Welters MJ, Valentijn AR, et al. Vaccination against HPV-16 oncoproteins for vulvar intraepithelial neoplasia. N Engl J Med 2009;361:1838-47. [Crossref] [PubMed]
- Muderspach L, Wilczynski S, Roman L, et al. A phase I trial of a human papillomavirus (HPV) peptide vaccine for women with high-grade cervical and vulvar intraepithelial neoplasia who are HPV 16 positive. Clin Cancer Res 2000;6:3406-16. [PubMed]
- Li W, Joshi MD, Singhania S, et al. Peptide Vaccine: Progress and Challenges. Vaccines (Basel) 2014;2:515-36. [Crossref] [PubMed]
- Lee SE, Kim SY, Jeong BC, et al. A bacterial flagellin, Vibrio vulnificus FlaB, has a strong mucosal adjuvant activity to induce protective immunity. Infect Immun 2006;74:694-702. [Crossref] [PubMed]
- Rhee JH, Lee SE, Kim SY. Mucosal vaccine adjuvants update. Clin Exp Vaccine Res 2012;1:50-63. [Crossref] [PubMed]
- Nguyen CT, Hong SH, Sin JI, et al. Flagellin enhances tumor-specific CD8(+) T cell immune responses through TLR5 stimulation in a therapeutic cancer vaccine model. Vaccine 2013;31:3879-87. [Crossref] [PubMed]
- Lee SE, Hong SH, Verma V, et al. Flagellin is a strong vaginal adjuvant of a therapeutic vaccine for genital cancer. Oncoimmunology 2015;5:e1081328. [Crossref] [PubMed]
- Zheng JH, Nguyen VH, Jiang SN, et al. Two-step enhanced cancer immunotherapy with engineered Salmonella typhimurium secreting heterologous flagellin. Sci Transl Med 2017;9:eaak9537. [Crossref] [PubMed]
- Yang MC, Yang A, Qiu J, et al. Buccal injection of synthetic HPV long peptide vaccine induces local and systemic antigen-specific CD8+ T-cell immune responses and antitumor effects without adjuvant. Cell Biosci 2016;6:17. [Crossref] [PubMed]
- Zwaveling S, Ferreira Mota SC, Nouta J, et al. Established human papillomavirus type 16-expressing tumors are effectively eradicated following vaccination with long peptides. J Immunol 2002;169:350-8. [Crossref] [PubMed]
- Songock WK, Kim SM, Bodily JM. The human papillomavirus E7 oncoprotein as a regulator of transcription. Virus Res 2017;231:56-75. [Crossref] [PubMed]
- Knapp AA, McManus PM, Bockstall K, et al. Identification of the nuclear localization and export signals of high risk HPV16 E7 oncoprotein. Virology 2009;383:60-8. [Crossref] [PubMed]
- Chamoto K, Al-Habsi M, Honjo T. Role of PD-1 in Immunity and Diseases. Curr Top Microbiol Immunol 2017;410:75-97. [Crossref] [PubMed]
- He X, Zhou S, Quinn B, et al. HPV-Associated Tumor Eradication by Vaccination with Synthetic Short Peptides and Particle-Forming Liposomes. Small 2021;17:e2007165. [Crossref] [PubMed]
- Upadhaya S, Neftelino ST, Hodge JP, et al. Combinations take centre stage in PD1/PDL1 inhibitor clinical trials. Nat Rev Drug Discov 2021;20:168-9. [Crossref] [PubMed]
- Saraiya M, Unger ER, Thompson TD, et al. US assessment of HPV types in cancers: implications for current and 9-valent HPV vaccines. J Natl Cancer Inst 2015;107:djv086. [Crossref] [PubMed]
- Roman A, Munger K. The papillomavirus E7 proteins. Virology 2013;445:138-68. [Crossref] [PubMed]
- Massarelli E, William W, Johnson F, et al. Combining Immune Checkpoint Blockade and Tumor-Specific Vaccine for Patients With Incurable Human Papillomavirus 16-Related Cancer: A Phase 2 Clinical Trial. JAMA Oncol 2019;5:67-73. [Crossref] [PubMed]
- Smalley Rumfield C, Pellom ST, Morillon Ii YM, et al. Immunomodulation to enhance the efficacy of an HPV therapeutic vaccine. J Immunother Cancer 2020;8:e000612. [Crossref] [PubMed]