Characteristics and significance of T lymphocyte subsets in peripheral blood of osteosarcoma mice
Introduction
Osteosarcoma (OS) is the most common malignant bone tumor. It usually occurs in childhood and young age, and often has lung metastasis. The survival rate after tumor metastasis is only 20%. Although the treatment of OS has progressed from single surgical resection to the combination of surgery, radiotherapy and chemotherapy, the survival rate of OS patients has not been significantly improved. Therefore, it is urgent to find new therapeutic targets and means to carry out therapeutic intervention in the early development of OS, which is of great significance to diagnose and improve the prognosis (1-3).
The formation and development of tumor are closely related to cellular immune function. Some studies have found that OS progression is attributed to immune escape mediated by immune checkpoints. Treatments including the antitumor immunocompetence of innate immune cells, which includes NK cells and γδ T cells, are found favorable for OS tumor (4). However, as a major means of early tumor detection, there are still few studies on T cells in peripheral blood of OS patients. Moreover, the progress of immunotherapy for OS mostly focused on PD1/PDL1 pathway. Therefore, a detailed study of the changes on T lymphocyte subsets in peripheral blood of OS mice is helpful to find new immunotherapy targets and provide new ideas for the treatment of OS.
In the current study, the OS mouse model was established, and the T lymphocyte subsets in peripheral blood of OS mice were detected by flow cytometry. It was found that the number of CD3+ T cells in peripheral blood of OS mice showed higher level than sham operation group, and the main source of the increase may be γδ T cells, not αβ T cells. The high level of CD4+ Treg cells may lead to the production of peripheral immunosuppressive microenvironment, resulting in the lower number of total αβ T cells, and these changes were related to the load of tumor. So, T lymphocyte subsets are potential therapeutic targets worthy of attention. We present the protocol in accordance with the ARRIVE reporting checklist (available at https://tcr.amegroups.com/article/view/10.21037/tcr-22-264/rc).
Methods
Animals
Male C3H/HeN mice (n=24, weight 20–25 g; 5 weeks old). Experiments were performed under a project license (No. 2020AE01025) granted by institutional ethics board of Nanjing Drum Tower Hospital, The Affiliated Hospital of Nanjing University Medical School, in compliance with its guidelines for the care and use of animals. Mice were kept in a SPF level environment with constant temperature (22±2 ℃), constant humidity (55%±5%) and light dark cycle for 12:12 hours and could freely obtain water and food. The mice were used the “rand” function in excel to randomly divided into two groups: sham operation group (Sham) and tumor group (Tumor).
Establishment of mouse OS model
NCTC 2472 sarcoma cells (ATCC, USA) were cultured in NCTC 135 medium containing 10% horse serum (sigma, USA) (GIBCO, USA), 37 ℃, 5% CO2 incubator (thermo forma, USA).
Mice were anesthetized by intraperitoneal injection of 2% Pentobarbital Sodium (50 mg/kg). The right knee joint of mice was cut, and then 20 µL α-MEM (Thermo Fisher Scientific, USA) containing 2×105 NCTC 2472 cells or cell-free α-MEM was injected into the medullary cavity of the right distal femur. Seal the injection hole with bone wax and suture the wound.
Flow cytometry
The eyeball blood of mice was collected with EDTA anticoagulant tube on the 14th and 21st day after operation. Red blood cells were lysed by lysing buffer (BD Pharmingen, CA, USA) according to the manufacturer’s protocol. For cell surface staining, aliquots of single cell suspensions (1×106) were incubated with fluorophore-conjugated monoclonal antibodies at room temperature in the dark (Alexa Fluor 488-anti-CD3, PE-cy7-anti-CD4 or EF450-anti-CD4, PE-cy5.5-anti-CD8, BV421-anti-TCRγδ and/or APC-anti-CD25; all from Biolegend, San Jose, CA, USA). For intracellular staining, cells were stimulated by incubation for 4 h in RPMI 1640 medium, phorbol 12-myristate 13-acetate (50 ng/mL), ionomycin (1 µg/mL), and brefeldin A (1 µg/mL) (all from Biogems, Rocky Hill, NJ, USA) in a 5% CO2 atmosphere at 37 ℃. The cells were then washed with PBS, fixed, permeabilized, and stained with PE-cy7-anti-IL-17A (Biolegend, San Jose, CA, USA) according to the manufacturer’s protocol. For intranuclear transcription factor detect, cells were washed, fixed, permeabilized, and stained with PE-anti-Foxp3 (BD Biosciences, San Jose, CA, USA) according to the manufacturer’s protocol. Fluorescence data were collected on a FACS Aria Ⅲ (BD Biosciences, San Jose, CA, USA) and analyzed with FlowJo software (Tree Star, Ashland, OR, US).
Statistical analysis
Data were analyzed with SPSS (Statistical Package for the Social Science) 20.0 software (IBM Corp., Armonk, NY, USA) and are expressed as the mean ± standard error (SE). Differences between two groups were evaluated using t-test or the non-parametric Mann-Whitney U-test. P values less than 0.05 were considered statistically significant.
Results
The proportion of T lymphocytes in peripheral blood of OS mice increased
In order to investigate the changes of T lymphocytes in peripheral blood of OS mice, the expression percentage of CD3 in peripheral blood cells was detected by flow cytometry. As shown in Figure 1, the proportion of CD3+ T cells in peripheral blood of OS mice did not change significantly compared with sham group on the 14th day after modeling but increased significantly on the 21st day after modeling (P<0.01), which suggested that T cells are activated and proliferated in OS environment.
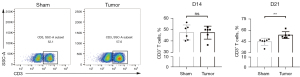
Changes of peripheral blood T lymphocytes subsets in OS mice
To further explore the effect of OS on peripheral blood T cells, we then detected the proportion of main T cell subsets. As illustrated in Figure 2A, the proportion of CD3+ γδ T cells in the tumor group was significantly higher than that in sham group no matter on the 14th or 21st day after modeling (P<0.05), suggesting that OS created an inflammatory environment and activated cellular immunity. In addition, as the main components of CD3+ αβ T cells, the level of CD4+ T cells and CD8+ T cells, did not change significantly compared with sham group on the 14th day after modeling, but were significantly lower than sham group on the 21st day after modeling (P<0.01, P<0.01, respectively, Figure 2B,2C) indicating that there is a certain peripheral immunosuppression.
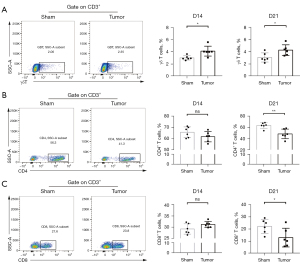
CD4+ Tregs and CD4+ Treg/Th17 ratio in peripheral blood of OS mice increased
Due to the generation of immunosuppression, we further analyzed the changes of CD4+ T cell subsets including Tregs, T helper cells 17 (Th17) cells and their ratio. Statistics showed that on the 14th day after modeling, the percentage of CD4+ Tregs in peripheral blood of tumor group mice increased significantly (P<0.05, Figure 3A,3B), while Th17 cells and Treg/Th17 ratio had no significant change. As shown in Figure 3C, on the 21st day after modeling, the proportion of CD4+ Tregs and Treg/Th17 ratio in peripheral blood of tumor group increased significantly (P<0.01, P<0.05, respectively) compared with sham group. There was no significant difference in Th17 cells.
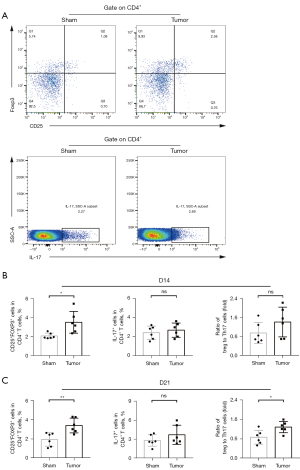
The changes of T lymphocytes in peripheral blood of OS mice were correlated with tumor progression
As illustrated in Figure 4, comparing the T lymphocytes subsets on the 14th and 21st day after modeling in the tumor group, it was found that CD3+ T cells and CD4+ Tregs on the 21st day after modeling were significantly higher than those on the 14th day (P<0.05, P<0.001, respectively), while CD4+ T cells, CD8+ T cells decreased significantly (P<0.05, P<0.05, respectively). These results suggested that the changes of T lymphocytes in peripheral blood of OS mice are related to tumor development.
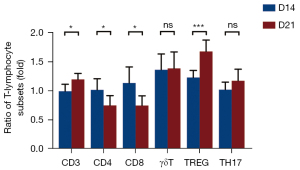
Discussion
T cells are important lymphocytes in tumor immunity. They can not only play an immune surveillance role in the human body, but also correct the tumor immune hypofunction. They could directly kill tumor cells and play a leading role in the process of tumor immunity. However, they would also differentiate into immunosuppressive cells to promote tumor development (5,6). Therefore, the dynamic balance of T cell clustering is particularly important in the fight between the body and tumor. T cells can be basically divided into αβ T cells and γδ T cells. αβ T cells are mainly constituted of CD4+ T cells and CD8+ T cells (7). Various studies have shown that CD4+ T cells are closely related to the occurrence and development of tumors (8). The known major CD4+ T cell subsets include Th1 cells, Th2 cells, Th17 and Tregs. These cell subsets maintain the stability of immune balance through secreting cytokines (9). Once unbalanced, it would lead to inflammation, autoimmune diseases and tumors (10). Tregs are a group of CD4+ T cell subsets with specific immunosuppressive effects. They play an anti-tumor immune role in breast cancer, colorectal cancer, non-small cell lung cancer and hepatocellular carcinoma, assisting tumor growth and metastasis (11-14). Th17 cells are also differentiated from CD4+ T cells. In contrast to Tregs-mediated immune tolerance, Th17 mainly secretes pro-inflammatory factors and mediates immune rejection. The imbalance of Treg/Th17 ratio reflects the disorder of the immune system (15). CD8+ T cells are one of the important anti-tumor cells. They kill tumor cells specifically through perforin granzyme, Fas-FasL and TNF-TNFR (16). γδ T cells are a kind of non-MHC restricted innate T lymphocytes that are widely distributed in peripheral blood and mucosal tissues. They link up natural and adaptive immunity and are the first line of defense. Recent studies have shown that γδ T cells participate in tumor immunity, which can not only play a cytotoxic effect to kill tumor cells, but also mediate the immune escape of tumor cells (17).
In the studies of OS, it was found that the number of CD4+ and CD8+ T cells in peripheral blood of dogs with OS was significantly lower than that of healthy dogs, meanwhile Tregs was significantly higher than that of healthy dogs (18). CD3+ T cells in peripheral blood of patients with human OS have higher expression of exhaustion markers than those of healthy controls (19). In this study, we found that CD4+ T cells and CD8+ T cells in peripheral blood of OS mice were significantly lower than those in sham group, and the level of Tregs was significantly higher than sham group, which was consistent with the study of dogs. Besides, the number of γδT cells and Treg/Th17 ratio were also significantly higher than those in sham group. These results jointly proved that OS is an immune cold tumor, which can improve immune cell activity and survival rate through treatment.
Although the mouse OS model is very similar to the human OS environment, it still cannot be completely simulated. The next important step is to collect fresh patient samples to further study the relationship between molecular events and various clinicopathological features. Furthermore, there are some studies that use mathematical models to investigate OS, including bone modeling, osteoblast cells, or OS treatments (20,21). Le et al. (22) build a data-driven model for the progression of OS tumors that considers immune cell interactions with tumor cells. And our current study can provide related data for computational oncologists to improve their models. These studies may find new predictors of chemotherapy response and survival, as well as other therapeutic targets.
In conclusion, the data of this study showed that in the peripheral blood of OS model mice, the proportions of T lymphocyte subsets were unbalanced. Tregs were increased and accompanied by peripheral immunosuppression, suggesting that these cells can be used as potential diagnostic indicators and therapeutic targets of OS.
Acknowledgments
Funding: This study was supported by grants from the National Natural Science Foundation of China (No. 81971044).
Footnote
Reporting Checklist: The authors have completed the ARRIVE reporting checklist. Available at https://tcr.amegroups.com/article/view/10.21037/tcr-22-264/rc
Data Sharing Statement: Available at https://tcr.amegroups.com/article/view/10.21037/tcr-22-264/dss
Conflicts of Interest: All authors have completed the ICMJE uniform disclosure form (available at https://tcr.amegroups.com/article/view/10.21037/tcr-22-264/coif). The authors have no conflicts of interest to declare.
Ethical Statement: The authors are accountable for all aspects of the work in ensuring that questions related to the accuracy or integrity of any part of the work are appropriately investigated and resolved. Experiments were performed under a project license (No. 2020AE01025) granted by institutional ethics board of Nanjing Drum Tower Hospital, The Affiliated Hospital of Nanjing University Medical School, in compliance with its guidelines for the care and use of animals.
Open Access Statement: This is an Open Access article distributed in accordance with the Creative Commons Attribution-NonCommercial-NoDerivs 4.0 International License (CC BY-NC-ND 4.0), which permits the non-commercial replication and distribution of the article with the strict proviso that no changes or edits are made and the original work is properly cited (including links to both the formal publication through the relevant DOI and the license). See: https://creativecommons.org/licenses/by-nc-nd/4.0/.
References
- Gill J, Gorlick R. Advancing therapy for osteosarcoma. Nat Rev Clin Oncol 2021;18:609-24. [Crossref] [PubMed]
- Isakoff MS, Bielack SS, Meltzer P, et al. Osteosarcoma: Current Treatment and a Collaborative Pathway to Success. J Clin Oncol 2015;33:3029-35. [Crossref] [PubMed]
- Meltzer PS, Helman LJ. New Horizons in the Treatment of Osteosarcoma. N Engl J Med 2021;385:2066-76. [Crossref] [PubMed]
- Chen C, Xie L, Ren T, et al. Immunotherapy for osteosarcoma: Fundamental mechanism, rationale, and recent breakthroughs. Cancer Lett 2021;500:1-10. [Crossref] [PubMed]
- Meng X, Wu X, Zheng Y, et al. Exploiting Ca2+ signaling in T cells to advance cancer immunotherapy. Semin Immunol 2020;49:101434. [Crossref] [PubMed]
- Lei K, Kurum A, Tang L. Mechanical Immunoengineering of T cells for Therapeutic Applications. Acc Chem Res 2020;53:2777-90. [Crossref] [PubMed]
- Morath A, Schamel WW. αβ and γδ T cell receptors: Similar but different. J Leukoc Biol 2020;107:1045-55. [Crossref] [PubMed]
- Chen YQ, Li PC, Pan N, et al. Tumor-released autophagosomes induces CD4+ T cell-mediated immunosuppression via a TLR2-IL-6 cascade. J Immunother Cancer 2019;7:178. [Crossref] [PubMed]
- Yin X, Chen S, Eisenbarth SC. Dendritic Cell Regulation of T Helper Cells. Annu Rev Immunol 2021;39:759-90. [Crossref] [PubMed]
- Le Menn G, Jabłońska A, Chen Z. The effects of post-translational modifications on Th17/Treg cell differentiation. Biochim Biophys Acta Mol Cell Res 2022;1869:119223. [Crossref] [PubMed]
- Duan MC, Han W, Jin PW, et al. Disturbed Th17/Treg Balance in Patients with Non-small Cell Lung Cancer. Inflammation 2015;38:2156-65. [Crossref] [PubMed]
- Adamczyk A, Pastille E, Kehrmann J, et al. GPR15 Facilitates Recruitment of Regulatory T Cells to Promote Colorectal Cancer. Cancer Res 2021;81:2970-82. [Crossref] [PubMed]
- Hughes E, Lauder SN, Smart K, et al. Primary breast tumours but not lung metastases induce protective anti-tumour immune responses after Treg-depletion. Cancer Immunol Immunother 2020;69:2063-73. [Crossref] [PubMed]
- Liu HR, Li WM. Treg-specific demethylated region activity in isolated regulatory t lymphocytes is a surrogate for disease severity in hepatocellular carcinoma. IUBMB Life 2015;67:355-60. [Crossref] [PubMed]
- Barbi J, Pardoll D, Pan F. Metabolic control of the Treg/Th17 axis. Immunol Rev 2013;252:52-77. [Crossref] [PubMed]
- Sanchez-Ruiz M, Iorgu AM, Küster F, et al. CD8 T cell-Derived Perforin and TNF-α Are Crucial Mediators of Neuronal Destruction in Experimental Autoimmune Enteric Ganglionitis. Am J Pathol 2021;191:1064-76. [Crossref] [PubMed]
- Chabab G, Barjon C, Bonnefoy N, et al. Pro-tumor γδ T Cells in Human Cancer: Polarization, Mechanisms of Action, and Implications for Therapy. Front Immunol 2020;11:2186. [Crossref] [PubMed]
- Biller BJ, Guth A, Burton JH, et al. Decreased ratio of CD8+ T cells to regulatory T cells associated with decreased survival in dogs with osteosarcoma. J Vet Intern Med 2010;24:1118-23. [Crossref] [PubMed]
- Sun CY, Zhang Z, Tao L, et al. T cell exhaustion drives osteosarcoma pathogenesis. Ann Transl Med 2021;9:1447. [Crossref] [PubMed]
- Burova I, Peticone C, De Silva Thompson D, et al. A parameterised mathematical model to elucidate osteoblast cell growth in a phosphate-glass microcarrier culture. J Tissue Eng 2019;10:2041731419830264. [Crossref] [PubMed]
- Fernández-Cervantes I, Morales MA, Agustín-Serrano R, et al. Polylactic acid/sodium alginate/hydroxyapatite composite scaffolds with trabecular tissue morphology designed by a bone remodeling model using 3D printing. Journal of Materials Science 2019;54:9478-96. [Crossref]
- Le T, Su S, Kirshtein A, et al. Data-Driven Mathematical Model of Osteosarcoma. Cancers (Basel) 2021;13:2367. [Crossref] [PubMed]